Glaucoma Drainage-Device Surgery
In an attempt to maintain patency of a drainage fistula in glaucoma filtering operations, a wide variety of foreign materials have been implanted in the eye, extending from the anterior chamber to a subconjunctival space. These were once referred to as setons, because the implants consisted of solid structures, such as threads, wires, or hairs, that were placed in a wound to form a drainage, permitting aqueous to run alongside the surface of the inserted material. These procedures were uniformly unsuccessful in maintaining a patent fistula. Most devices use tubes that drain aqueous out of the eye to external reservoirs and have been clinically beneficial. This chapter reviews the most commonly used drainage implant devices, the surgical techniques of implantation, the complications and their management, and the comparative merits and indications for this group of glaucoma surgical procedures.
PHYSIOLOGY OF DRAINAGE IMPLANTS
Most current drainage implant devices (Fig. 39.1) have the same basic design, which typically consists of a silicone tube that extends from the anterior chamber (or, in some cases, the vitreous cavity) to a plate, disc, or encircling element beneath conjunctiva and Tenon capsule. The edge of the external plate has a ridge, through which the distal end of the tube inserts onto the upper surface of the plate. The ridge decreases the risk for obstruction of the posterior opening of the tube with the surrounding tissue and fibrous capsule. The plates of the glaucoma drainage devices have large surface areas and promote the formation of the filtering bleb posteriorly, near the equator.
Figure 39.1 Examples of glaucoma drainage devices. A: Ahmed FP-7 (silicone).B: Ahmed S2 (polypropylene; image shown at left), S3 pediatric (polypropylene; middle), and B1 (polypropylene—double plate; right).C: Single-plate Molteno device.D: Baerveldt 250 mm2(left) and 350 mm2(right).
The mechanism by which drainage implant devices control the intraocular pressure (IOP) relates to a fibrous capsule that forms a filtering bleb around the external portion of the draining device and, to some degree, the surface area of the implant plate. The morphology of this filtering bleb differs from that of the blebs seen after trabeculectomy.
After insertion of the drainage device, a thin collagenous capsule, surrounded by a granulomatous reaction, is present at 1 month. The granulomatous reaction resolves after 4 months, capsule thickness remains relatively stable, and the collagen stroma becomes less compact. The fibrous capsule matures over time and becomes thinner after 6 months in rabbit eyes (1). Although the bleb histology in the rabbit model is similar to that of humans and other primates, the eventual development of a fibroblastic inner lining in the rabbit model differs from that in humans, in whom the inner lining remains only as a meshwork of collagen-like bundles at some areas of the inner bleb wall (1,2). Even though the filtering bleb around the implant is lined with a thick layer of connective tissue, microcystic spaces within that layer, seen on light and electron microscopy, may serve as the channels for aqueous drainage (2). Studies of monkey eyes with single-plate Molteno implants indicate that the capsule functions by a passive mechanism, shunting the flow of aqueous humor to the surrounding orbital tissues (3). All surfaces of the fibrous capsule contribute to filtration, which is consistent with echographic studies in human eyes that reveal bleb formation on both sides of the plate in successful cases (4). Histopathologic study of human eyes enucleated 2 to 6 years after Molteno implant surgery revealed patent tubes with no appreciable anterior chamber reaction and minimal inflammatory reaction in the outer layers of the bleb wall (5).
Measurement of the flow resistance using modified Baerveldt plates in rabbits showed a direct relationship between the surface area of the implants and the filtering capacity of their surrounding capsule (6). At the same time, reduction of the bleb diameter decreases surface tension on the bleb, capsular fibrosis, and thickness, which increases the effectiveness of the filtering surface (7,8).
Drainage devices with open tubes are likely to be complicated by early postoperative hypotony and therefore require temporary closure with a ligature or stent. The vast majority of glaucoma drainage devices develop an elevated IOP in the weeks to months after implantation as a result of capsule formation around the implant plate. This is frequently termed the hypertensive phase (9,10).
The filtering bleb may fail after surgery due to the increased thickness of fibrous capsule around the drainage implant. Movement of the drainage plate against the scleral surface may be the mechanism of glaucoma implant failure resulting from the stimulation of the low-level wound healing response, increased collagen scar formation, and increased fibrous capsule thickness (11).
IMPLANT DESIGNS
Performance of similar drainage devices can vary significantly, depending on the standards in manufacturing. This causes a wide range of clinical outcomes and indicates a strong need for enhanced quality-control procedures in the device-manufacturing process (12). The glaucoma drainage devices also differ according to the size, shape, and materials from which the external component and tube are constructed. External portions of glaucoma drainage devices are made from materials that prevent fibroblast adherence. Different materials may influence the amount of inflammation in surrounding tissues. Polypropylene, used in some Ahmed and Molteno implants, may produce more inflammation than the silicone used in Baerveldt, Krupin, and Ahmed devices. Flexible plates caused less inflammation in the subconjunctival space of rabbit eyes than in the rigid ones (13,14).
Alternative materials, such as hydroxylapatite (15) and expanded polytetrafluoroethylene (16,17), that increase vascularization of the fibrous capsule around the plate, may offer a theoretical advantage by enhancing the efficacy, decreasing the capsule size, and increasing the functional lifetime of the implant (15).
One of the most fundamental design differences, however, is whether the device has an open, unobstructed drainage tube or one that contains a pressure-regulating valve. Baerveldt, Molteno, and Schocket implants are examples of open-tube implants. Ahmed and Krupin implants are designed to have a flow-restricting valve mechanism.
Open-Tube Drainage Devices
Baerveldt Implant
The unique feature of this series of popular nonvalved drainage implants is the large surface area of the plates, which are designed in such a way that they can be easily implanted through a one-quadrant conjunctival incision. A silicone tube is attached to a soft barium-impregnated silicone plate with a surface area of 250 mm2 (20 mm × 13 mm) or 350 mm2 (32 mm × 14 mm) (18). In an 18-month prospective study, the 350-mm2 implant had a similar rate of success but lower risk for complications than did the 500-mm2 model (19), which is no longer available.
The plate is typically positioned under the rectus muscle insertions, typically in the superotemporal quadrant (Fig. 39.2). The Baerveldt plate has fenestrations that allow growth of fibrous tissue through the plate, serving to reduce the height of the bleb, which reduces the risk for diplopia and helps secure the implant (19). A fibrous capsule forms after the first 3 to 6 postoperative weeks into which fluid can drain and from which fluid can be absorbed by the surrounding tissues.
Figure 39.2 Baerveldt implant is positioned under the superior and temporal rectus muscles.
After a previously failed trabeculectomy or cataract surgery, Baerveldt drainage devices were found to be more likely to control IOP, compared with trabeculectomy, but at the cost of greater ocular motility disturbances after 1 year of follow-up in a randomized, controlled trial (20,21). In retrospective case–control or consecutive case series, a 350-mm2 Baerveldt implant had lowered IOP by a similar amount as a double-plate Molteno implant or Ahmed implant (discussed later) in patients with uncontrolled, complicated glaucoma (22–24). In a retrospective study, the 350-mm2 implant maintained IOP below 21 mm Hg in 87% of the eyes, compared with 70% with the 500-mm2 implant after 3 years (25). The success rate declined to 79% in the 350-mm2 group and to 66% in the 500-mm2 group after 5 years. The rate of complications was similar between the two groups, but complications occurred slightly more often in the 500-mm2 group (25). Retrospective studies are limited by selection bias and may not detect small or mild differences.
Molteno Implant
This is the prototype drainage implant device and has had the longest and most extensive clinical experience since Molteno introduced it in 1969 (26). The original design consists of a single plate of thin acrylic with a diameter of 13 mm and an area of 135 mm2. A silicone tube with an external diameter of 0.62 mm and an internal diameter of 0.30 mm connects to the upper surface of the plate. The plate has a thickened rim, which is perforated to allow suturing to the sclera.
Subsequent modifications addressed various problems encountered with the original design. Success rates with single-plate Molteno implantation for glaucomas with poor surgical prognoses (aphakic or pseudophakic eyes, prior failed filters, neovascular glaucoma, and patient age younger than 3 years) ranged from 25% to 46% in one study but rose to 40% to 71% with implantation of a second plate (27). A double-plate Molteno implant combines two plates, one of which is attached to the silicone tube in the anterior chamber, whereas a second tube connects the two plates, giving an increased surface area of 270 mm2 (28). In a randomized trial comparing single-plate and double-plate implants, the latter provided better IOP control but was associated with a greater risk for complications, most of which were related to hypotony (29). Another modification, which addresses the problem of hypotony, is the dual-chamber, single-plate implant, in which a V-shaped “pressure ridge” on the upper surface of the plate encases an area of 10.5 mm2 around the opening of the silicone tube (30). In concept, the pressure ridge and overlying Tenon capsule regulate the flow of aqueous into the main bleb cavity during the early postoperative period, thereby minimizing excessive filtration and hypotony. The validity of this concept was supported in one study of 40 consecutive patients (31), but the ridge effect was found to be unpredictable in a more recent study (32). A third-generation implant, named Molteno 3, has a bowl-shaped structure on the implant plate immediately at the opening tube. It is designed to function as a biologic valve by limiting the available area of filtration during times of low aqueous production. To date, no data on the effectiveness of the Molteno 3, which is available with plate sizes of 175 and 230 mm2, have been published.
Schocket Tube Shunt
Schocket and associates (33,34) developed a technique in which a silicone, or silastic, tube is extended from the anterior chamber to a 360-degree encircling silicone band, as used in retinal detachment repair (Fig. 39.3), which functioned in developing the reservoir for aqueous drainage. Modifications have included insertion of the tube into a band extending for only 90 degrees beneath two rectus muscles or into the preexisting encircling band in eyes with glaucoma after scleral buckling surgery (35,36). A long Krupin–Denver valve implant (discussed later under “Krupin Implants”) has also been used in combination with a 180-degree scleral band (37).
Figure 39.3 Schocket glaucoma drainage device.
Two randomized trials compared Schocket tube shunts with double-plate Molteno implants. Although the Schocket shunt typically provides a larger surface area of the reservoir than the Molteno implants do, the latter provided lower final IOP in both studies (38,39).
Flow-Restricted Drainage Devices
Little resistance is offered to aqueous outflow until the plate becomes encapsulated. The incorporation of a valve mechanism in implants seems to decrease early postoperative hypotony by providing resistance to the flow and therefore regulating the pressure within a desired range.
Ahmed Glaucoma Valve
The Ahmed glaucoma valve implant is one of the most commonly used flow-restricted implants in difficult glaucomas. In this valved drainage implant design, a silicone tube is connected to a silicone sheet valve, which is held in a polypropylene body (40) (Fig. 39.4). The body of the S2 and FP-7 models has a surface area of 184 mm2 (16 mm × 13 mm) and is 1.9 mm thick; the reservoir plate of the S2 model is made from polymethylmethacrylate, whereas that of the FP-7 model is made from silicone. A small retrospective comparison suggested that the FP-7 model may lower IOP at 1 year more than the S2 model does (41). The valve mechanism consists of two thin silicone elastomer membranes, 8 mm long and 7 mm wide, which allows one-way regulation of the flow with a goal of keeping the IOP between 8 and 10 mm Hg in the early postoperative period. A second plate can be connected to the reservoir plate and implanted in a second quadrant to increase the surface area by 180 mm2. These plates are made from both silicone and polypropylene, models FX1 and B1, respectively, to connect to corresponding valved plate FP-7 or S2. Furthermore, a smaller valved implant of 96 mm2 is available and made from silicone (FP-8) or polypropylene (S3).
Figure 39.4 Ahmed glaucoma drainage device.
The inlet cross section of the chamber is wider than the outlet, which offers a theoretical small pressure differential between the anterior chamber and subconjunctival space, which is claimed to enable the valve to remain open even when only a small difference in pressure exists. However, no definitive proof of this has been given, and application of the Bernoulli equation (flow rate of a fluid is inversely proportional to pressure of the fluid) to the parameters that exist within the physiologic IOP range shows that the Bernoulli effect is almost nonexistent in either the Ahmed glaucoma valve chamber or the Krupin eye valve (discussed later). Calculations indicate that there is no significant pressure drop across the “valves” and that the critical site for pressure drop is at the capsule surrounding the glaucoma implants (42).
One study evaluated obstruction to aqueous flow with the Ahmed implant. The obstructions were separated into tube-related and capsule- or valve-related obstructions. The Ahmed implant, as with other implants, has a hypertensive phase, which is a transient phase of low capsule permeability seen at 4 to 8 weeks postoperatively. The authors also introduced the concept of the “no-touch zone” on the Ahmed glaucoma valve, which is the area of the implant covering the chamber with the silicone leaflets. If the implant is grasped with forceps along the center line, it may separate the valve cover from the implant. The external pressure on the valve chamber can cause a defect in closure of the valve with consequent early postoperative hypotony and fibrovascular membrane ingrowth between the leaflets (43). This may lead to a failure of the valve due to adhesion of the valve membranes (44).
In a retrospective review, the double-plate Molteno implant with mitomycin C was more likely than the Ahmed drainage device with mitomycin C to result in an IOP lower than 15 mm Hg (45). Success rates at 1 year were 80% for the Molteno implant, 39% for the Krupin eye valve with disc, and 35% for the Ahmed drainage device. However, the Ahmed device was less likely to cause complications requiring another surgery (45).
Krupin Implants
In 1976, Krupin and associates introduced the concept of a one-way valve that opens at a predetermined IOP level to avoid the early postoperative complications of excessive drainage and hypotony (46). The original Krupin–Denver valve was composed of an internal Supramid tube cemented to an external silastic tube (46). The valve effect was created by making slits in the closed external end of the silastic tube, designed to open at an IOP between 9 and 11 mm Hg. The tube was short, extending only a few millimeters subconjunctivally, and had no external plate. Although preliminary experience was encouraging (47,48), fibrosis eventually closed the subconjunctival portion of the valved tube (49), which led to failure in most cases.
In a subsequent technique, a long Krupin–Denver drainage tube, with the same one-way valve design, was attached to a 180-degree Schocket-type scleral explant, as previously described (37). This led to development of the Krupin eye valve with disc, which is the design in current use. A silastic tube is attached to an oval silastic disc, conformed to the curvature of the globe, 13 mm × 18 mm, with side walls that are 1.75 mm high (50). The valve at the distal end of the tube is the same design as in the earlier Krupin implants and is manometrically calibrated to open at pressures between 10 and 12 mm Hg. In the newer design of the Krupin implant, the valve lies inside the rim of the plate at its insertion and, as such, is exposed directly to the subconjunctival tissues (51). A review of 113 patients with the Krupin eye valve with disc implants identified 8 patients with primary valve malfunction requiring surgical revision, which involved manipulation, replacement of the valve, and amputation of the valve. Transient postoperative hypotony was noted in three patients, and chronic hypotony with loss of light perception in one patient. One explanted valve was examined and found to have partially fused leaflets, possibly related to the sterilization process and prolonged storage before implantation (51).
Other Drainage Devices
The Ex-PRESS glaucoma drainage device is a more recently introduced implant, but it differs significantly from the aforementioned devices. The other implants have the basic design of a silicone tube that connects an intraocular space, most commonly the anterior chamber, with a subconjunctivally located reservoir plate, whereas this reservoir plate allows for the development of a delimited potential space and the formation of a fibrous capsule to create the resistance to outflow. The Ex-PRESS device does not have a reservoir plate, is implanted under a traditional trabeculectomy flap, and is subject to all of the considerations of a trabeculectomy (see Chapter 38).
At the time of publication, two other drainage devices are under investigation for approval in the United States. The Solx Gold Shunt (Solx Inc., Waltham, MA) is made from 24-karat gold and works to connect the anterior chamber and suprachoroidal space. Although the device is implanted by using an ab externo approach, no subconjunctival drainage occurs (i.e., no bleb). Effectiveness data have yet to be published. The iStent trabecular microbypass stent (Glaukos Corporation, Laguna Hills, CA) is a stainless-steel stent with a lumen that is implanted from an ab interno approach. The device traverses the trabecular meshwork and drains aqueous from the anterior chamber into the Schlemm canal, enhancing aqueous drainage (52). According to early studies, the iStent appears to lower IOP in chronic open-angle glaucoma as a stand-alone procedure and when used in conjunction with cataract extraction (53,54).
IN VITRO COMPARISON OF DEVICES
The Krupin, Baerveldt, Ahmed, and OptiMed implants were compared at physiologic flow rates in vitro and in vivo in rabbits (55). With all devices, opening pressures were higher in vivo than in vitro because of tissue-induced resistance around the explant. Pressures with all devices dropped to 0 mm Hg after conjunctival wound disruption. In air, the Krupin and Ahmed implants had opening pressures of 7.2 and 9.2 mm Hg and closing pressures of 3.9 and 5.2 mm Hg, respectively. The OptiMed implant had the highest resistance values, with IOPs of 19.6 mm Hg, compared with 7.5 mm Hg with the Ahmed implant in vivo. The resistance was similar for the Baerveldt, Krupin, and Molteno dual-chamber devices implanted in vivo. Both Ahmed and Krupin valves functioned as flow-restricting devices, rather than true valves at the flow rates studied, but did not close after initial perfusion with fluid. Neither the Ahmed nor Krupin device had demonstrable opening or closing pressures in balanced salt solution. In another comparative study, the Joseph implants provided slightly lower IOPs and had significantly fewer failures than did the Schocket devices, although the Molteno implants provided the lowest pressures at 12 months among eyes with successful IOP control (56).
Resistance and pressure responses of the OptiMed, Krupin, and Ahmed drainage devices were compared by using a 30-gauge cannula as a simple resistor to determine whether the devices function as true valves. Resistance remained relatively constant for the Krupin and OptiMed implants, whereas the Ahmed offered a variable resistance over a range of flow rates and pressures between 12 and 15 mm Hg. The Ahmed device functioned as a valve that closely regulated pressure within a desired range by decreasing or increasing resistance as a function of flow (57).
SURGICAL TECHNIQUES
Basic Principles
Although certain variations of surgical techniques are required for implantation of the different implant designs, the basic surgical principles apply in general to all glaucoma drainage devices.
Adequate surgical exposure is dependent on proper placement of a traction suture. A 6-0 polyglactin (Vicryl) or silk traction suture on a spatulated needle is placed through superficial cornea near the superior limbus and attached to the drape beneath the eye.
A fornix-based conjunctival–Tenon capsule flap is created, usually in the superotemporal quadrant, to expose the scleral bed (Fig. 39.5A). The flap is slightly elevated to allow for blunt dissection between Tenon and episclera with blunt Westcott scissors. Radial relaxing incisions on one or both sides of the conjunctival flap can improve surgical exposure. A muscle hook is then used to isolate the two rectus muscles on either side of the surgical site.
Figure 39.5 A: Creation of subconjunctival space in the superotemporal quadrant.B: Insertion of plate into subconjunctival space. C: Cutting the tube to the appropriate length.
Whenever possible, the superonasal quadrant should be avoided, especially with the larger plate designs, to reduce the risk for strabismus (discussed later) (58). The Ahmed drainage device, when placed in the superonasal quadrant, has also been shown to come within 1 mm of the optic nerve (59).
With Ahmed valved implants, balanced salt solution must be irrigated through the tube using 27-gauge cannula, before the insertion into the anterior chamber, to ensure that the valve opens properly.
The external plate is then tucked posteriorly into the sub-Tenon space (Fig. 39.5B) and is sutured to sclera with nonabsorbable 9-0 Prolene or nylon sutures through the anterior positional holes of the plate, with the anterior border at 8 to 10 mm posterior to the limbus. Variations of this technique are required for different plate designs. Implants with plates of larger circumferential dimensions, such as the Baerveldt, should be tucked under adjacent rectus muscles, whereas Schocket-type designs require dissection of one or more additional quadrants, depending on the extent of the encircling band. In the case of the Ahmed device, which has larger anteroposterior dimensions, extending the anterior border of the plate more than 8 mm behind the limbus is not advisable. However, it is advisable to have the reservoir plates at least 5 to 6 mm posterior to the limbus to prevent conjunctival erosion over the plate (Fig. 39.6).
Figure 39.6 Exposed plate of an Ahmed glaucoma drainage device that was placed too close to the limbus.
With nonvalved devices, restriction of aqueous flow to avoid severe early postoperative hypotony can be achieved by using a two-stage implantation technique, in which the external plate is placed in the subconjunctival space without inserting the tube into the anterior chamber. The tube is inserted 6 to 8 weeks later, after the fibrous capsule has formed around the external plate (60–62). A more popular technique is to occlude the tube by a ligature of 6-0, 7-0, or 8-0 Vicryl before inserting it into the anterior chamber. Injection of a balanced salt solution with a 30-gauge cannula into the tube helps to confirm that the tube is totally occluded. This procedure prevents any drainage of aqueous until 4 to 6 weeks after the operation when the Vicryl suture dissolves, allowing aqueous to drain into the preformed capsule. This technique provides the advantage over the two-stage technique of avoiding a second operation (63). Various tube ligatures and stents have been used to minimize postoperative hypotony (as discussed later under “Complications: Prevention and Management”).
The tube is then cut, bevel up, to permit its extension 2 to 3 mm into the anterior chamber (Fig. 39.5C). Before the tube is inserted into the anterior chamber, a limbal area is cauterized to prevent bleeding from the insertion. A paracentesis can be made inferotemporally to allow placement of a small amount of viscoelastic in the anterior chamber. It is best to maintain the anterior chamber at a normal depth and avoid displacing iris posteriorly in order to assess the true position of the implant tube in the anterior chamber.
The anterior chamber is then entered through the cauterized limbal area with a 23-gauge or a 22-gauge needle, parallel to the iris plane (Fig. 39.7). The needle creates a watertight seal, preventing leakage around the tube and thus reducing the risk for postoperative hypotony (64). The angle at which the needle enters the anterior chamber is critical, because it is important that the tube, which will pass through this needle track, is positioned between cornea and iris, without touching the cornea.
Figure 39.7 Entering the anterior chamber through the limbal area with a 23-gauge or a 22-gauge needle parallel to the iris plane.
The tube is then inserted into the anterior chamber via the needle track; there are specially designed tube-insertion forceps, but these generally are not necessary (Fig. 39.8). The tube can be secured to the sclera by using a nonabsorbable suture, such as 9-0 Prolene or nylon, but this step is also optional. Contact of the tube with the iris does not seem to cause any clinically noticeable problems, although tube occlusion by the iris and a distortion of the pupil have been reported (65,66). The anterior chamber may need to be deepened with balanced salt solution, or viscoelastic, via the paracentesis, and the tube is checked for proper position in the anterior chamber.
Figure 39.8 Tube insertion into the anterior chamber via the needle track, using nontoothed or specially designed tubeinsertion forceps.
The tube may occasionally erode through both sclera and overlying conjunctiva at the limbus. To avoid this potential complication, most surgeons suture a rectangle of preserved donor tissue of approximately 5 mm × 7 mm over the tube at the limbus (67) (Fig. 39.9). Processed pericardium (Tutoplast), donor sclera, dura, and fascia lata are available commercially for this purpose. It is also possible to use autologous sclera or to place the tube under a partial-thickness scleral flap, similar to a trabeculectomy procedure.
Figure 39.9 Suturing of donor sclera or other patch material over the tube area at the limbus.
The conjunctiva is then sutured back to its original position using Vicryl sutures. Subconjunctival steroids and antibiotics are injected at the completion of the procedure in a quadrant away from the surgical site. The basic postoperative management is the same as that described in Chapter 38 for filtering surgery, using topical steroid–antibiotic and mydriatic–cycloplegic preparations for the first several weeks.
Modifications of Basic Technique
Sometimes the conjunctiva is scarred at the limbus, making conjunctival dissection impossible without destroying much of the conjunctival tissue. In this case, the initial conjunctival incision may be made approximately 8 mm from the limbus to create a limbal-based conjunctival flap. Another option is to use the inferotemporal or a superonasal quadrant.
Various occlusion ligatures include a posteriorly placed suture, a releasable suture, and an anterior chamber tube ligature. A 5-0 nylon suture can be threaded into the tube at the plate end and secured with one or two absorbable sutures around the tube (68,69). The exposed end of the nylon suture is positioned subconjunctivally near the limbus for subsequent removal. Biodegradable stents, such as collagen lacrimal plugs or 4-0 chromic suture, have also been evaluated, but they have been less satisfactory because they do not always dissolve (70,71). The internal and external occlusion techniques may be combined. For example, a 5-0 nylon or 3-0 Supramid internal occlusion suture is placed, along with an external Vicryl ligature around the tube. The internal stent is then pulled without difficulty in the office treatment room (68).
Using a clear corneal graft, tied with 8-0 nylon, instead of the pericardium or scleral graft to cover the outer portion of the tube provides the view of the tube with the suture for postoperative laser suture lysis (72).
As an alternative to the use of a preserved tissue, an autologous, partial-thickness scleral patch graft crafted from the sclera adjacent to the tube has been described. No complications were reported in the study, but the risk for perforation of the globe exists during the dissection of the flap, and this may not be a good choice in the eyes with high myopia or with scleritis (73).
Some surgeons combine stent occlusion (Fig. 39.10) with longitudinal slits in the tube to provide early IOP control (74), and a laboratory study indicated that a slit valve of 2.0 mm appears to provide an opening pressure of approximately 10 mm Hg (75). Using the 350-mm2 Baerveldt implant, the role of fenestrations in the tube and antimetabolites was studied in controlling IOP in the early postoperative period. An occlusive 7-0 Vicryl suture was placed just anterior to the plate, followed by a through-and-through penetration of the tube with a standard 15-degree blade in longitudinal orientation just anterior to the ligature. The IOP was elevated at day 21 because of fibrotic blockage of the fenestrations before the ligature dissolved, but the pressure was well controlled by antiglaucoma medication use or laser suture lysis of the 7-0 Vicryl occlusion suture. The use of antimetabolites did not improve the outcome (76).
Figure 39.10 Example of a Baerveldt 350-mm2 implant in which a 5-0 nylon stent suture is placed and a 7-0 polyglactin suture is tied around the silicone tube, constricting it occlusively around the stent suture. The needle of the 5-0 nylon suture allows for easy externalization and placement under the bulbar conjunctiva.
Another potential complication, as with all filtration procedures, is failure due to excessive fibrosis. In a rabbit study that compared intraoperative use of mitomycin C, 0.5 mg/mL for 5 minutes, with implantation of 200-mm2 Baerveldt implants, the mitomycin C–treated eyes had lower IOPs and higher perfusion rates at 2, 4, and 6 weeks (77). In one retrospective study, implantation of Ahmed glaucoma drainage devices combined with the use of mitomycin C achieved lower postoperative IOP, with fewer glaucoma medications and similar complication rates compared with Ahmed devices implanted without antimetabolites (78). However, three retrospective studies of Baerveldt and Molteno implants and a randomized trial with Baerveldt implants did not show any benefit to using intraoperative mitomycin C (76,79–81). At the present time, the preponderance of evidence indicates that use of antimetabolites in conjunction with glaucoma drainage-device surgery incurs little benefit.
Special Situations
Pars Plana Insertion
In aphakic (or possibly pseudophakic) eyes in which a vitrectomy has been performed, the tube can be inserted through a pars plana incision into the vitreous cavity. The pars plana tube shunts are usually used when placing the tube into anterior chamber is impossible or undesirable or when a need for pars plana vitrectomy coexists. A Hoffman elbow has been designed for pars plana insertion, and excellent results were demonstrated with the Baerveldt implant following pars plana vitrectomy and fluid–gas exchange. A mean postoperative IOP of 14 mm Hg with an average of 0.6 glaucoma medications was reported in one study (82). The pars plana insertion has the advantage of keeping the tube away from the cornea (especially after penetrating keratoplasty [PKP]) and iris and of reducing the risk for epithelial downgrowth. It is especially important in eyes with corneal grafts. Repositioning of the glaucoma drainage device from the anterior chamber into the vitreous cavity after pars plana vitrectomy for anterior segment complications, such as corneal decompensation, or recurrent tube erosion, is another option (83).
Preexisting Scleral Buckle
The treatment of a retinal detachment may be associated with postoperative glaucoma. The presence of the scleral buckle presents a special challenge in cases in which IOP cannot be controlled medically. Conjunctival scarring caused by retinal surgery can significantly decrease the success of trabeculectomy, even with the use of antimetabolites. Cyclodestructive procedures can be used, but they are unpredictable and may cause significant complications. Glaucoma drainage devices are a useful option to control IOP in such eyes, although the presence of scleral buckle makes placement of the plate challenging.
When a scleral buckle has been placed in the eye for more than 3 months, a silicone tube can be inserted into the anterior chamber, with the distal end introduced into the fibrous capsule of the preexisting scleral buckle, which serves as an external reservoir for aqueous drainage. Because the buckle is already encapsulated, no ligation of the tube to restrict the flow is necessary. In one study, the IOP was successfully controlled in 85% of patients (36).
Long Krupin–Denver valved implants with a flow restrictor at the distal end of the tube can further decrease the chances of postoperative hypotony in eyes with scleral buckle. If the scleral buckle was placed more recently than 3 months and the fibrous capsule has not formed yet, a smaller Baerveldt implant can be used, and the “wings” of the device sometimes need to be trimmed to position the plate underneath the existing scleral band. The fibrous capsule then is expected to grow around the buckle and the Baerveldt implant (84).
Successful insertion of a Baerveldt drainage device behind or over a preexisting scleral buckle, or in the segment without retinal hardware, has been described. Excising the capsule overlying the band allows continuous encapsulation of the band and Baerveldt plate to achieve greater IOP reduction. After 1 year, IOP control was achieved without medications in 78% of patients with 350-mm2 plates, but in only 29% of patients with 250-mm2 plates (85).
Preexisting Corneal Graft
Glaucoma after PKP remains a difficult management issue. PKP often causes additional damage to the angle, inducing peripheral anterior synechiae formation, with further impediment to aqueous outflow. Control of post-PKP glaucoma is complicated by the need to preserve graft clarity for visual function. When medical management fails, if the angle is open and grossly normal, argon laser trabeculoplasty may be an option. If further intervention is indicated, a glaucoma drainage device, in eyes with good visual potential, is recommended. For eyes with poor visual potential (or for patients who cannot undergo surgery), transscleral cyclophotocoagulation may be a better option (86).
However, placement of glaucoma drainage devices in the anterior chamber may be complicated by tube–cornea touch and endothelial decompensation, particularly after corneal transplantation. Only 70% and 55% of the corneal grafts survived at 2 and 3 years, respectively, after insertion of glaucoma drainage devices into the anterior chamber (87). A retrospective review of simultaneous PKP and Ahmed glaucoma drainage-device implantation showed 92% and 50% graft success and 92% and 86% IOP control at 1 and 3 years, respectively (88).
Pars plana insertion is a reasonable option for patients who have undergone PKP or in whom PKP is anticipated, despite the need for a complete pars plana vitrectomy. The pars plana approach avoids complications related to limbal tube placement and offers better corneal graft survival, but the incidence of posterior segment complications may be higher for pars plana insertion (89).
Implantation of the tube through the ciliary sulcus is another alternative to anterior chamber angle placement in pseudophakic or aphakic eyes with refractory glaucoma and a high risk for corneal decompensation, or eyes with a shallow anterior chamber or extensive synechial angle closure. Positioning of the tube under the iris may be particularly advantageous in the presence of an anterior chamber intraocular lens, because the tube would not disturb the lens. This procedure is contraindicated in phakic eyes because of a possible injury to the crystalline lens (90).
COMPLICATIONS: PREVENTION AND MANAGEMENT
Hypotony
Until the fibrous capsule has developed around the external plate to regulate aqueous flow, the open, nonvalved drainage devices, as noted earlier, provide very low resistance to flow, and hypotony in the early postoperative course with nonvalved implants is a serious complication. By far the best way to prevent this potential complication is by temporarily obstructing the tube lumen. Many techniques have been described to achieve this goal. Basic techniques include suture ligation of the tube, as previously described; temporary occlusion of the tube lumen with a stent; two-stage implantation; or use of a valved implant. Early postoperative hypotony was found in fewer than 10% of patients after Ahmed glaucoma drainage-device surgery (9,91). If early postoperative hypotony happens in combination with a flat anterior chamber, then injection of dense viscoelastic into the anterior chamber and close observation in the first 24 hours may be helpful. If the flat chamber and hypotony reoccur, then removal of the tube from the anterior chamber is recommended to prevent corneal decompensation with planning to reposition the tube into the anterior chamber within the next few days.
Late hypotony from glaucoma drainage-device implantation is usually treated with permanent occlusion of the proximal tube or removal of the tube from the anterior chamber, which permanently removes the effect of the entire implant. Permanent ligation of the tube to the distal plate of double-plate Molteno implant has the advantage of reducing, but not completely eliminating, the effect of the implant (92).
Elevated Intraocular Pressure
Glaucoma drainage-device procedures can also be complicated by elevated IOP in the early or the late postoperative period. Before the ligature around the tube dissolves, there may be a transient elevation of the IOP. It can be prevented by combining a trabeculectomy without mitomycin C with the drainage device, or it can be managed medically. Within the first 7 to 10 days after surgery, a hypotensive phase may present with low IOP, conjunctival and corneal edema, and congestion of conjunctival blood vessels in tissues covering the plate of the implant. This may be followed by a second, hypertensive phase, which is characterized by IOP elevation associated with the formation of the capsule. In this phase, the edema disappears and fibrous tissue develops in the deepest layers of the bleb. During the first 1 to 4 weeks of this phase, the bleb wall becomes congested, causing the IOP elevation. Congestion and inflammation subsequently subside, with IOP reduction and stabilization over the next 3 to 6 months. The hypertensive phase portends a poor prognosis for IOP control (9,93).
Elevated IOP in the early postoperative period may be due to obstruction of the tube by fibrin, blood, iris, vitreous membranes, or silicone oil (Fig. 39.11). This was observed in 11% of eyes after implantation of an Ahmed glaucoma drainage device (91), with iris and fibrinous membranes being the most common tissues responsible for blockage (30.8% each), followed by a neovascular membrane, a fibrinous strand, and an iridocorneal endothelial membrane. Iridectomy at the site of the tube ostium has been recommended to prevent iris plugging the tube ostium (94) but requires a larger incision. Nd:YAG laser membranectomy was effective for reopening blocked glaucoma tube shunts and maintaining the patency over time in 84.6% of the eyes in one retrospective study, but recurrence of the blockage occurred in 53.8% of eyes within the first 11 weeks. Postlaser complications included moderate anterior chamber reaction, hyphema, corneal edema, pressure spike, and a shallow anterior chamber (95). Distal tube occlusion by fibrous tissue has been reported after placement of glaucoma drainage devices in the fibrous capsule around a preexisting scleral buckle (36,56,96).
Figure 39.11 Occlusion of a glaucoma drainage device by fibrin in a patient with neovascular glaucoma. (From Junk AK, Katz LJ. Tube shunts for refractory glaucomas. In: Tasman W, Jaeger EA, eds. Duane’s Clinical Ophthalmology. Vol 6. Lippincott Williams & Wilkins; 2007:chap 17.)
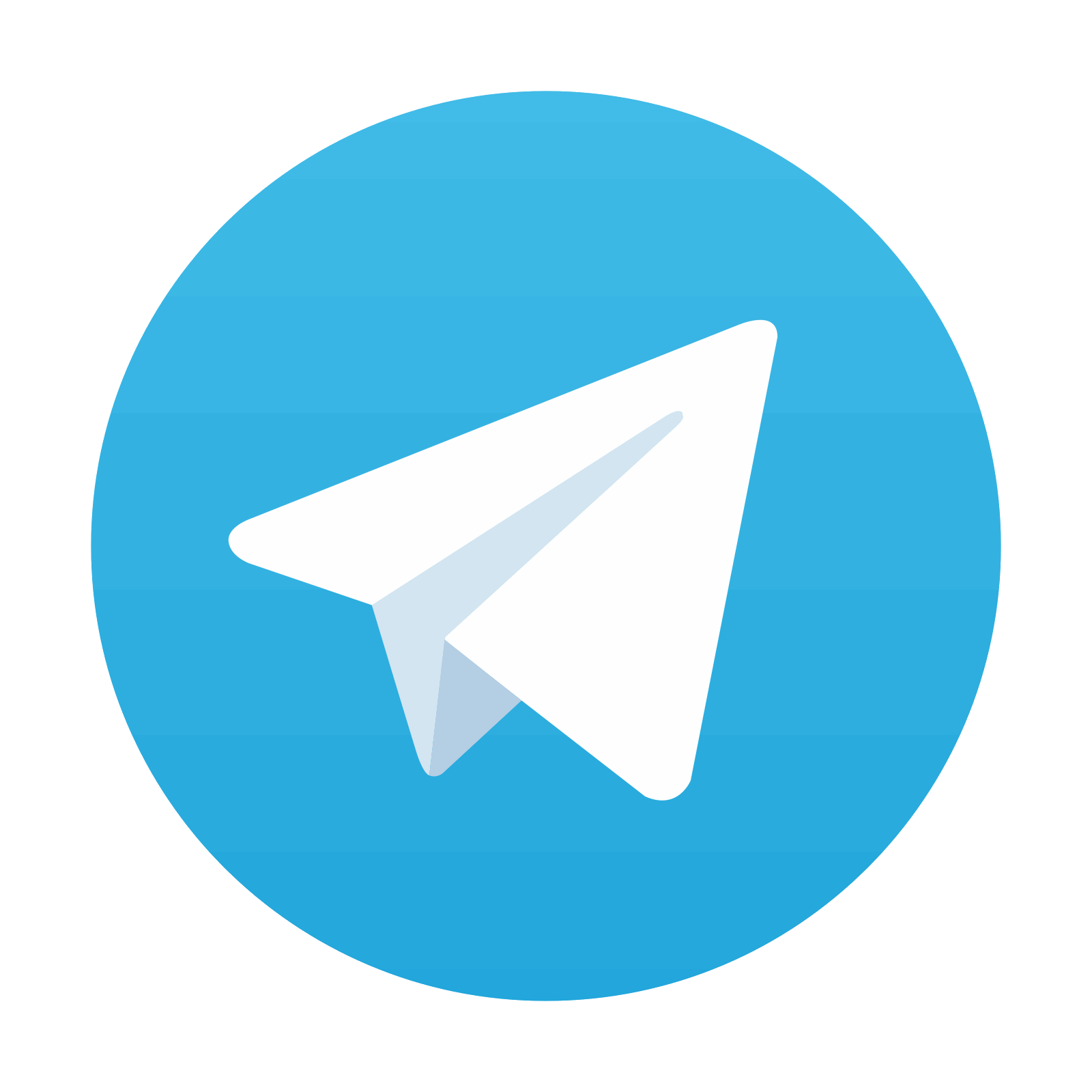
Stay updated, free articles. Join our Telegram channel

Full access? Get Clinical Tree
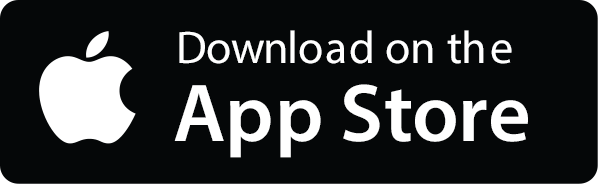
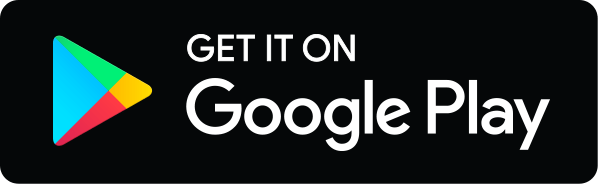