Purpose
To evaluate the objective accommodative response, change of aberrations, and depth of focus in eyes implanted with the Crystalens accommodative intraocular lens (IOL) at different accommodative demands.
Design
Prospective, observational study.
Methods
Eleven cataract patients (22 eyes) who underwent implantation of a Crystalens accommodative IOL, and control groups of 9 normal subjects (17 eyes) and 17 pseudophakic patients (17 eyes) implanted with monofocal IOLs were evaluated. A custom-developed laser ray tracing aberrometer was used to measure the optical aberrations. The monochromatic wave aberrations were described using a sixth-order Zernike polynomial expansion. Measurements were obtained under dilated and natural viewing conditions (for accommodative efforts ranging from 0 to 2.5 diopters [D]). The accommodative response was obtained by analyzing changes in paraxial defocus (associated to changes in defocus) and by evaluating the differences in the effective defocus (associated with defocus, spherical aberrations, and pupil diameter) with the accommodative demand. Depth of focus was estimated from through-focus objective optical quality.
Results
Wave aberration measurements were highly reproducible. Vertical trefoil (Z 3 −3 ) was the predominant higher-order aberration in the Crystalens group and significantly higher ( P < .0001) than in the young group, but similar to the monofocal IOL group. The coma root mean square also was higher ( P < .005) in the Crystalens group than in the young group. On average, the defocus term (Z 2 0 ), astigmatism, or higher-order aberrations did not change systematically with accommodative demand in Crystalens eyes. As found for paraxial defocus, the effective defocus in Crystalens eyes did not show significant differences between conditions: 0.34 ± 0.48 D (far), 0.32 ± 0.50 D (intermediate), and 0.34 ± 0.44 D (near). Depth of focus was statistically significantly higher in the Crystalens eyes than in the control groups.
Conclusions
The accommodative response of eyes implanted with the Crystalens accommodative IOLs, measured objectively using laser ray tracing aberrometry, was lower than 0.4 D in all eyes. Several subjects showed changes in astigmatism, spherical aberration, trefoil, and coma with accommodation, which must arise from geometrical and alignment changes in the lens with accommodative demand. Pseudoaccommodation from increased depth of focus may contribute to near vision functionality in Crystalens-implanted patients.
With aging, the crystalline lens first loses its capability to accommodate to near and far objects (presbyopia), and later it loses transparency (cataract). An emerging solution for presbyopia and cataract correction are accommodating intraocular lenses (A-IOLs), artificial lenses that replace the aged crystalline lens of the eye, ideally mimicking the dynamic focusing capability of the young human crystalline lens in response to the ciliary muscle contraction, and that restore both lens transparency and accommodation. Multiple A-IOL designs have been proposed, ranging from Food and Drug Administration-approved A-IOLs to conceptual proposals, and relying on various principles of operation (axial shifts, lateral shifts, or curvature-changing surfaces). To date, the most generalized A-IOL designs rely on an axial shift of the intraocular lens (IOL). The lenses consist of either a single optical element expected to move forward (eg, the Crystalens A-IOL by Bausch & Lomb, Rochester, New York, USA) or 2 optical elements expected to increase their separation axially (e.g., Synchrony by, Abbot Medical Optics (AMO) Inc., Santa Ana, California, USA), in response to an accommodative stimulus.
Most clinical evaluations of performance of A-IOLs primarily are based on the patient’s visual function, that is, testing of near visual acuity, reading tests, through-focus curves, or subjective measurements of accommodation. Although these measurements assess visual performance at different distances, the results provided by these tests generally cannot conclude whether the lenses are actually working according to their functional mechanism. In fact, although several subjective studies report functional near vision with the Crystalens, studies using ultrasound biomicroscopy, partial coherence interferometry, and more recently, comprehensive quantitative 3-dimensional (3-D) optical coherence tomography (OCT) imaging show irrelevant forward shifts of the A-IOL with accommodation. Different studies have shown than the subjective accommodative response after Crystalens A-IOL implantation ranged from 0.44 to 2.36 diopters (D), which was close to the magnitude of standard monofocal IOLs (range, ± 0.85 to ± 1.82 D). Subjective accommodation measurements therefore have proved not very accurate for assessing the accommodative response, because these methods do not differentiate the functional range of near vision attributable to the depth of focus of the eye. Alternatively, dynamic photoretinoscopy, and particularly dynamic autorefraction, have proved to be rapid and repeatable techniques to assess the accommodation response. Langenbucher and associates showed a mean accommodative response of 1.00 ± 0.44 D using photorefraction in patients implanted with the 1CU A-IOL (HumanOptics AG, Erlangen, Germany). Recently, Zamora-Alejo and associates showed no change with accommodative effort in the spherical equivalent in patients implanted with the Crystalens HD.
Besides potential axial displacements of the A-IOL, different factors (pupil size, residual defocus, astigmatism, and higher-order aberrations [HOAs]) may contribute to an expansion of the ocular depth of focus, allowing some near vision functionality. Aberrometry therefore seems to be a highly suitable objective technique to evaluate optical performance of A-IOLs, including potential accommodative responses and the factors that may result in a potential pseudoaccommodation. Static and dynamic aberrometry have been used in the past to assess the change of aberrations with aging or accommodation, as well as the impact of aberrations on the accommodative lag in young subjects. In addition, aberrometry has been used extensively to evaluate optical performance in patients implanted with monofocal IOLs and to estimate the impact of the IOL design on depth of focus. However, whereas optical bench studies and ray-tracing simulations analyzed optical quality in A-IOLs, there are few reports in the literature on the optical aberrations in eyes implanted with A-IOLs. Using dynamic Hartmann-Shack aberrometry, Dick and Kaiser found small changes in defocus in patients implanted with the Crystalens AT-45 (Bausch & Lomb) and 1CU (HumanOptics AG) A-IOLs. Wolffsohn and associates reported some changes in ocular aberrations (defocus, astigmatism, coma, and trefoil) with increased accommodative demand in eyes implanted with the Tetraflex A-IOL (model KH-3500; Lenstec, St. Petersburg, Florida, USA).
Although quantification of the 3-D position of the A-IOL with accommodation has proved to be an ideal tool to assess whether the mechanism of operation of the A-IOL complies with the expected design, aberrometry will be essential to understand the causes of eyes seeming to gain near vision functionality with these A-IOLs. In fact, a future link between geometrical factors and optical outcomes may be established by means of customized eye models. In this study, we measured ocular aberrations for different accommodative demands in 22 eyes implanted with Crystalens AO A-IOL. The absolute amounts of aberrations and their change with accommodation were compared with those of a young control group and those of pseudophakic control group of patients implanted with monofocal IOLs. These measurements allowed evaluation of the objective accommodative response, change of aberrations, and depth of focus in Crystalens A-IOL eyes at different accommodative demands and comparison of the outcomes with the young lens (accommodative response, aberrations, and depth of focus) and nonaccommodating IOLs (aberrations and depth of focus).
Methods
All enrolled patients provided informed consent after they have been informed on the nature and consequences of the study. The protocols were approved by the institutional review boards of the Fundación Jiménez-Díaz, Madrid, Spain, and complied with the tenets of the Declaration of Helsinki.
Patients, Surgery, and Accommodative Intraocular Lenses
Twenty-two eyes from 11 patients were measured (mean age, 75 ± 4 years; range, 67 to 81 years; mean spherical equivalent, −0.5 ± 0.4 D; range, −1.25 to 0.75 D) in this prospective, observational study. Consecutive patients scheduled for cataract surgery with good general health and meeting the inclusion criteria (age older than 50 years, manifest astigmatism less than 1.5 D, and bilateral cataract considered as the sole cause of visual acuity decrease) were invited to participate. The same cohort also followed quantitative 3-D OCT patients for another study.
Patients were implanted with the Crystalens AO A-IOL. This lens has a biconvex single-optic design, with aspheric anterior and posterior surfaces (nominally aiming at 0 IOL aberration, according to the manufacturer). The IOL power (selected using the SRK/T or the Holladay II formula, or both) of the implanted IOLs ranged from 19.50 to 24.50 D.
All procedures were performed by the same surgeon (S.D.) using standard phacoemulsification under local anesthesia The IOLs were implanted using a purpose-designed injector through a clear sutureless corneal incision created in superior–temporal and superior–nasal locations in the right and left eyes, respectively, and enlarged to approximately 2.8 mm. Anterior curvilinear capsulorrhexis (6.5-mm intended diameter) was created manually. All surgeries were uneventful, and all IOLs were implanted successfully in an intracapsular manner. More information on the lens and surgical procedures in this group of patients can be found in an earlier publication.
Control Groups: Young Control Group and Monofocal Intraocular Lens Group
In addition to the patients implanted with A-IOLs, we also measured wave aberrations in young eyes (n = 17; mean age, 28 ± 4 years; range, 21 to 34 years; mean spherical equivalent, −0.2 ± 0.6 D; range, −1.0 to +1.25 D) under natural conditions and similar accommodative demands as those for Crystalens patients. Subjects were recruited specifically for this study among normal volunteers, following a standard ophthalmologic examination, and signed institutional review board-approved informed consent forms before participating in the study.
An additional control group included an aged-matched group of pseudophakic patients implanted with monofocal IOLs (n = 17; mean age, 74 ± 9 years; patients implanted with Tecnis (Abbot Medical Optics (AMO) Inc., Santa Ana, California, USA) and AcrySof (Alcon Research Labs, Fort Worth, Texas, USA) aspheric IOLs). Patients had participated in a previous study, in which ocular aberrations had been measured at 2 different wavelengths to investigate chromatic aberrations using the same aberrometry system. Patients in the monofocal IOL group underwent surgery performed by the same surgeon (S.D.) as the Crystalens patients. The IOLs were implanted using a purpose-designed injector through a clear sutureless corneal incision (2.2 mm) created in superior–temporal and superior–nasal locations in the right and left eyes, respectively. A 6.0-mm continuous curvilinear capsulorrhexis was made under viscoelastic material. A detailed description of the sample and procedures was provided in a prior publication.
Laser Ray Tracing Measurements
In this study, total wave aberrations were measured in Crystalens A-IOL implanted patients 3 months after surgery using a custom-developed laser ray tracing (LRT) optical system for 3 different accommodation stimuli. Measurements were conducted in 2 sessions. In a first session, measurements were performed under natural conditions. In a second session, measurements were obtained under instillation of phenylephrine, which allowed larger pupils without paralyzing the ciliary muscle. The same instrument was used to measure aberrations under natural conditions and 3 different accommodation stimuli in the young eyes and under dilated pupils (tropicamide 1%) and relaxed accommodation in monofocal IOL eyes. Figure 1 summarizes the conditions of the different measurements obtained in each group of patients.
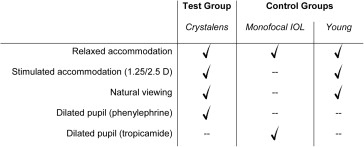
The LRT technique measures the ray aberrations at the retinal plane (incoming aberrometry), and it was first described in detail in previous publications. Illumination was provided by an infrared laser diode at 785 nm (Schäfter + Kirchhoff, Hamburg, Germany). Maximum energy exposure was 6.8 μW. The beam samples the pupil sequentially, and the sampling pattern can be configured by software. A sampling pattern consisting of 37 entry positions arranged in a hexagonal configuration within the pupil was used for this study. The sampling pattern was adjusted by software to fit the pupil of the patient’s eye. In Crystalens eyes, the pupil ranged from 4 to 6 mm after inducing mydriasis (phenylephrine) and from 2 to 4 mm in natural conditions. In young control eyes, the natural pupil ranged from 4 to 6.5 mm (natural conditions), and in the monofocal IOL eyes, the pupil ranged from 4 to 6 mm (tropicamide 1%). The eye’s pupil was monitored during measurements with a charge-coupled device (CCD) camera conjugate to the pupil to ensure correct alignment between the pupil center and the optical axis of the setup, and therefore a line-of-sight measurement reference. A high-sensitivity CCD camera (Retiga 2000-R; QImaging, Surray, British Columbia, Canada) captured the retinal aerial images corresponding to each entry pupil beam. In addition to recording the retinal aerial images, the CCD camera displays the retinal image in real time, allowing users to find objectively the best focus position while assessing the aerial image for a centered ray. During the measurement, the retinal camera is synchronized with the scanner and pupil camera. The acquisition time is approximately 1.5 seconds for an entire typical run. For the purposes of this study (static measurements of aberrations under steady accommodation), an open-field external fixation channel was incorporated in the LRT setup to stimulate accommodation. Figure 2 shows a schematic view of the system, including the accommodation stimulus. The subjects viewed the stimulus monocularly (the contralateral eye was covered with a patch during the measurement). The desired accommodative demand was produced by changing the fixation distance. The far fixation target (4 m) was the middle letter in the last line seen by each patient in an Early Treatment Diabetic Retinopathy chart (typically corresponding to 20/25 visual acuity). The intermediate and near fixation targets were the middle word of the last line read by each patient in Early Treatment Diabetic Retinopathy test intermediate vision (80 cm; equivalent to 1.25 D) and near vision (40 cm; equivalent to 2.5 D) charts, respectively. The size of the stimulus therefore was adjusted to the visual acuity of each patient and condition. Each set of measurements consisted of 5 runs under the same conditions for every fixation target (far, intermediate, and near), and the results presented are the average of 5 repeated measurements.
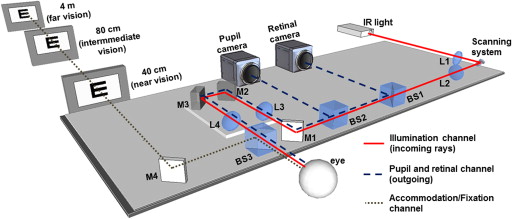
Data Analysis
Wave aberrations were fitted by Zernike polynomials expansions up to the sixth order. The change of defocus (Z 2 0 ), astigmatism (Z 2 2 and Z 2 −2 ), coma (Z 3 1 and Z 3 −1 ), trefoil (Z 3 3 and Z 3 −3 ), and spherical aberration (Z 4 0 ) with accommodative demand were analyzed specifically. Root mean square (RMS) also was used to report the magnitude of HOAs (excluding tilt, defocus, and astigmatism) and of certain characteristic aberrations (astigmatism, coma, and trefoil). When averaging individual Zernike coefficients across eyes, the mirror symmetry terms were flipped in right eyes to account for the enantiomorphism of the right and left eyes.
The accommodative response was obtained as the difference between the accommodative demand and the measured effective defocus. The effective defocus takes into account potential interactions between the second-order Zernike defocus term and the fourth-order Zernike spherical aberration, as well as potential changes in pupil diameter with accommodation, and was defined as:
M = − 4 3 C 2 0 + 12 5 C 4 0 − 24 7 C 6 0 R 2
Previous studies have shown that the spherical error computed using Equation 1 agrees well with that computed from the best focus using retinal plane image quality metrics.
Unless otherwise noted, the analysis was carried out for a 4-mm pupil diameter for all eyes (under dilated pupils) and for the natural pupil diameter in each eye and condition (under natural viewing conditions).
Additionally, the astigmatism (C) and its angle (α) were analyzed from Zernike polynomials expansion by using Equation 2:
J 0 = −2 6 C 2 2 R 2 ; J 45 = −2 6 C 2 − 2 R 2 ; C =−2 J 0 2 + J 45 2 ; α= arctan J 45 J 0 2
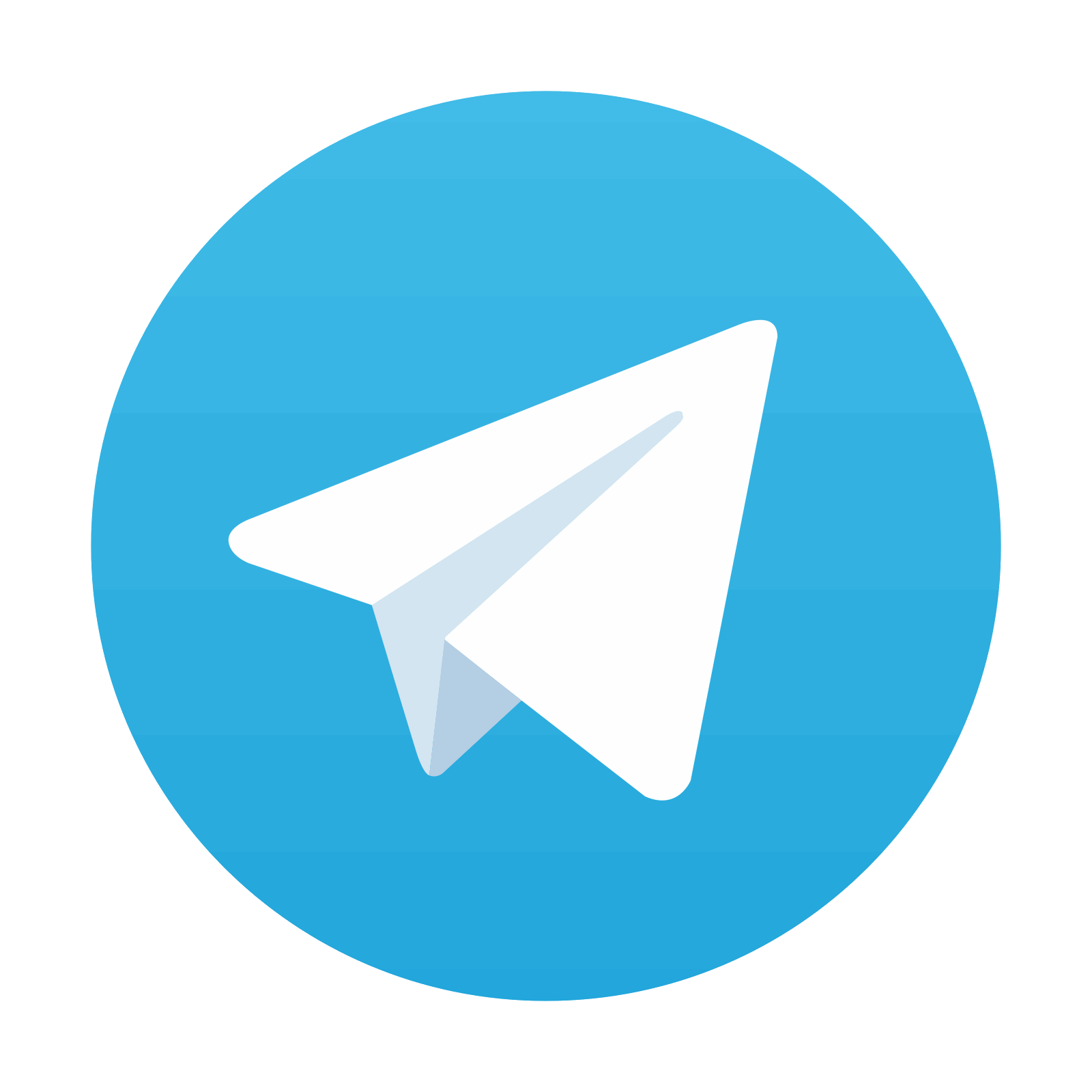
Stay updated, free articles. Join our Telegram channel

Full access? Get Clinical Tree
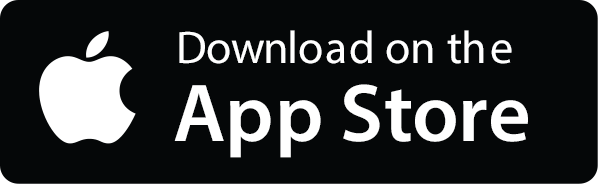
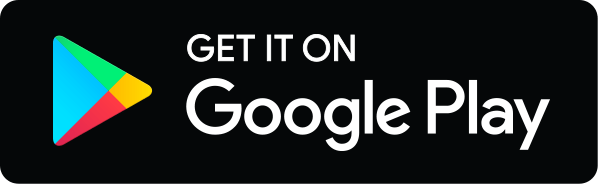