Animal Models for Age-Related Macular Degeneration
Matías E. Czerwonko
Mariana Ingolotti
Amelia Nelson
Jamel L. F. Brown
D. Virgil Alfaro III
INTRODUCTION
Age-related macular degeneration (AMD) is a late-onset, multifactorial, neurodegenerative disease of the retina and the leading cause of irreversible vision loss in the elderly in the Western world (1). It affects 30 to 50 million individuals, and clinical hallmarks of AMD are observed in at least one-third of persons over the age of 75 in industrialized countries (2). Animal models, in particular, have contributed greatly to current knowledge regarding the etiology, molecular mechanisms, and histologic features of this extremely prevalent condition.
As we consider animal models of AMD, with the potential for preclinical investigation, various pathologic and nonpathologic histologic elements must be considered. In the particular case of AMD, we can roughly divide its typical features into two types: histologic and functional alterations.
Among the histologic features that have been reported from the eyes of patients with AMD are the following:
▪ Thickening of the Bruch’s membrane (BM)
▪ Subretinal pigmented epithelium (RPE) basal laminar deposits and basal linear deposits (i.e., drusen)
▪ Hyperplasia
▪ Changes in the RPE including loss of the basal infoldings
▪ Accumulation of immune cells (i.e., macrophages and microglia)
▪ Atrophy
▪ Photoreceptor atrophy
▪ Deposition of activated complement proteins
▪ Retinal or choroidal neovascularization (CNV)
▪ Fibrosis
▪ Accumulation of lipofuscin in RPE cells with increased levels of A2E and corresponding increases in autofluorescence
Functional changes refer to decreased signals on electro-retinograms (ERGs) secondary to photoreceptor atrophy (3,4,5,6,7). Although AMD disease mechanisms are still poorly understood, several pathogenic pathways have been proposed, each associated with manifestations of AMD, including oxidative damage, metabolic deregulation, and chronic inflammatory processes. These pathways have been tested and confirmed in studies based on animal models, most of which are explained in this chapter.
Mice have been widely used for generating models that simulate human AMD features for investigating the pathogenesis, treatment, and prevention of the disease. Focal atrophy of photoreceptors and RPE, lipofuscin accumulation, and increased A2E can be present in aged mouse eyes, even though the mouse does not have a macula.
Unfortunately, drusen are rarely seen in mice because of their simpler BM and different process of lipofuscin extrusion compared with humans. Analyzing basal deposits at the ultrastructural level and understanding the ultra-structural pathologic differences between various mouse AMD models are therefore critical to comprehend the significance of research findings. More recently, some other animal models have entered the world of AMD research. While nonhuman primate models have gained popularity over the past few years because of their resemblance to the ocular anatomic features of humans, rabbits and pigs are less often used.
Unfortunately, drusen are rarely seen in mice because of their simpler BM and different process of lipofuscin extrusion compared with humans. Analyzing basal deposits at the ultrastructural level and understanding the ultra-structural pathologic differences between various mouse AMD models are therefore critical to comprehend the significance of research findings. More recently, some other animal models have entered the world of AMD research. While nonhuman primate models have gained popularity over the past few years because of their resemblance to the ocular anatomic features of humans, rabbits and pigs are less often used.
The purpose of this chapter is to provide a framework for better understanding some of the existing animal models and the knowledge that has been derived from their study regarding both dry and wet types of AMD. Some of these models have encouraged the development of some promising lines of investigation concerning novel therapeutic approaches. Nevertheless, it is important to understand that most of these models are not necessarily etiologic representations of human disease.
ETHICS OF ANIMAL USE
The use of animals in biomedical research dates back to the fifth century BCE. Our ancient ancestors realized the homogenous relationship between humans and other animals and the subsequent medical discoveries that come from experimentation. Since then, nonhuman animals have proved themselves to be appropriate models for scientific inquiries, contributing to medical science. However, with the use of animals in research must come a regard for the ethical treatment and general welfare of the animals used.
The first mention of animal usage in the medical field occurred around 500 BCE. Pythagoras, an ancient philosopher and mathematician, recognized a common soul between animals and humans—a connection that could be used to make certain comparisons. Hippocrates was the first to directly compare specific organs. Vivisection was even practiced among certain scientists, including Alcmaeon, Herophilus, and Erasistratus, at this time in history. Years later, the use of vivisection was exercised even more so by one of the fathers of medical experimentation, Galenus. The first true published experiment involving animals did not occur until 1638 CE by English physician William Harvey. However, the issue of ethics in these experiments was not mentioned until the 18th century. British philosopher Jeremy Bentham published his ideas on the welfare of animals, which led to the start of regulations and protection of animals. Bentham is responsible for projecting the idea of utilitarianism, the ethical doctrine stating if an action provides benefit to society, it is indeed morally right. He was the first to question the actual suffering of animals in experiments, and laws regarding such would be the result.
Britain was the first country to pass laws concerning animal welfare. The first association to establish themselves as protectors of animals in medical research was the Society for the Prevention of Cruelty to Animals, begun in the year 1824 in England. The first law to pass was the British Cruelty to Animals Act in 1876. The issue of animal rights did not die in England during the 1900s; in 1969, FRAME (Fund for the Replacement of Animals in Medical Experiments) was established.
In 1909, Americans started publishing on behalf of animal rights. The first federal law passed in America regarding animal cruelty was created in 1966 and called the U.S. Animal Welfare Act, serving to protect the welfare of laboratory animals. Animal rights organizations such as PETA (People for the Ethical Treatment of Animals) and the Johns Hopkins Center for Alternatives to Animal Testing began forming in the 1980s. The three R’s, replace, reduce, and refine, became a sentiment for animal activists across the globe. Laws and regulations on the ethics of animals in the laboratory are still fighting to be passed, and the conflict lingers with a conclusion still remaining to be determined.
The first topic to be addressed when discussing animal research is the obvious question: What is the purpose? Or, in other words, is there indeed a need for animal experimentation? Some claim that animals and humans are simply too different to make valid comparisons and that ultimately society does not benefit from animal research. Of course, this is true if one was to compare completely dissimilar organisms, such as two animals in entirely different animal kingdoms. Nonetheless, properly executed animal experiments have led to many historical medical breakthroughs, including the discovery and refinement of insulin in dogs.
Another concern when evaluating the ethics of using animals in the laboratory is the actual success rate of the research itself. How often does an experiment involving animals actually lead to something valuable and how often does it simply fail to achieve useful results? To answer this question, one must determine what constitutes a low success rate, a term whose meaning is relative to the person who is using it. Since a “low success rate” is not attached to a tangible number, there is no way of knowing if animal experimentation would qualify as such. The rebuttal to this argument points out that trial and error is one of the characteristics of scientific research and explains that upon comprehension of the scientific method should come a realization of the inevitable chance of failure.
While there is still disagreement among people on opposing sides of the issue, there is common ground being reached. Scientists have resorted to alternative methods of experimentation. In many instances, in vitro techniques can replace animal testing. Scientists take stem cells from humans to mimic real human responses to testing. They can also use brain imaging, such as MRI or CT scans, to study human brains instead of animal brains and even imitate real human responses through computer simulation. Although the two different sides of the issue conflict, alternative methods to animal testing serve as a satisfying compromise.
Another point to be addressed is the inclusion of policies on ethical standards in lab experiments and reports. One study shows that 40.7% of published work involving animal testing was performed with a respect for ethical standards. A different study, using articles from PubMed, shows that 53% of the research had ethical policies. Both of these studies show that there is much improvement to be done in this field. Inclusion of ethical standards in animal research should hopefully reach 100%, proving that all research involving animals displays ethical treatment to the laboratory animals. Not only does reporting ethical standards in studies show that the science has taken a moral standpoint, but it also implies scientific integrity and respect in the medical field (8,9,10,11,12).
ANIMAL MODELS OF DRY MACULAR DEGENERATION
Rodent Models for Dry AMD
The active role of oxidative stress, inflammation, and metabolic deregulation in AMD pathogenesis has been well established (13). Pathologic hallmarks of the dry type of AMD include drusen accumulation, BM thickening, RPE, and photoreception degeneration followed by atrophy. Mice have been the most commonly used models for retinal disease because their retina resembles the human nasal and peripheral retina. The described murine models for dry AMD have drusen-like lesions and RPE atrophy on funduscopy, ERG abnormalities, lipofuscin accumulation, sub-RPE basal deposits, and BM thickening. Undoubtedly, one of the most seductive advantages of murine models is the ease to manipulate them genetically, surgically, and pharmacologically.
The aforementioned dry AMD murine models can be conveniently classified into three categories:
▪ Genetically engineered mice
▪ Immunologically manipulated mice
▪ Naturally occurring mouse strains
Most murine models are genetically engineered to manipulate genes that are suspected to cause macular degeneration-like disorders in humans or might be somehow associated to AMD pathogenesis. Inflammatory genes (Cfh, Ccl2, Ccr2, and Cx3cr1), oxidative stress-associated genes (Sod1 and Sod2), and metabolic pathway genes (mcd, Cp, Heph, ApoE, and ApoB100) have been manipulated to create models with features that are typical of dry AMD. The immunologically manipulated mouse model is immunized against carboxyethylpyrrole (CEP), an endogenous biomarker of oxidative stress. Finally, the naturally occurring mouse models include retinal degeneration models with mice exposed to nocive and AMD-related conditions and the senescence-accelerated mouse (SAM) models with systemic and retinal age-related pathology, including BM thickening, basal deposits, and CNV.
In the following paragraphs, we explain the most important models of each of the groups mentioned above. Overall, these models contrast with the murine models of wet AMD that are mostly induced mechanically and are explained later in this chapter.
LIPID AND GLUCOSE METABOLISM MODELS
A growing body of the literature indicates the involvement of lipids, lipoproteins, and glucose in the formation of extracellular lesions in aging BM, basal deposits and drusen, and other AMD hallmarks. Observational studies have indicated that maintaining adequate levels of fatty acids or a low glycemic index (GI) diet may be particularly beneficial for early stages of the disease. From a pathophysiologic perspective, lipids and cholesterol that concentrate within the BM with aging are believed to restrict the metabolite transference between the RPE and the choriocapillaris (14,15,16,17,18). From a structural point of view, lipids and cholesterol deposits may promote the formation of both basal laminar and basal linear deposits. We could fairly claim that there are three major metabolism-related risk factors that have been associated to AMD pathogenesis:
▪ Diet: High intakes of cholesterol and monounsaturated, polyunsaturated, and saturated fats have been associated with the onset and progression of AMD (19).
▪ Cardiovascular diseases: An apparent correlation between the deposition of cholesterol and lipids in atherosclerotic plaques and the material accumulated in the BM was described. Nevertheless, numerous studies have shown opposing results so that controversy still exists as to how tight this association really is, if there is any (20,21,22).
The following sections are dedicated to mention and describe the most important animal models that have been designed to assess the potential connection between lipid and glucose metabolism and AMD pathogenesis.
Aging and a High-Fat Diet
To examine the histologic, histochemical, and ultrastructural changes in the BM in C57BL/6 mice on a high-fat (HF) diet, Dithmar et al. compared 2-month-old models on a normal diet and 8-month-old mice on a normal or HF diet. Confirming the importance of aging, thickening of the BM was found in 8-month-old mice on both normal and HF dietary regimens. However, 8-month-old mice exposed to HF diets showed greater thickness compared to that of nonexposed counterparts. Since the plasma cholesterol level was significantly higher in mice on HF diets than the controls, the results were consistent with the suspected role of lipids in the pathogenesis of AMD. A year later to
Dithmar’s studies, Cousins et al. (25) compared 2-month-old and 16-month-old C57BL/6 mice fed normal and HF diets. In this case, basal laminar deposits were observed in the 16-month group exclusively.
Dithmar’s studies, Cousins et al. (25) compared 2-month-old and 16-month-old C57BL/6 mice fed normal and HF diets. In this case, basal laminar deposits were observed in the 16-month group exclusively.
Aging and High Glycemic Index Diet
The GI indicates how fast blood glucose is raised after consuming a carbohydrate-containing food. Human metabolic studies indicate that GI is related to abnormal responses after meals. Recent epidemiologic evidence indicates positive association between GI and risk for AMD in people without diabetes. Therefore, it is reasonable to suspect low GI diets as protective factors for the development of AMD (26,27,28,29,30).
Based on these assumptions, Uchiki et al. (31) compared mice that consumed low and high GI diets, showing that the latter promote the apparition of AMD-like lesions. Mice that consumed the lower GI diet displayed a significantly reduced frequency and severity of age-related retinal lesions such as basal deposits. Glycation-altered proteolysis is accounted for linking dietary GI, aging, and age-related diseases like AMD. Not surprisingly, consuming higher GI diets was associated with over threefold higher accumulation of advanced glycation end products (AGEs) in the retina in the age-matched mice. Both incidence and severity of retinal changes aggravated with age, as older mice exhibited more thickening of the BM, more basal laminar deposits, disorganization of RPE basal infolding, and increased photoreceptor atrophy compared to 17-month-old counterparts (32).
Apolipoproteins
Cholesterol and its transporter, apolipoprotein E (ApoE), are major constituents of sub-RPE deposits in AMD eyes. Based on these findings, the following murine models were designed and studied:
▪ ApoE knockout mice: ApoE is a constituent of very-low-density lipoprotein (VLDL) synthesized by the liver and of a subclass of high-density lipoprotein (HDL) involved in cholesterol transporting among cells. ApoE mediates high-affinity binding of ApoE-containing lipoprotein particles to the low-density lipoprotein (LDL) receptor and is thus responsible for the cellular uptake of these particles. Mice with inactivated endogenous ApoE gene exhibited very high levels of circulating cholesterol (33,34) and revealed thickening of the BM combined with electrolucent particles and membrane-bounded material at 8 months of age (35).
▪ ApoEe2/ApoEe4 transgenic mice: With the purpose of studying the multiple ApoE alleles seen in humans, transgenic mice were created replacing native ApoE with human ApoE equivalents (36,37,38). Eyes of aged mice expressing human ApoE2, ApoE3, or ApoE4 and maintained on a high fat and cholesterol (HF-C) diet showed ApoE isoform-dependent pathologies of diverse severity. They develop a constellation of changes that mimic the pathology associated with human AMD such as
▪ Diffuse subretinal pigment epithelial deposits
▪ Drusenoid deposits
▪ Thickened BM
▪ Atrophy, hypopigmentation, and hyperpigmentation of the RPE
Interestingly, ApoE4 mice were the most severely affected, to the point of having sometimes developed CNV. Transgenic ApoE4 mice fed HF-C diets have also shown to accumulate amyloid b (Ab), a well-known constituent of human drusen (39). What is more, systemic anti-Aβ immunotherapy was shown to protect against loss of visual function and retinal damage, suggesting a role for Ab in the AMD pathogenesis and functional manifestations (40). Ironically, while the ApoEe4 allele is correlated with relative protection for AMD in humans, in the mice, the opposite appears to be true (23,41,42,43). The reason for this discrepancy between species still remains unclear.
▪ ApoE3–Leiden transgenic mice: ApoE3-Leiden is a dysfunctional form of ApoE3 that is associated with a dominantly inherited form of familial dysbetalipoproteinemia and early onset of atherosclerosis (44). With the intention of investigating the ApoE3-Leiden mouse as an animal model for retinal extracellular deposits, transgenic mice carrying the ApoE3-Leiden gene were created. Eyes were obtained from ApoE3-Leiden transgenic mice on a HF-C diet or on a normal mouse chow diet for 9 months. As controls, eyes were collected from ApoE knockout mice on the same dietary regimens. All eyes of the ApoE3-Leiden mice on a HF-C diet exhibited basal laminar deposit, whereas ApoE3-Leiden mice on normal chow displayed basal laminar deposits only occasionally. In both cases, however, the ultrastructural features of these deposits were comparable with those seen in human eyes, and they all showed immunoreaction with antihuman ApoE antibodies. Importantly, no deposits were found in any of the control mice. These results indicate that ApoE3-Leiden mice can be used as an animal model for the pathogenesis of basal laminar deposits and that a HF-C diet enhances the accumulation process. Furthermore, this study supports the previously suggested involvement of dysfunctional ApoE in the accumulation of extracellular deposits in the human disease (45,46).
▪ ApoB100 transgenic mice: ApoB100 is the major apolipoprotein in LDL cholesterol. Lipoprotein particles in the BM have been shown to contain ApoB100. To study how ApoB100 might contribute to the formation of lipoproteinaceous deposits in AMD, several groups have examined mice that express human ApoB100. Young ApoB100 mice developed basal laminar deposits when fed with a HF diet and exposed to oxidative blue-green light (47). At older ages, ApoB100 mice exposed to normal diets
exhibited BM thickening, loss of the basal infoldings of the RPE, and basal laminar deposits (48,49). When these animals were additionally fed HF diets, more basal linear deposits were observed.
Lipoprotein Receptors
LDL receptor (LDLR) knockout mice are incapable of importing cholesterol, leading to increased plasma cholesterol levels and development of atheromatosis. Since atherosclerosis is a well-established risk factor for developing AMD, a murine knockout model with LDLR deficiency was used to evaluate changes in the BM. As it was expected, mice lacking LDLR exhibited a degeneration of the BM with accumulation of lipid particles, which was further increased after fat intake due to elevated blood lipid levels.
Alternatively, alterations in the gene that codes for VLDL receptor (VLDLR) have also shown some impact on the onset and progression of retinopathies. Mice with both alleles mutated do not show signs of dyslipidemia but still develop retinal neovascularization as early as 2 weeks of age (50,51,52). These vessels grow toward the subretinal space, and can form choroidal anastomosis or cause retinal hemorrhages between 30 and 60 days. Deeper analysis of this model revealed the development of CNV, elevated levels of vascular endothelial growth factor (VEGF), and dysregulation of the Wnt pathway all of which suggested VLDLR as a negative regulator of CNV (53). Therefore, modulation of the VLDLR may have a great potential from a therapeutic perspective.
More recently, polymorphisms in CD36 have also been shown to be protective factors in AMD (54). CD36 is a scavenger receptor that binds oxidized LDL (OxLDL) and is mostly expressed in the apical and basolateral membrane of the RPE (55,56,57). Mice deficient for CD36 accumulate subretinal OxLDL even when fed a regular diet (58). Even though lipids in the RPE come mostly from photoreceptor outer segments and are exocytosed through the base of the RPE to be eliminated via the choroid, RPE cells can also take up oxidized lipids from their cell base, suggesting they may be involved in clearing the subretinal space from such deposits. Laminar deposits in the BM have been found to contain OxLDL among other compounds. Consistently, CD36 deficiency in mice resulted in both age-associated accumulation of oxidized lipids and BM thickening. Coherently, treatment of HF-C-fed ApoE null mice with a CD36 agonist was shown to diminish thickening of the BM notably as well as to partially lessen photoreceptor atrophy and preserve function (58).
Cathepsin D
Cathepsin D is an aspartic protease that is highly expressed in the RPE and plays a decisive role in processing photoreceptor outer segments (59,60). Cathepsin D is secreted as a proenzyme, which accumulation has been linked to RPE dysfunction (61). To study the mechanism, transgenic mice with a mutant form of cathepsin have been developed (62). Mice homozygous for the transgene demonstrated areas of RPE atrophy as early as 9 months of age (63). Between 10 and 18 months old, these mice exhibited areas of RPE hypertrophy, photoreceptor atrophy, and reduced ERGs. Remarkably, they also displayed small yellowish spots in the fundus that were similar to basal laminar and basal linear spots.
OXIDATIVE DAMAGE MODELS
Clinical and experimental evidence supports that chronic oxidative stress is a primary contributing factor to numerous retinal degenerative diseases, specially AMD (64,65,66). Oxidative damage is normally minimized by the presence of a wide range of antioxidant and efficient repair systems. Unfortunately, aging intensifies oxidative damage while decreasing antioxidant capacity, thereby perturbing the oxidative homeostasis. Postmortem eye examinations from AMD patients have shown signs in line with extensive free radical damage (67). Consistently, antioxidant-enriched diets have been shown to reduce the progression from dry to wet AMD (68).
Several mouse models of chronic oxidative stress displayed many of the most characteristic hallmarks of AMD. These models can be classified into two groups: those that lack intrinsic antioxidant mechanisms and those where additional oxidative stress is applied. However, whether oxidative stress is an etiologic component or if it is just involved in disease progression is still controversial.
Carboxyethylpyrrole-Adducted Products
Recent evidence has implicated AMD as an immunologically mediated disease (69). The protein adduct CEP is present in AMD eye tissue and in the blood of AMD patients at higher levels than found in age-matched non-AMD tissues. Autoantibodies to CEP are also higher in AMD blood samples than in controls. It has been suggested that a signal from the outer retina initiates an immune response in AMD. CEP acts as a reliable biomarker of oxidative stress and is currently suspected to be one of those signals (70,71).
To test such hypothesis, Hollyfield et al. (72,73) created a mouse model by immunizing animals with CEP-adducted mouse serum albumin. Essentially, two groups were compared: a short-term group that received a strong immunologic challenge over 3 months and a long-term group that was inoculated with a weaker challenge but over a year. Both groups successfully developed antibodies to CEP-adducted albumin. At 3 months, the short-term group showed deposition of complement component C3d in BM, RPE swelling and lysis, sub-RPE deposits, pyknosis of overlying photoreceptors, and invasion of macrophages. Longterm counterparts, on the other hand, exhibited a three- to fivefold thickening of BM. There existed a solid correlation
between the levels of CEP-specific antibodies and the severity of pathologic alterations. Importantly, although CEP is also proangiogenic via a VEGF-independent pathway (74), neither the short-term nor long-term group exhibited signs of CNV, confirming that the utility of this model is limited for dry AMD only.
between the levels of CEP-specific antibodies and the severity of pathologic alterations. Importantly, although CEP is also proangiogenic via a VEGF-independent pathway (74), neither the short-term nor long-term group exhibited signs of CNV, confirming that the utility of this model is limited for dry AMD only.
SOD Models
Superoxide dismutases (SODs) are one of the major antioxidant defense systems, which consist of three isoforms in mammals: the cytoplasmic SOD (SOD1), the mitochondrial SOD (SOD2), and the extracellular SOD (SOD3). All of these isoforms require catalytic metal (Cu or Mn) for their activation (75). To investigate the role of oxidative stress in AMD pathogenesis, transgenic mice targeting the SOD oxidative stress recovery pathways have been created and examined. With some significant variances, knockout mice for different SOD genes showed typical features of AMD.
SOD1 levels are particularly high in the retina, and its protective role against oxidative damage is unquestionable. Imamura et al. showed that SOD1 knockout mice display age-related pathologic changes in the retina reminiscent of AMD as early as 7 months of age. Among the most frequent findings were the presence of drusen, the thickening of BM, and the development of CNV (being thereby suitable for simulating wet forms of AMD as well) (76). However, prior to the 7-month period, retinas from these animals were absolutely indistinguishable from age-matched wild-type animals (76). As with many other models, lesions increased in number and severity with age: At 10 months of age, 86% of mice had drusen, compared to very few drusen in age-matched wild-type mice (76), and at a year of age, RPE vacuolization and degenerative changes were noted. Remarkably, yellowish drusen-like deposits between the RPE and BM stained for some of the most typical drusen biomarkers such as vitronectin, carboxymethyl lysine, and tissue inhibitor metalloproteinase 3 (TIMP3) (76,77). Senescent SOD1 knockout mice demonstrated evidence of necrotic death of cells in the inner nuclear layer (INL) and reduced ERGs (78).
SOD2 knockout mice have been used as models for dry AMD as well, but not without some difficulties. Contrary to SOD1, the antioxidant enzyme manganese superoxide dismutase (MnSOD), encoded by SOD2, is in the mitochondrial matrix, and polymorphisms in its encoded gene have been strongly associated with the development of AMD (79). Unfortunately, most SOD2 knockout mice died of dilated cardiomyopathy by the early age of 10 days, limiting their use as a model for maculopathies (80). Nevertheless, retinal histology from some of the few that survived over 3 weeks demonstrated significant central thinning of all retinal layers (81). Justilien et al. (82) managed to overcome the limitations of this model by transfecting mouse retina and RPE with an adenovirus that expresses a ribozyme that degrades SOD2, thereby reducing its levels only locally and diminishing major systemic consequences. Thickening of BM, degeneration of the RPE, increased autofluorescence, elevation of A2E levels, and atrophy of the photoreceptors were among the most remarkable histologic findings.
Ceruloplasmin and Hephaestin
Iron is an essential cofactor for many enzymes, but ferrous iron (Fe2 +) can cause oxidative damage via the Fenton reaction. Such oxidative stress increases in the body with aging and may contribute to AMD pathogenesis when affecting the retina (83). Indeed, compared to normal eyes, AMD eyes show a statistically significant increase in total iron in the RPE and BM (84). Since ceruloplasmin is believed to facilitate iron export from cells, it is reasonable to expect humans without functional ceruloplasmin to develop drusen and retinal pigmentary changes later in life (85,86,87). Nevertheless, mice lacking functional ceruloplasmin only showed a trivial iron overload (88) with mild retinal alterations. The most satisfactory explanation for such discrepancies lies in the presence of a second ferroxidase named hephaestin (Heph), which may compensate, at least partially, the impact caused by the ceruloplasmin loss (88,89,90). As a result, it was necessary to create mice with combined deficiencies in Cp and Heph so as to assess the potential influence of iron overload in AMD (91). By 5 months of age, these double knockout models had fruitfully increased iron levels and displayed subsequent iron-laden electrondense vesicles inside the RPE cells. Focal areas of RPE hypertrophy and hypopigmentation in the midperipheral retina, subretinal deposits, photoreceptor atrophy, and sub-retinal neovascularization start appearing at the age of 6 to 9 months, and after a year, these models showed infiltration of macrophages, focal areas of hyperautofluorescence, and complement disposition (92). It is of interest to note that the RPE hypertrophy in this model was greater than what had been observed in AMD retinas (91,92). Unfortunately, most double knockout mice die from a movement disorder at a very young age, thus hindering possibility to study long-term effects.
Exogenous Oxidative Conditions: Cigarette Smoke, Hydroquinone, High-Fat Diet, and Blue Light
It is unanimously accepted that smoking constitutes the major preventable risk factor for AMD (93,94,95,96,97). Cigarette smoke contains over 4,000 potentially damaging toxins, many of which are pro-oxidants including carbon monoxide, nitric oxide, and hydroquinone (98). The association between AMD pathogenesis and oxidative harm caused by environmental factors has been studied in multiple animal models. Espinosa-Heidmann et al. described the histologic alterations of mice exposed to combinations of HF diets, blue light, and whole cigarette smoke versus oral hydroquinone. Animal exposed to these conditions
exhibited changes to the BM and had increased basal laminar deposits (25,99). Plus, several mice exhibit invasion of the choriocapillaris into the BM suggestive of early AMD. Wild-type mice treated with hydroquinone in their drinking water develop decreased expression of Ccl-2 in the RPE and choroid and demonstrated altered ratios of proangiogenic VEGF versus antiangiogenic pigment epithelium-derived factor (PEDF) (100). Therefore, it was hypothesized that hydroquinone may raise the risk of CNV by disturbing the normal balance between angiogenic factors. Nevertheless, some studies have reached different results regarding hydroquinone activity so that it remains a matter of debate.
exhibited changes to the BM and had increased basal laminar deposits (25,99). Plus, several mice exhibit invasion of the choriocapillaris into the BM suggestive of early AMD. Wild-type mice treated with hydroquinone in their drinking water develop decreased expression of Ccl-2 in the RPE and choroid and demonstrated altered ratios of proangiogenic VEGF versus antiangiogenic pigment epithelium-derived factor (PEDF) (100). Therefore, it was hypothesized that hydroquinone may raise the risk of CNV by disturbing the normal balance between angiogenic factors. Nevertheless, some studies have reached different results regarding hydroquinone activity so that it remains a matter of debate.
OXY Rat
The term OXY rats refer to an inbred strain of Wistar rats that were chosen for susceptibility or resistance to the early development of cataracts when fed with diets rich in galactose (101). The susceptible line (OXYS) displays high levels of hydroxyl radical formation and lipid peroxidation causing mitochondrial oxidative damage and a senescence-accelerated model. As a result, these rodents show premature aging and suffer age-related conditions such as cataracts, emphysema, scoliosis, tumors, and myocardiopathy relatively early in life (101,102,103,104). As for the retina, funduscopic analysis on these rats revealed choriocapillaris and atrophic areas in the RPE at the age of 45 days (105). Eventually, rats also exhibited thickening of BM, drusen and RPE detachments, and, by the age of a year, photoreceptor atrophy, decreased ERGs, destruction of the choriocapillaris with signs of fibrosis, and even hemorrhagic detachment of the retina secondary to neovascularization (101,105,106).
COMPLEMENT PATHWAY AND INFLAMMATION MEDIATORS
Chronic inflammation contributes to the onset and progression of multiple age-related degenerative diseases including AMD (107,108). The pathology of AMD lesions reveals signs of chronic persistent inflammatory damage such as macrophage infiltration and microglial accumulation. Plus, several acute-phase reactant proteins, in particular elements of the complement cascade, have been identified as molecular components of drusen shaft (69,109,110,111,112).
Interestingly, underneath the AMD-related inflammatory processes, there is a strong genetic connotation.
Polymorphisms in many of the genes that have been accounted for AMD encourage a proinflammatory state in the eye denoted by increased C-reactive protein and decreased inhibitory complement factors (113,114). Such polymorphisms include genes that code for factor H (CFH), complement component 2 (C2), complement component 3 (C3), complement factor B (FB), and complement factor I (FI) (115,116,117,118,119). Suffice to mention the particular case of the Y402H polymorphism in CFH to understand the great role, these genetic variations may have within AMD pathogenesis. Not only does such polymorphism increase the risk of AMD five- up to sevenfold, but it has also been found in half of AMD cases. Additional polymorphisms related to toll-like receptor (TLR) (e.g., TLR-3 and TLR-4) and chemokines (e.g., CX3CR1 and CCR3) have been also linked to the development and progression of AMD (120,121,122,123,124). In fact, the association between chronic inflammation and AMD is strong, and murine models that target specific aspects of the inflammatory process have been created to further elucidate this connection. These models are as varied as interesting and are discussed in detail in the following sections.
COMPLEMENT CASCADE
CFH Models
CFH negatively regulates the complement system by inhibiting the alternative pathway either by promoting factor I-mediated inactivation of C3b or by displacing factor Bb from the C3bBb complex and blocking the formation of C3 convertase (125,126). CFH dysfunction may lead to excessive inflammation and tissue damage, which may contribute to the pathogenesis of AMD in the retina (127). Patients and mice lacking functional CFH develop macular drusen similar to those seen in AMD (128,129,130,131). At the age of 2 years, these genetically engineered mice suffered decreased visual acuity, reduction in rod-driven ERG a- and b-wave responses, increased subretinal autofluorescence, complement deposition in the retina, and disorganization of photoreceptor outer segments. However, though there is an association between CFH and AMD, the CFH knockout mice do not display many of the hallmark AMD features. Contrary to other models of AMD, almost 30% of these animals showed a substantial thinning of their BM. Plus, since CFH itself is a main component of drusen, the loss of this protein may reduce the volume of sub-RPE deposits.
Transgenic CFH Y402H Mice
Recent studies have shown an increase risk of AMD in individuals with the Y402H polymorphism in the complement factor H gene (132). This polymorphism has been located to a region of CFH that binds heparin and C-reactive protein (124). In order to understand the role that such particular CFH mutation have in AMD pathogenesis, Ufret-Vincenty et al. (133) created transgenic mouse lines expressing the Y402H polymorphism under control of the human ApoE.
At the age of 1 year, transfected mice showed larger amounts of drusen-like deposits than those observed in either wild-type mice or even CFH knockout mice. While immunohistochemistry has revealed an increased amount of both microglial and macrophages in the subretinal space,
the electron microscopy showed thickening of the BM and deposits of C3d in the basement membrane. Contrary to the CFH knockout mice, transgenic CFH Y402H models did not show signals of photoreceptor atrophy. This occurrence might be explained either by the younger age at which these animals were examined (1 year vs. 2 years) or by the existence of some functional activity of CFH protein that disallowed a more severe dysregulation of the alternative pathway.
the electron microscopy showed thickening of the BM and deposits of C3d in the basement membrane. Contrary to the CFH knockout mice, transgenic CFH Y402H models did not show signals of photoreceptor atrophy. This occurrence might be explained either by the younger age at which these animals were examined (1 year vs. 2 years) or by the existence of some functional activity of CFH protein that disallowed a more severe dysregulation of the alternative pathway.
Transgenic Mice Overexpressing C3
C3 plays a major role in the complement activation and generation of immune responses by integrating signals from every complement-activating pathway. Since C3 can activate complement system and the complement system is a major risk factor for developing AMD, it is reasonable to expect that overexpression of this protein might lead to accelerated development of retinal pathology. To put this theory in practice, Cashman et al. (134) injected mice in the subretinal space with a recombinant adenovirus-expressing murine C3. Such delivery of C3 induced significant functional and anatomic changes that reproduce not only many of the features of AMD but also those of other retinal diseases. Examination of transfected eyes revealed more retinal changes (significantly increased vascular permeability, endothelial cell proliferation and migration, RPE atrophy, loss of photoreceptor outer segments, reactive gliosis, retinal detachment, and reduced retinal function) relative to those injected with a control adenovirus. Deposition of the membrane attack complex was observed on endothelial cells and photoreceptor outer segments.
This novel model may be useful in assessing the role of complement in retinal pathology and in developing anticomplement therapies for retinal diseases associated with complement activation. However, as for its accuracy to emulate AMD, there are some limitations that could not be ignored. In particular, the fact that C3-overexpressing animals demonstrated an unexpectedly high incidence of retinal detachments (a feature not shared with AMD) raises question about its similarity to the actual disease. Plus, it is unknown whether or not the mere activity of the transfected adenovirus could contribute to the pathologic features, thereby biasing the results.
C3a and C5a Receptor
Both C3 and C5 have been found in drusen (39,135). Although these proteins are best known for their role in opsonization and formation the membrane attack complex, their activity is multifaceted. In fact, mice lacking the receptors for these components showed smaller lesions in the laser-induced CNV model, decreased levels of VEGF expression, and impaired leukocyte recruitment (136). Such data support the hypothesis that activation of the C3a and C5a receptors contributes in the appearance of CNV in AMD.
Chemokines and Chemokine Receptors
Chemotactic cytokines, also named chemokines, are a family of small cytokines, which name is derived from their ability to induce directed chemotaxis in nearby responsive cells (137). Some chemokines are considered proinflammatory and can be induced during an immune response to recruit cells of the immune system to a site of infection, while others are considered homeostatic and are involved in controlling the migration of cells during normal processes of tissue maintenance or development. From a structural perspective, however, chemokines are frequently divided into four families: CXC, CX3C, CC, and C (138,139).
As for AMD, chemokines appear to be crucial in the subretinal microglia/macrophage accumulation observed and may also participate directly in the genesis of several abnormal processes such as retinal degeneration and CNV (108,140,141). The CCL2/CCR2 and CX3CL1/CX3CR1 ligand/receptor pairs excel among all the chemokines studied (142
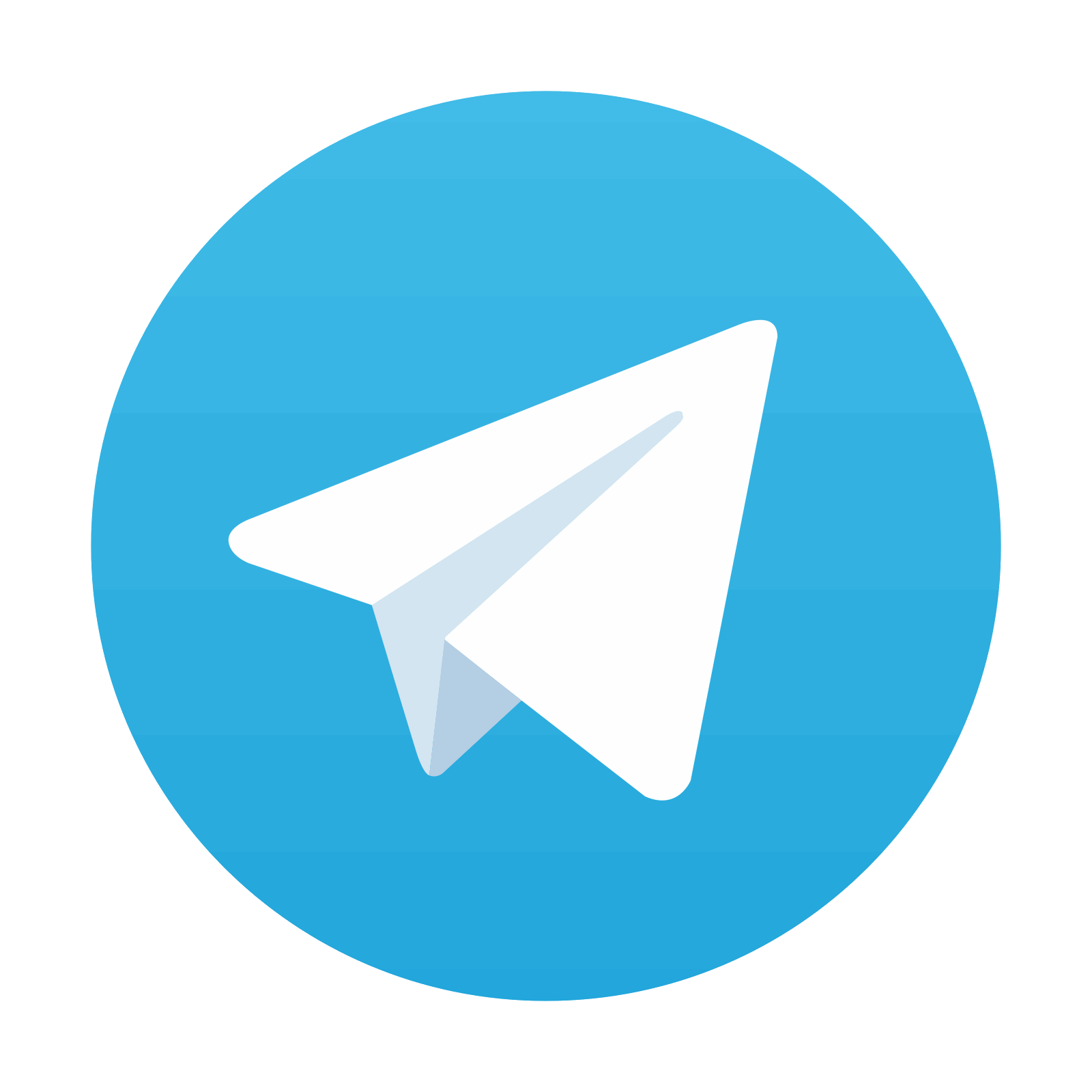
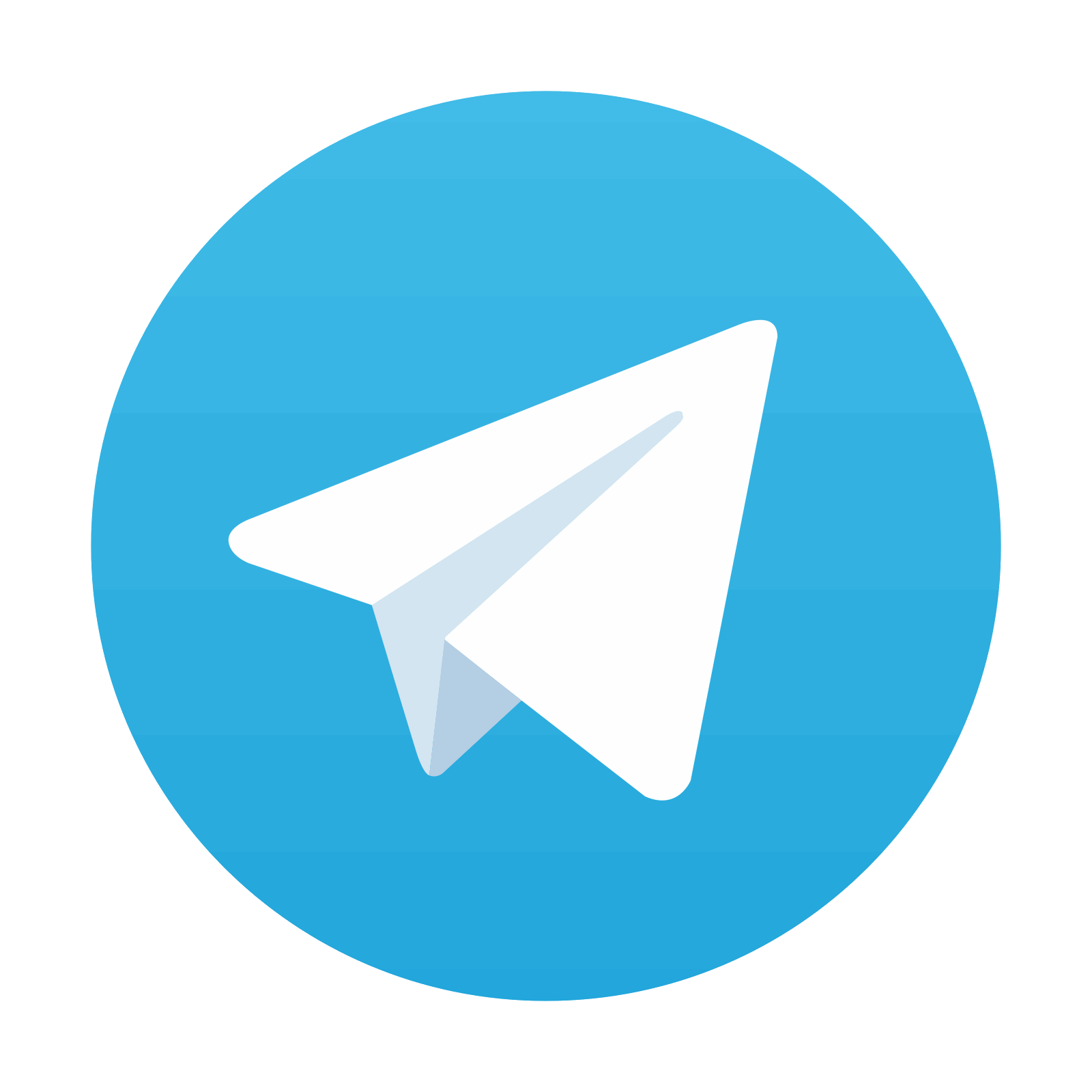
Stay updated, free articles. Join our Telegram channel

Full access? Get Clinical Tree
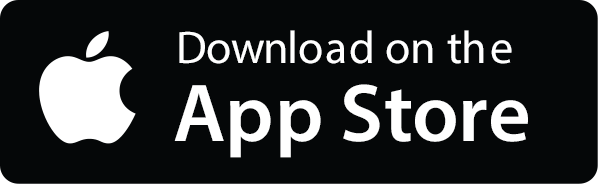
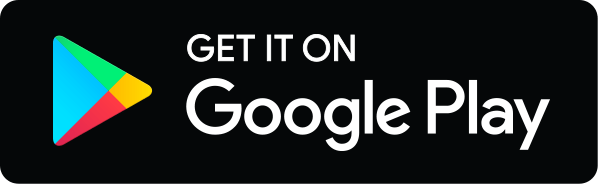
