Fig. 26.1
Acoustic rhinometry equipment (Rhinoscan SRE 2000, Interacoustics A/S, Assens, DK)
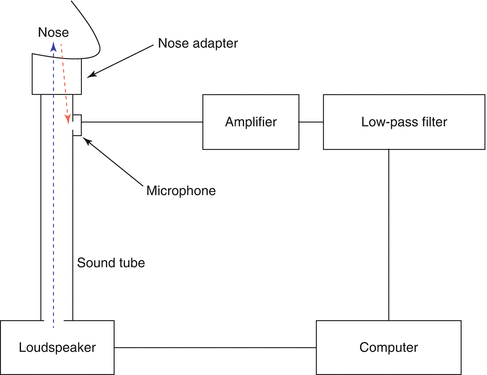
Fig. 26.2
Schematic representation of an acoustic rhinometry circuit
The changes in cross-sectional area of the airway affect acoustic impedance. Since the nasal cavity is not a straight pipe and has an irregular and complex anatomy, acoustic waves show different reflection patterns at differing cross-sectional areas on their route. Although the mathematical background of the analysis is very complex and will be detailed to some extent later in this chapter, computerised calculations basically give two parameters for a given cross-section: Comparison of the amplitudes of the sent and reflected sound waves gives cross-sectional areas, while the time difference between the sent and reflected sound waves gives the distance of a given cross-sectional area to the reference point. These data are then combined and an area–distance curve is obtained. The areas here define the cross-sectional areas that are vertical to the acoustic pathway, i.e. the way that acoustic waves follow in the nasal cavity. Cross-sectional area of a given section is plotted on vertical, while distance of that section to the reference point is plotted on horizontal axis of the graph. In order to evaluate the nasal valve region better, vertical axis can be plotted in logarithmic scale.
26.5 26.5 Test Technique
Classic acoustic rhinometry utilises single impulse and one microphone (transducer) setup. However, there are other methods that use two microphones (Louis et al. 1994) or devices that use continuous acoustic stimulation (Djupesland and Lyholm 1997). Although the theory is identical to that of the single-impulse method and the test technique is similar, a continuous wide-band noise model allows for almost real-time adjustment of the equipment since a visual output is generated more than 20 times per second (Straszek 2008).
By means of the modern computer software, the use of acoustic rhinometry is relatively simple. Since external noise, temperature and humidity have potential effects on measurements, testing room should have standard environmental conditions. Test should be applied by experienced staffs that are aware of the recommendations for reliable testing (Fisher et al. 1995). Both sides should be tested separately. Single measurement takes about a few seconds, and whole testing process ends in a couple of minutes. After the device is switched on and computer program is opened, calibration is simply done by following the on-screen instructions that are provided by the manufacturer. Calibration should be done every time the device is opened. The patient should have a sitting position and position should not be changed during the tests. Swallowing and breathing should be avoided during the measurements. Any kind of secretions can narrow the airway and affect the measurements. Hence, nasal cavity should be cleaned off from secretions before the test (Cakmak et al. 2001). Several types of nose adapters with different rim shapes are available (Fig. 26.3). A nose adapter that would best fit to the shape of the patient’s nostril should be chosen and attached to the probe. To avoid acoustic leak, a medical sealant gel should be applied circumferentially to the edge of the nose adapter, providing an air secure contact between the nose adapter and the nostril (Hamilton et al. 1997). Nose probe should be placed accordingly to prevent acoustic leak, but distortion of the nose or changes in position of the nose probe should be strictly avoided (Fig. 26.4).
Measurements should be repeated for at least three times to obtain the most correct results. By this way, erroneous measurements due to acoustic leakage or distortion of the vestibule owing to incorrect positioning of the probe can be detected and eliminated.
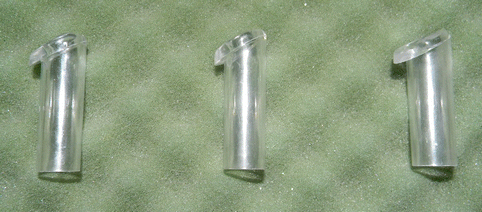
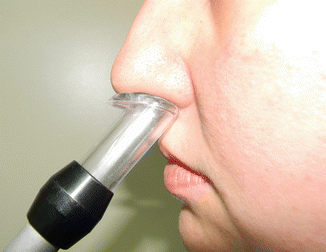
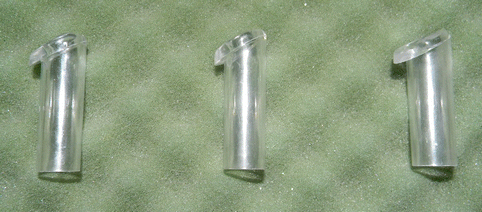
Fig. 26.3
Various types of nose adapters are designed to achieve a better fit to shape of the right or left nostril
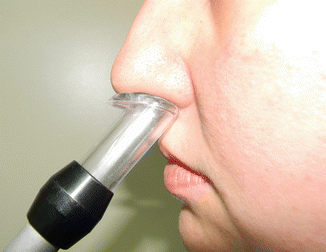
Fig. 26.4
During the measurements, nose probe should be placed accordingly to prevent acoustic leak. Distortion of the nose should be avoided
The operator should be aware of the above-mentioned testing principles and instructions. Besides the operator errors, complex anatomy of the nasal cavity, physical limitations of the AR and factors inherent to the AR algorithms may influence the measurements and lead to systematic errors. Nasal cavity has a complex geometry consisting of cartilaginous and bony framework covered with erectile tissue and mucosa including a narrow segment of nasal valve at the anterior part and sinus ostia more posteriorly. Narrow segments at anterior parts negatively affect the measurements of more posterior parts, and this leads to a potential problem since the narrowest part of the nasal cavity, the nasal valve, is on anterior part of the cavity. Similarly, ostia of the paranasal sinuses affect AR measurements, and cross-sectional areas behind the paranasal sinus ostia are overestimated. In order to understand the results of AR measurements, we will try to take a closer look to the cross-sectional area–distance curve.
26.6 26.6 Cross-Sectional Area–Distance Curve
In order to interpret AR results correctly, it is essential to know how some anatomical structures in nasal cavity appear on AR area–distance curves and how these structures’ localisations and size affect the area–distance curve.
In a typical AR area–distance curve, there is a minimum on the junction between the nose adapter and the nostril (Fig. 26.5). This minimum is generally not considered as a “minimum” and hence not termed as the “1st minimum”, since it occurs at “0 cm” which is the start point of the nose. First minimum after the nostril represents the nasal valve region (Fig. 26.5).
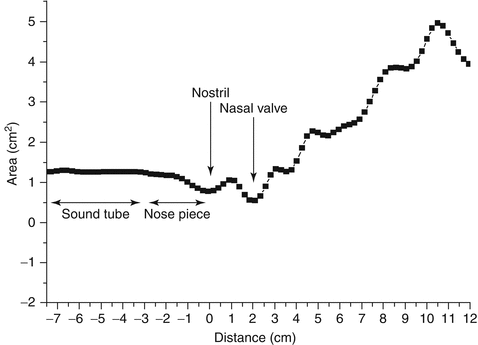
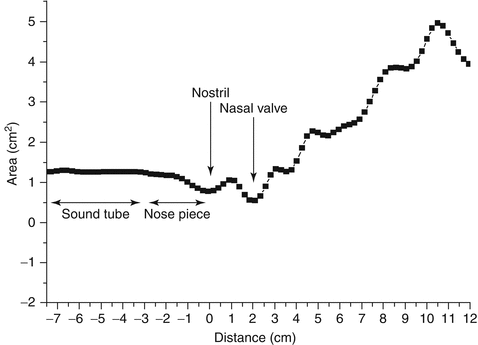
Fig. 26.5
A typical AR cross-sectional area–distance curve. Start point of the nasal cavity (nostril) is accepted as “0 cm”. Horizontal axis gives the distance of a given cross-sectional area that is perpendicular to the acoustic axis to the nostril. The cross-sectional area of that section is plotted on vertical axis
There is no clear consensus on interpretation of AR results, even in healthy humans. Inspection of the literature reveals that up to four local minima have been commonly observed on the AR area–distance curves, and different terms have been used to define these minima. The terms “1st constriction, I-notch, CSA1, MCA, start of isthmus region, start of valve region or ostium internum” have been used for the first minimum, which was attributed to the nasal valve; “2nd constriction, C-notch, CSA2, inferior concha or piriform aperture” have been used for the second minimum, which was attributed to the head of the inferior turbinate; and “CSA3” have been used for the third minimum, which was usually attributed to the middle turbinate (Hilberg 2002; Cakmak et al. 2003b). However, recent experimental studies with nasal cavity models and clinical studies revealed that the second and third minima on cross-sectional area–distance curves do not represent an anatomical point, and hence, most of these terms might be used inappropriately (Celik et al. 2004; Cankurtaran et al. 2003, 2007; Cakmak et al. 2001, 2003a, 2003b 2005a, 2005b; Tarhan et al. 2005).
In healthy humans, cross-sectional areas measured by different imaging modalities, such as CT and MRI, were compared with AR cross-sectional area measurements, and the techniques were found to give comparable results especially on the anterior part of the nasal cavity (Cakmak et al. 2003b, 2005b; Terheyden et al. 2000; Hilberg et al. 1993; Min and Jang 1995; Gilain et al. 1997; Corey et al. 1997; Dastidar et al. 1999). Regarding the validation of AR curve with imaging modalities, a methodological issue has to be concerned. Areas calculated on AR measurements are the cross-sectional areas that are perpendicular to the sound wave propagation axis (acoustic axis). In some of the validation studies, images of the nose were taken perpendicular to the base of the nose, not to the acoustic axis, and the reference point to be used in distance measurements was either chosen as the anterior nasal spine (Hilberg et al. 1993; Gilain et al. 1997) or tip of the nose (Dastidar et al. 1999) or even not clearly defined (Corey et al. 1997). Studies that do not care to the sloped anatomy of the nasal cavity or use different reference points may lead into significant errors when interpreting the AR curve.
In a study on healthy humans, the actual cross-sectional areas of the nasal cavity together with the actual locations of the nasal valve, the head of the inferior turbinate, the head of the middle turbinate, the openings of the ostia of the maxillary and frontal sinuses and the choanae were calculated from computed tomography sections perpendicular to the curved acoustic axis of the nasal passage (Cankurtaran et al. 2007). The findings were then compared with the corresponding cross-sectional areas measured by AR. Comparison of the CT- and AR-derived area–distance curves both before and after decongestion revealed that the nasal valve is identified by a pronounced minimum (the first minimum after the nostril) on the CT- and AR-derived area–distance curves. However, neither the head of the inferior turbinate nor the head of the middle turbinate could be distinctly identified on the CT area–distance curves of healthy humans. The same held true for the AR measurements in both cadaver cast models and healthy humans (Cakmak et al. 2005b; Cankurtaran et al. 2007). The second and third minima on the AR area–distance curves did not correspond to the actual locations of the head of the inferior turbinate and the head of the middle turbinate determined from CT, neither before nor after decongestion (Cakmak et al. 2005b; Cankurtaran et al. 2007).
In a study that used cast model of the nasal cavity of a cadaver, Cakmak et al. demonstrated that AR was able to detect changes in cross-sectional area larger than approximately 0.19 cm2 and 0.38 cm2 at the head of the inferior turbinate and the head of the middle turbinate, respectively (Cankurtaran et al. 2007). This finding suggests that AR cannot resolve any change in the cross-sectional area of the nasal passage at each of these specific anatomic sites that is smaller than the corresponding limit. In addition, the ability of AR in measuring abrupt changes in cross-sectional area is poor, because of the limited spatial resolution and the long rise distance of the technique (Celik et al. 2004; Cakmak et al. 2005b).
In summary, with the exception of the first minimum after the nostril, which represents the nasal valve, the subsequent minima on the AR area–distance curves for both non-decongested and decongested nasal cavities do not correspond to any anatomic structure in the healthy human nose (Figs. 26.6a, b and 26.7a, b). These minima are formed because of the acoustic resonances in nasal cavity behind the nasal valve region. Effects of some important anatomical landmarks on AR cross-sectional area–distance curves are summarised below.
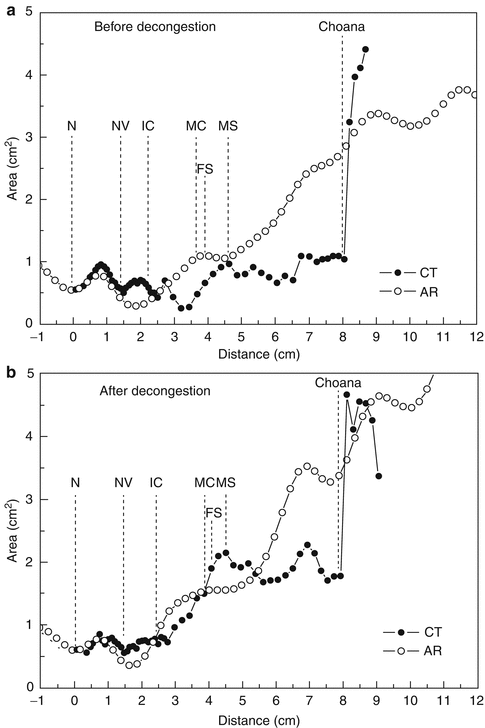
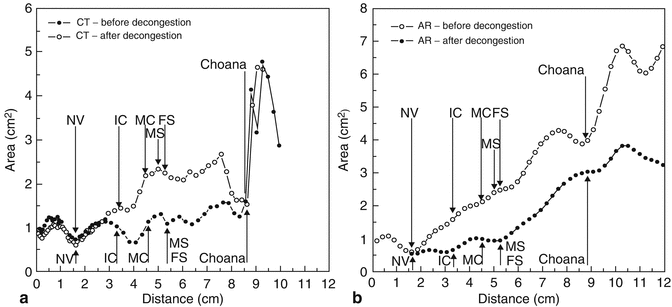
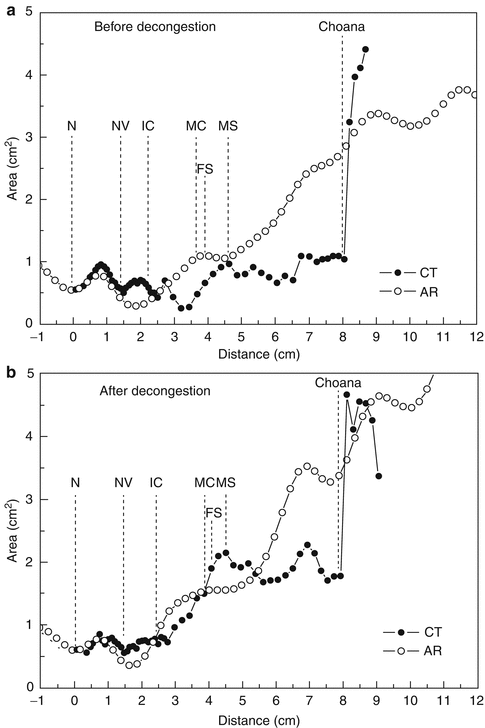
Fig. 26.6
The cross-sectional area–distance curves of a healthy human’s nasal cavity as determined by computed tomography and acoustic rhinometry, before (a) and after (b) decongestion. Actual locations of the anatomical structures are determined on computed tomography sections and depicted on the graphs with vertical dashed lines. The ability of acoustic rhinometry to detect the anatomical structures in nasal cavity can be seen. Acoustic rhinometry fails to quantify the volume change after decongestion (CT cross-sectional area–distance curve as determined by computed tomography, AR cross-sectional area–distance curve as determined by acoustic rhinometry, N nostril, NV nasal valve, IC head of the inferior concha [turbinate], MC head of the middle concha [turbinate], FS frontal sinus ostium, MS maxillary sinus ostium)
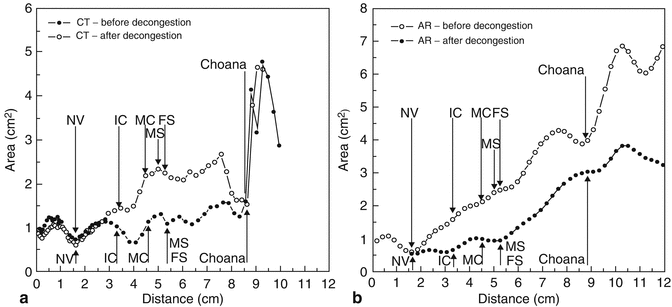
Fig. 26.7
The cross-sectional area–distance curves of a nasal cavity before and after decongestion, as determined by computed tomography (a) and acoustic rhinometry (b). Actual locations of the anatomical structures before and after decongestion are determined on computed tomography sections and depicted on the graphs with arrows. Acoustic rhinometry fails to detect the localisations of the anatomical structures except for the nostril and nasal valve (CT cross-sectional area–distance curve as determined by computed tomography, AR cross-sectional area–distance curve as determined by acoustic rhinometry, N nostril, NV nasal valve, IC head of the inferior concha [turbinate], MC head of the middle concha [turbinate], FS frontal sinus ostium, MS maxillary sinus ostium)
26.7 26.7 Nasal Valve
The nasal valve area is widely accepted as the most important part of the nasal passage with respect to its essential role in respiratory physiology. Boundaries of this triangular region are formed by the caudal septum (medial wall), caudal edge of the upper lateral cartilages and head of the inferior turbinate (lateral wall) and floor of the nose (inferior wall). Nasal valve is the narrowest part of the nasal passage and functions as an essential regulator of nasal airflow. The accuracy of AR measurements in the anterior part of the nose, which contains the nasal valve, is substantial in terms of the value of this method in rhinology. Individual anatomical variations of the anterior narrow segment might significantly limit the role of AR as a diagnostic tool for the entire nasal cavity.
As mentioned above, the nasal valve is identified by a pronounced minimum (the first minimum after the nostril) on the AR area–distance curve. Experimental studies on pipe models and nasal cavity models have shown that AR gives an accurate measure of the distance from the nose adapter to the narrow segment that simulates nasal valve, and AR measurements of the anterior nasal passage are reasonably accurate if the nasal valve area is within normal adult ranges (Cankurtaran et al. 2003; Cakmak et al. 2005a). Clinical studies that compare the cross-sectional areas derived by AR and by imaging modalities such as computed tomography and magnetic resonance imaging also showed that AR is a valuable method for measuring nasal valve area (Cakmak et al. 2003b; Cankurtaran et al. 2007; Terheyden et al. 2000; Hilberg et al. 1993; Min and Jang 1995; Gilain et al. 1997; Corey et al. 1997). These studies noted significant correlations between the cross-sectional areas obtained by imaging modalities and AR, with particularly high agreement in the anterior part of the nasal cavity and nasal valve. For the area of the nasal valve, agreement between the AR and imaging techniques was apparent when imaging was obtained perpendicular to the acoustic axis that follows the curve of the nasal passage through the centre of the curved airway (Cakmak et al. 2003b).
One of the best-recognised problems with acoustic pulse-response analysis is its inability to precisely measure the cross-sectional areas beyond narrow apertures. Concomitantly, the accuracy of AR measurements of the nasal cavity depends greatly on nasal passage anatomy, especially that of the narrowest section. In model studies, the cross-sectional area and the length of the narrow segment have been shown to be the factors that most significantly influence the accuracy of AR (Celik et al. 2004). When the cross-sectional area and the length of the narrowest part of the passage were relatively small and short, the probability of measurement error was higher. It is well established that the area of a region beyond a severe constriction may not be measured accurately by AR, and a narrowing in the anterior part of the nasal cavity causes errors in AR-derived areas posterior to the site of constriction (Hilberg et al. 1989; Cankurtaran et al. 2003; Cakmak et al. 2001; Hilberg and Pedersen 2000; Hilberg et al. 1998). The results obtained for living human subjects suggest that when the nasal valve passage area is within the normal adult range, AR is a valuable method for measuring the cross-sectional areas of the nasal cavity anterior to the paranasal sinus ostia (Cankurtaran et al. 2007; Tarhan et al. 2005). In other terms, accuracy of AR measurements is closely related with the narrowest section of the nasal passage, the nasal valve, and the passage area of the nasal valve is the most important limiting factor when quantifying the geometry of the anterior nasal cavity with AR. The effects of nasal valve passage area on accuracy of AR measurements were examined by Cankurtaran et al. using simple pipe models with a constriction (Cankurtaran et al. 2003). These authors demonstrated that the constriction reflects most of the incident sound power. In models with constrictions of small passage area, the high-frequency components of the acoustic pulse generated by AR equipment do not reach the portion of the model beyond the constriction, because these waves are reflected back from the barrier created by the constriction. This finding is of vital importance for AR because the transmitted sound waves probe, and hence provide information about, the cross-sectional area posterior to the constriction. Accordingly, an examiner should expect relatively higher degrees of error when measuring the cross-sectional area of a nasal cavity model beyond a constriction of small passage area. Since AR measures the intensity of reflected sound waves and compares this with the intensity of incident waves, AR-measured cross-sectional areas beyond the constriction (a nasal valve that is smaller than normal adult size) are underestimated, and the corresponding area–distance curve shows pronounced oscillations (Celik et al. 2004; Cakmak et al. 2005a).
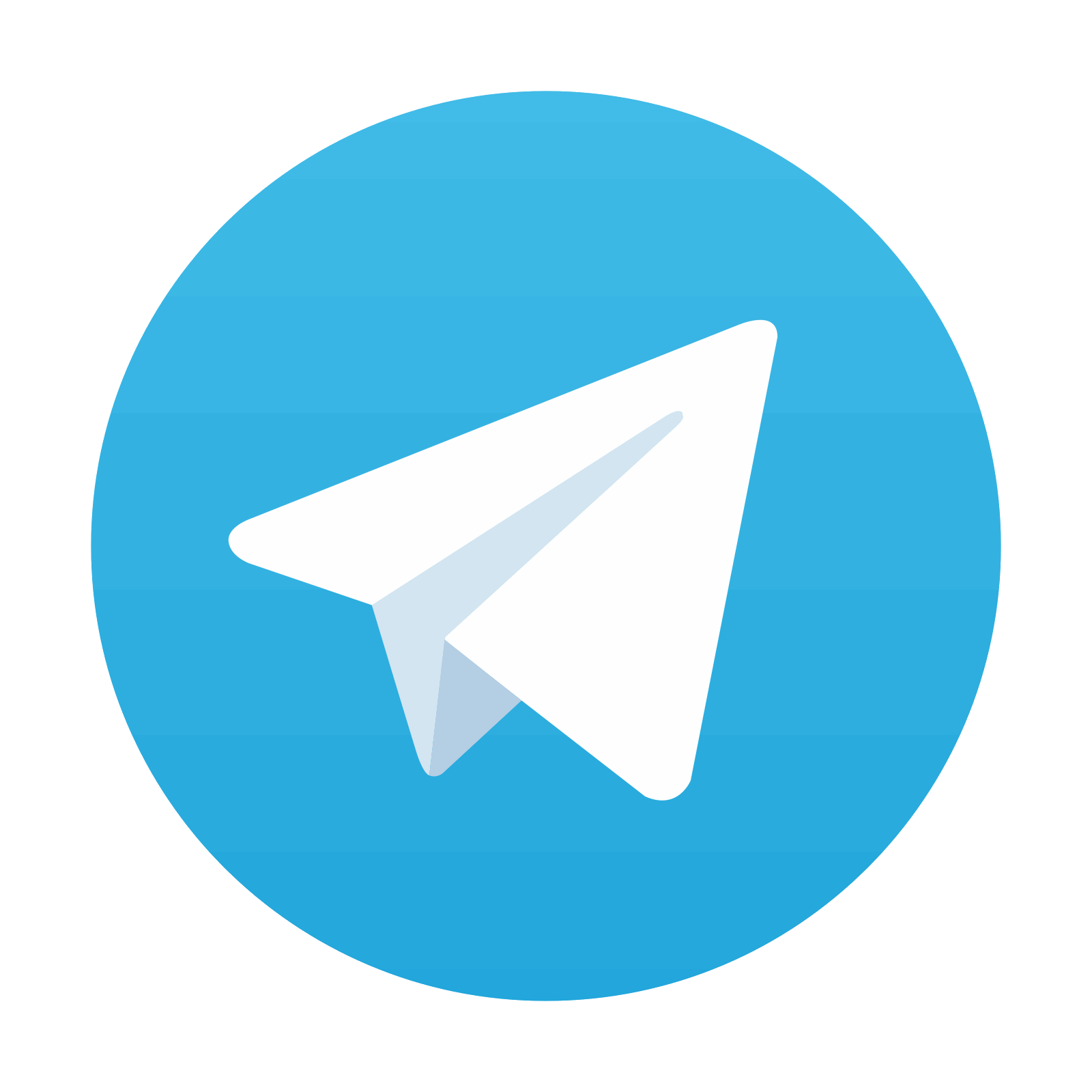
Stay updated, free articles. Join our Telegram channel

Full access? Get Clinical Tree
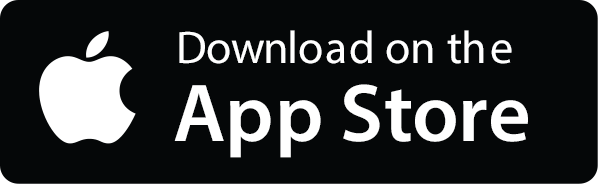
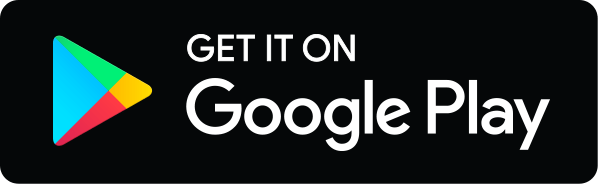