Purpose
To investigate the expression, release, and proteolytic degradation of galectin-3 in patients with dry eye disease.
Design
Observational case series with a comparison group.
Methods
Tear washes and conjunctival impression cytology specimens were collected through standard procedures from 16 patients with dry eye and 11 age-matched healthy subjects. Galectin-3 content in tears was analyzed by quantitative Western blot, using recombinant galectin-3 protein to generate a calibration curve. The relative expression of galectin-3 and matrix metalloproteinase 9 (MMP9) was evaluated by quantitative polymerase chain reaction. The cleavage of galectin-3 was studied in vitro using activated recombinant MMP9 and protease inhibitors.
Results
The concentration of galectin-3 protein in tears, but not galectin-3 expression in conjunctival epithelium, was significantly higher in tears of patients with dry eye (0.38 ng/μg total protein, range 0.04–1.36) compared to healthy subjects (0.12 ng/μg total protein, range 0.00–0.41) ( P < .01). By Western blot, an intact (∼28.0 kDa) galectin-3 band was identified in tear samples from healthy subjects, whereas 50% of the dry eye samples were characterized by the additional presence of a partially degraded form (∼25.4 kDa). In our experiments, elevated expression of MMP9 in dry eye subjects correlated with the ability of active MMP9 to cleave galectin-3 from recombinant origin. Interestingly, cleavage of endogenous galectin-3 in tear samples was impaired using a broad-spectrum proteinase inhibitor cocktail, but not the pan-specific MMP inhibitor GM6001, suggesting the presence of proteases other than MMPs in promoting galectin-3 degradation in dry eye.
Conclusions
Our results indicate that release of cellular galectin-3 into tears is associated with epithelial dysfunction in dry eye, and that galectin-3 proteolytic cleavage may contribute to impaired ocular surface barrier function.
Disruption of epithelial barrier function is associated with a wide range of disorders that includes dry eye, a disease affecting millions of people worldwide and whose pharmacologic treatment remains unsatisfactory. While the intercellular junctions that connect individual cell membranes on mucosal surfaces have been clearly defined as components of the paracellular barrier, research performed during the last decade has redefined and highlighted the contribution of apical cell membranes as an additional layer of protection—the transcellular or glycocalyx barrier. Indeed, both molecular and structural changes of the glycocalyx in apical cells within the stratified squamous epithelium have been observed in drying diseases and appear to correlate with loss of barrier function at the ocular surface.
Transmembrane mucins (MUCs), such as MUCs 1, 4, and 16, are an essential component of the glycocalyx barrier in mucosal epithelia. They have single membrane–spanning regions with large extracellular glycosylated domains that form rod-like structures extending 200–500 nm above the plasma membrane, far above other glycoconjugates in the glycocalyx. Extensive research has shown that O-glycans, a form of glycosylation resulting from the addition of N-acetylgalactosamine to serine or threonine residues in the mucin ectodomain, are critical to preventing epithelial damage and infection of mucosal surfaces. At the ocular surface, transmembrane mucins contribute to provide boundary lubrication and to prevent the penetrance of extracellular molecules and pathogenic microorganisms onto the epithelia.
It is now clear that transmembrane mucins and their associated glycans promote the integrity of the epithelial barrier at the ocular surface by interacting on the apical glycocalyx with galectin-3. Galectin-3 is a multivalent β-galactoside-binding lectin known to promote formation of plasma membrane lattices with unique functions, concentrating specific glycoproteins within the lattice while excluding other molecules. Similarly to other members of the galectin family, galectin-3 is defined by the presence of an evolutionarily conserved carbohydrate-binding domain that mediates receptor binding. Critical to the multimerizing function of galectin-3 is the presence of an N-terminal domain that allows self-association through intermolecular interactions when bound to a multivalent ligand. Using site-directed mutagenesis, we have recently shown that maintenance of ocular surface glycocalyx integrity in vitro requires the N-terminal multimerization domain of galectin-3. To date, however, much remains unknown about the synthesis and structural integrity of galectin-3 in patients with altered ocular surface barrier function. In the present study, we investigated whether alteration of galectin-3 is associated with dry eye, by evaluating the expression and release of galectin-3 onto the ocular surface and examining whether galectin-3 undergoes proteolytic degradation in tears.
Methods
Study Population
This observational case series study was conducted in compliance with good clinical practice, Institutional Review Board (IRB) approval (Campus Bio Medico University of Rome, IRB #07/06.PARComEtCBM; Massachusetts Eye and Ear Infirmary, IRB #12-159H), informed-consent regulations, and the tenets of the Declaration of Helsinki. A total of 16 patients (10 women, 6 men) with dry eye disease were recruited from Fondazione GB Bietti and Massachusetts Eye and Ear Infirmary, with a mean age of 56.9 ± 11.0 years (range, 35–84 years). Within this population, 12 patients were aqueous deficient without meibomian gland disease (MGD), 3 were aqueous deficient with MGD, and 1 was aqueous sufficient with MGD ( Supplemental Table ; available at AJO.com ). Among the MGD patients, 1 had Sjögren syndrome and 2 had graft-vs-host disease (GVHD). None of the patients had chalasis. The subjects completed an IRB-approved questionnaire regarding history of ocular allergies, disease, surgery, contact lens wear, current medications, type and frequency of symptoms of dry eye and dry mouth, and the use of dry eye therapy. Inclusion criteria were symptoms of dry eye and at least 1 of the following objective signs: Schirmer I <10 mm at 5 minutes, decreased tear breakup time (TBUT) of ≤5 seconds, and positive vital dye staining of the conjunctiva or cornea. The degree of positive staining used for the diagnosis of dry eye corresponds to scores higher than 1 in the Oxford Scheme, or higher than 3 in the Bijsterveld score. Patients with a history of ocular surgery, topical antiglaucoma or anti-inflammatory therapy, or eye allergies were excluded. Eleven age- and sex-matched healthy subjects (7 women, 4 men) without dry eye symptoms, eye diseases, or history of eye surgery or contact lens wear were used as a control group. The mean age of the control group was 55.6 ± 11.5 years (range, 34–72 years).
Sample Collection
Tear fluid and conjunctival epithelium from healthy subjects and patients with dry eye disease were collected to determine galectin-3 protein levels, and galectin-3 and matrix metalloproteinase 9 (MMP9) messenger ribonucleic acid (mRNA) expression, respectively. Tear fluid was collected from unanesthetized eyes by pipetting 60 μL sterile saline (AddiPak [Teleflex, Morrisville, North Carolina, USA] 0.90% sodium chloride sterile solution) into the inferior fornix using a sterile transfer pipette, followed by movement of the eyes to mix the tear fluid content as described. The volumes acquired ranged between 14.0 and 72.6 μL (average 42.9 μL). Then, a drop of topical anesthetic (proparacaine hydrochloride, 0.5%, Alcaine; Alcon Inc, Ft Worth, Texas, USA) was applied to the eye. A sterile disk of nitrocellulose filter paper, 10 mm in diameter, was placed on the temporal bulbar conjunctiva, gentle pressure was applied to the disk for 15 seconds, and the disk was then carefully transferred into an Eppendorf tube containing TRIzol reagent (Gibco; Grand Island, New York, USA). All samples were promptly frozen at −80 C until the time of analyses.
Individual tear samples were centrifuged for 30 minutes at 18 000 g at 4 C using a microcentrifuge (centrifuge 5424R; Eppendorf, Hamburg, Germany). Protein concentration was determined using the Micro bicinchoninic acid (BCA) Protein Assay Kit (Thermo Scientific, Waltham, Massachusetts, USA), according to the manufacturer’s protocol.
Production of Recombinant Human Galectin-3
Recombinant human galectin-3 (rhGal-3) was expressed as previously reported. Briefly, heterologous protein expression was induced by addition of 0.3 mM isopropyl β-D-1-thiogalactopyranoside (IPTG) to Rosetta Escherichia coli clones carrying an rGal-3 vector, followed by incubation overnight at 15 C with shaking. The rhGal-3 was purified from lysates by affinity chromatography using lactosyl sepharose. To eliminate contaminating bacterial endotoxins, rGal-3 was further purified by polymyxin B affinity chromatography. The absence of lipopolysaccharide was confirmed using ToxinSensor Chromogenic LAL Endotoxin Assay Kit (GenScript, Piscataway, New Jersey, USA). Protein solutions were concentrated by filtration (VIVASPIN, Littleton, Massachusetts, USA), dialyzed against phosphate-buffered saline (PBS) buffer containing 10% glycerol and stored at −20 C. Protein concentration was determined using the BCA Protein Assay Kit (Thermo Scientific).
Immunoblotting
Tear samples containing 1–10 μg total protein were separated by electrophoresis on 10% sodium dodecyl sulfate–polyacrylamide gel electrophoresis (SDS-PAGE) gels and transferred onto nitrocellulose membranes (Bio-Rad Laboratories). Membranes were then blocked with 5% nonfat dry milk in Tris buffered saline with Tween (TBST) for 2 hours at room temperature, followed by incubation overnight at 4 C with the following primary antibodies diluted in 5% nonfat dry milk in TBST: anti-galectin-3 (H160; 1:2–3000; Santa Cruz Biotechnology, Dallas, Texas, USA) and anti-MMP9 (ab38898; 1:2000; Abcam; Cambridge, Massachusetts, USA). Following incubation with the corresponding peroxidase-conjugated secondary antibody (1:2000; Santa Cruz Biotechnology), positive binding was visualized using the SuperSignal West Pico Chemiluminescent Substrate (Thermo Scientific) on HyBlot CL autoradiography film (Denville Scientific, Inc, Metuchen, New Jersey, USA). When no band intensity was detected using the West Pico substrate, membranes were subsequently incubated with an enhanced and more sensitive substrate (SuperSignal West Femto Maximum Sensitivity Substrate; Thermo Scientific), which enables detection of low femtogram amounts of protein. Band intensities were quantified by densitometry (ImageJ software; National Institutes of Health, Bethesda, Maryland, USA; in the public domain, available at http://rsb.info.nih.gov/nihimage ).
Quantification of protein concentrations in tear fluid by immunoblotting was performed as previously described. Galectin-3 content (ng/μg total protein) in tears was determined using a standard curve based on signal intensity generated with different concentrations of rhGal-3 and 2 internal controls of rhGal-3 per gel when analyzing experimental samples.
RNA Isolation, cDNA Synthesis, and Quantitative Polymerase Chain Reaction
Total RNA was extracted from conjunctival impression cytology specimens using an extraction reagent (TRIzol; Life Technologies) according to the manufacturer’s protocol. The integrity and concentration of the total RNA were determined using a NanoDrop 2000 spectrophotometer (Thermo Scientific). One microgram of total RNA was treated with amplification-grade DNase I (Life Technologies) to eliminate residual genomic DNA and reverse transcribed into cDNA using random hexamer primers and reverse transcriptase (iScript; Bio-Rad, Hercules, California, USA) according to the manufacturer’s protocol.
Detection of gene expression in conjunctival impression cytology specimens was performed by quantitative polymerase chain reaction (qPCR) using PrimePCR primers for galectin-3 (unique assay ID: qHsaCID0008552) and MMP9 (unique assay ID: qHsaCID0011597). The qPCR reactions were carried out in triplicate in a 20 μL reaction volume using 1 μL cDNA, 1 μL primers, and the SYBR Fast master mix (KAPA Biosystems, Wilmington, Massachusetts, USA) in a Mastercycler ep realplex thermal cycler (Eppendorf, Hauppauge, New York, USA). The following parameters were used: 2 minutes at 95 C, followed by 40 cycles of 5 seconds at 95 C and 30 seconds at 60 C. All samples were normalized using glyceraldehyde-3-phosphate dehydrogenase (GAPDH) housekeeping gene expression (PrimePCR GAPDH primers; Bio-Rad). The comparative C T method was used for relative quantitation of the number of galectin-3 and MMP9 transcripts, selecting the relative mRNA levels in samples from healthy subjects as the calibrator.
Protease Cleavage Assays
For recombinant galectin-3 protease cleavage assay, 10 ng rhGal-3 was incubated with increasing concentrations of activated recombinant human MMP9 (rhMMP9; Calbiochem, San Diego, California, USA) in Dulbecco’s phosphate-buffered saline supplemented with magnesium and calcium (Thermo Scientific) at 37 C for various time periods as indicated. Reactions were stopped by the addition of Laemmli buffer and then subjected to boiling for 5 minutes. For endogenous galectin-3 protease cleavage assay, tear samples (3–5 μg total protein) from 3 patients with dry eye disease were incubated in PBS buffer at 37 C for 6 hours. In additional experiments, samples were supplemented with the pan-specific MMP inhibitor GM6001 (2 μM for rhGal-3; 40 μM for human tears) or a broad-spectrum proteinase inhibitor cocktail (cOmplete EDTA free; Roche Applied Science, Mannheim, Germany). The reaction products were analyzed by electrophoresis on 10% SDS-PAGE gels and visualized by immunoblotting.
Statistical Analyses
Statistical significance between normal and dry eye groups was evaluated with the Mann-Whitney U test for nonparametric data, using a P value of .05 as the threshold for significance. Correlations between TBUT and galectin-3 protein concentration or gene expression were determined using the Spearman rank correlation test. All statistical analyses were performed using InStat3 software (GraphPad Software, La Jolla, California, USA).
Results
Galectin-3 Levels Increase in Tears of Dry Eye Patients
Altered levels of galectin-3 in biological fluids and sera have been associated with the pathophysiology of a number of diseases, such as asthma, heart failure, and cancer. To study a potential role of galectin-3 in dry eye disease, we developed a defined immunoblot method to quantify the levels of endogenous galectin-3 in human tear samples. For this purpose, a standard curve from a 2-fold serial dilution series of rhGal-3 was produced to determine the linear range of detection for the galectin-3 antibody. Densitometric analysis of the protein bands revealed linear responses between 0.6 and 18 ng of rhGal-3 ( Figure 1 , Upper left). Next, we obtained the relative intensities of endogenous galectin-3 in human tears, normalized to the amount of total protein as previously described. We found a significant increase in galectin-3 levels in tears of dry eye patients. As shown in Figure 1 (Upper right) and the Supplemental Table (available at AJO.com ), the mean concentration of galectin-3 in control eyes was 0.12 ± 0.04 ng/μg total protein (range 0.00–0.41), whereas in dry eye it was 0.38 ± 0.08 ng/μg total protein (range 0.04–1.36) ( P < .01).
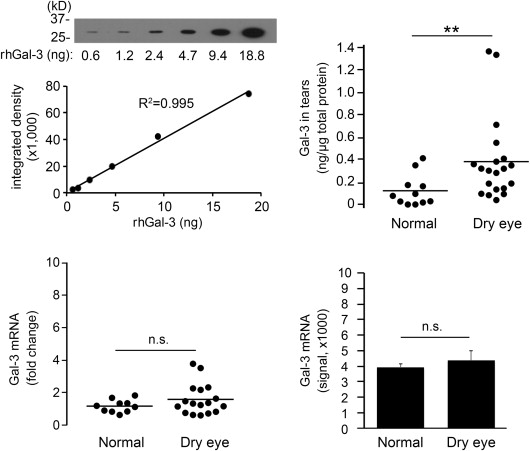
To determine whether enhanced expression of galectin-3 mRNA at the ocular surface was responsible for the increase of galectin-3 protein in tears, we performed qPCR on samples of human conjunctival epithelium obtained by impression cytology from the same population groups. Interestingly, no significant differences in expression were observed between the healthy and dry eye groups ( Figure 1 , Lower left). These results were consistent with data from a public database of microarray gene expression ( http://www.functionalglycomics.org ), showing no statistical differences in signal intensity for galectin-3 in conjunctiva of normal subjects and patients with dry eye disease ( Figure 1 , Lower right).
Galectin-3 Release Into Tears Correlates With Tear Film Instability
A key step in dry eye disease is the occurrence of an unstable tear film that eventually breaks up when in contact with an altered ocular surface epithelium. To determine a potential association between the amount of galectin-3 in tears and the occurrence of an unstable tear film, we performed a Spearman rank correlation test. As shown in Figure 2 (Upper panel), we found a significant correlation between galectin-3 protein concentration in tears and tear instability as measured by tear film breakup time (R = −0.4988; P < .01). In contrast, there was no correlation between galectin-3 gene expression in human conjunctival epithelium and tear film breakup time ( Figure 2 , Lower panel).
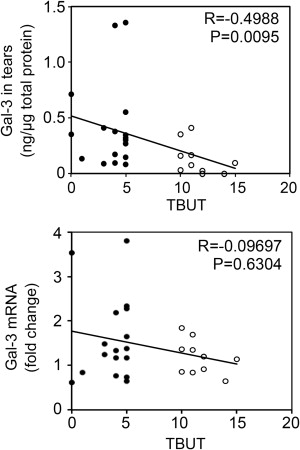
Galectin-3 Is Partially Degraded in Tears of Dry Eye Patients
Proteolytic degradation of galectin-3 is thought to impair the ability of the molecule to multimerize in the presence of binding partners, as well as to abrogate biological functions that depend on the ability of the lectin to form lattices. The human gelatinases MMP2 and MMP9 have been shown to induce cleavage of galectin-3 at the Ala 62 -Tyr 63 bond in the N-terminus, resulting in a 22-kDa cleavage product lacking the multimerization domain known as galectin-3C ( Figure 3 , Upper panel). In our experiments, analysis of galectin-3 in human tear samples by immunoblot revealed the presence of a major galectin-3 band with a molecular weight of 28.0 kDa ( Figure 3 , Lower left). Interestingly, we also detected the presence of a partially degraded form with a molecular weight of 25.4 kDa. Quantification of the ratio between galectin-3C and galectin-3 revealed a significant increase in tears of dry eye patients compared to tears of healthy subjects ( Figure 3 , Lower right; Supplemental Table ). Further, whereas higher values of galectin-3 were not associated with a particular etiology, the ratio between galectin-3C and galectin-3 in tears of patients with Sjögren syndrome and GVHD was significantly higher than in other patients (data not shown).
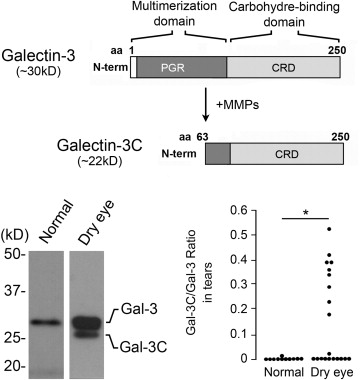
Degradation of Galectin-3 in Human Tears Involves Protease Activity
Previous studies have shown that MMP9 activity is significantly higher in patients with dry eye disease. Therefore, to further explore the mechanism by which galectin-3 is degraded in human tears, we analyzed the levels of MMP9 expression in conjunctival impression cytology samples collected from our study population. Consistent with previous data, we found a significantly higher expression of MMP9 mRNA in samples from dry eye patients compared to normal subjects ( Figure 4 , Upper panel). In subsequent experiments, we tested whether active human rhMMP9 would cleave rhGal-3. Our results showed that active MMP9 promotes galectin-3 cleavage in a concentration- and time-dependent manner, producing a 25-kDa cleavage product ( Figure 4 , Middle and Lower panels). As expected, the pan-specific MMP inhibitor GM6001 attenuated the degradation of full-length galectin-3 in control experiments.
