19
Acoustical and Electrical Speech Processing
Bruce J. Gantz, Christopher W. Turner, and Kate E. Gfeller
Combining electrical and acoustical speech processing is the newest application of cochlear implant technology. Speech perception performance in quiet with a cochlear implant in postlingually deafened adults and prelingually deafened children has gradually improved over the past 20 years (Gantz et al, 2002; Svirsky et al, 2000; Tomblin et al, 1999). A combination of factors, including improved implant speech processing algorithms, implant design, and importantly the residual hearing of those receiving an implant, has improved performance almost threefold. The average postlingually deafened adult can converse on the telephone and understand almost 50% of monosyllabic words and over 80% of words in sentences. Results continue to be variable for the individual patient. Prelingually deafened children have also benefited from cochlear implant technology. When an implant is stimulated prior to 13 months of age, language development appears to be within normal-hearing language development norms (Tomblin et al, 2005). These results have stimulated an interest in expanding cochlear implants to those with more residual hearing because duration of deafness and residual hearing have been shown to be predictive parameters of performance with an implant (Gantz et al, 1993; Rubinstein et al, 1999). The remarkable word understanding scores obtained by these groups of individuals have enabled those with less than profound deafness to be considered for implantation.
♦ Advantages and Disadvantages of Cochlear Implants: The Role of Acoustic Hearing
The decision to undergo implantation surgery, however, involves some trade-offs, as the patients’ residual acoustic hearing is usually no longer usable, and only electric stimulation is available. Residual low-frequency acoustic hearing has some important advantages compared with electrical signal processing available in cochlear implants. Expanding selection criteria for standard cochlear implants to include those with substantial residual low-frequency hearing should be viewed with some caution.
Several factors should be considered before the decision to destroy residual acoustic hearing is undertaken. The electrical signal-processing algorithm that provides important information for speech perception in quiet is reported by users of cochlear implants as “mechanical” or “raspy” when compared with their memories of acoustic hearing. In addition, some report that many of the aesthetic qualities of sound are diminished. This loss of aesthetic quality of sound is most likely related to a decrease in the ability to perceive the pitches of sounds (Gfeller et al, 2002). The loss of pitch perception is primarily a consequence of the limited spectral resolution of current cochlear implants. This does not, however, appear to be a limitation for understanding speech in quiet for the most successful implant users (Fishman et al, 1998). Understanding speech in background noise requires spectral resolution even finer than that required for understanding speech in quiet (Fu et al, 1998). Even the most successful implant users only realize perhaps six to eight channels of distinct “place-frequency” information across the entire spectral range, and this deficit in spectral resolution has a direct negative consequence on the implant user’s ability to understand speech in background noise (Friesen et al, 2001).
With regard to music, implant recipients using conventional long electrode implants typically have shown much poorer performance than normal-hearing persons on several pitch-related tasks, including detecting pitch change (frequency difference limens), perception of direction of pitch change (higher or lower), and discrimination of brief pitch patterns. Cochlear implant (CI) recipients using conventional long electrodes almost always require considerably larger frequency differences than normal-hearing adults for detecting pitch change (frequency difference limens) as well as the direction of a pitch change (i.e., whether the second pitch of a pair of notes is higher or lower than the first) (Fishman et al, 1998; Gfeller et al, 2002).
Poor pitch resolution has a detrimental effect on the aesthetic quality of musical sounds, and it also impedes the CI user’s ability to do a very basic listening task that normal-hearing people tend to take for granted—the ability to recognize familiar melodies such as “Happy Birthday,” “The Star Spangled Banner,” holiday songs, and spiritually significant songs when no lyrics are provided. Melody recognition requires the listener to hear a sequence of pitches and accurately perceive the direction of pitch change (higher or lower) as well as the magnitude of each pitch change. Because melodies are made up of sequential pitch patterns, poor perception of pitch, as is common for CI recipients who use the conventional long electrode, has negative implications for this perceptual task (Friesen et al, 2001; Fu et al, 1998; Gfeller et al, 1991, 2002; Pijl & Schwartz, 1995). In short, because acoustic hearing has an important role in pitch resolution, and those hearing tasks facilitated by accurate pitch perception, such as understanding speech in noise, the loss of residual hearing is an important clinical consideration when determining the most appropriate options for patients with severe hearing losses.
The loss of residual acoustic hearing during implantation is the result of a combination of factors including the technique used to create the cochleostomy as well as the size of cochleostomy performed. In addition, the diameter, stiffness, and length of standard intracochlear electrodes may induce substantial intracochlear damage to the basilar membrane and cochlear hair cells as they advance around the upper basilar turn during insertion. This damage is likely caused by forces against the outer cochlear wall during the insertion (Roland, 2005).
♦ Development of Cochlear Implants that Combine Residual Low-Frequency Acoustic Hearing with High-Frequency Electric Speech Processing
In early 1995 we began to explore the possibility of placing a shortened small-diameter electrode into the scala tympani in an attempt to combine residual low-frequency acoustic hearing with high-frequency electrical speech processing of the cochlear implant. Animal research by Shepherd and his colleagues had demonstrated that electrodes placed in cats with residual hearing resulted in damage adjacent to the electrode, whereas the apical organ of Corti remained undisturbed. Low-frequency acoustic hearing was preserved in these animals (Ni et al, 1992; Xu et al, 1997). It was our intent to develop a limited electrode that could be placed in the basal turn of the scala tympani without inducing damage by advancing around the upper basilar turn. The Cochlear Corporation (Sydney, Australia) built the first electrodes that were 6 mm in length and had a 0.2 × 0.4 mm diameter. The implant had a short 6 mm, six-channel electrode and a secondary reserve standard length (24 mm) electrode containing 16 active electrodes. The reserve electrode was to be used if the short electrode induced a profound deafness without providing significant speech perception. This electrode was placed in the mastoid during the surgery.
The concept of hearing preservation and cochlear implantation was presented at the National Institutes of Health–National Institute on Deafness and Other Communication Disorders (NIH-NIDCD) 10th anniversary meeting in Bethesda, Maryland in October 1998 (Gantz, 1998). In early 1999, the first clinical subject was implanted with the device. Subsequently, two others also received the 6-mm electrode. All three subjects reported that the percept was very high pitched and one thought it was unpleasant. These results were presented at the 5th International Conference on Cochlear Implants in Miami in February 2000 (Gantz and Turner, 2000). A small but significant increase in speech understanding was observed from the addition of the electrical stimulation. The electric speech processing sound was perceived as very high pitched and unpleasant to some. The most important finding of this initial trial was that residual acoustic speech perception and pure tone audiometric testing were unchanged from preoperative levels. Because of the unpleasant pitch percept, it was decided to lengthen the electrode and move the active electrodes into an area in the cochlea closer to a frequency of about 2500 to 3500 Hz according to the Greenwood place frequency map (Greenwood, 1990). The 10-mm electrode was developed with the same diameter dimensions (0.2 × 0.4 mm) as the 6-mm design. The active electrodes were placed toward the apical end (Fig. 19–3). Results with the 10-mm (Iowa/Nucleus Hybrid Cochlear Implant; Cochlear Corporation, Sydney, Australia) have been published (Gantz and Turner, 2003). Volunteers implanted with this device have usable acoustic hearing up to 750 Hz, and the electrical stimulation provides the patient with high-frequency speech information (1500–6000 Hz). Thus, these subjects perceive sound via a combined acoustic and electric (A +E) mode. They report that the aesthetic qualities of sound is preserved, they enjoy music, and they exhibit better speech recognition in background noise (Turner et al, 2004).
♦ Surgical Technique for Implanting Iowa/Nucleus Hybrid Implant
The Iowa/Nucleus Hybrid Implant was designed to limit damage to the scala media. The intracochlear electrode has a reduced diameter of 0.2 ×0.4 mm. Unique features of the electrode include a Dacron collar to limit the intracochlear placement to 10 mm and a titanium marker to orient the electrode contacts, thus ensuring the correct alignment of the electrodes adjacent to the modiolus. The length of the electrode was limited to 10 mm to prevent injury to the ascending basal turn of the cochlea and prevent the electrode from curling on itself on insertion. A mastoidectomy is done, the bony seat is created for the electronic package, and the facial recess is opened similarly to implantation of a standard cochlear implant. The bony overhang of the round window niche is removed with a 1.8-mm diamond burr exposing the round window membrane.
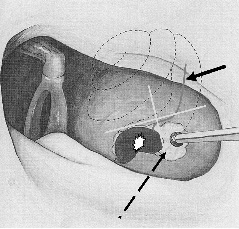
Figure 19–1 Position of cochleostomy in the inferior-posterior quadrant of a box created by drawing a line at the superior margin of the round window and a perpendicular line at the inferior margin of the round window (dashed line). Basilar membrane position (solid arrow), round window membrane (star).
The strategy for creating the cochleostomy and entering the scala tympani should involve surgical detail similar to performing a “drill-out” stapes for otosclerosis. Bleeding must be controlled and suction must be avoided once the inner ear is opened. The cochleostomy is created anterior-inferior to the round window. To standardize the placement of the cochleostomy, it is suggested that the cochleostomy be placed in the anterior-inferior quadrant of a box created by drawing a line at the superior margin of the round window and one that crosses perpendicular at the inferior aspect of the round window (Fig. 19–1).
Creation of the cochleostomy is begun in the inferior portion of the quadrant, slowly saucerizing the otic capsule bone with a 1-mm diamond burr. Approaching the scala tympani from a caudal direction ensures that the cochleostomy will be inferior to the equator of the scala and thus avoiding the basilar membrane, and spiral ligament (Fig. 19–2). It should be remembered that the basal turn of the cochlea is oriented oblique in the temporal bone with the helicotrema usually positioned deep to the tensor tympani muscle, and the ascending basilar turn can be deep to the level of the internal carotid artery. As bone is slowly removed over the scala tympani, the lumen will appear as bluish or a faint gray hue. If the cochleostomy is too far superior, adjacent to the spiral ligament, a whitish color will be evident. Drilling inferior will permit entrance into the scala tympani.