Prostaglandins and Hypotensive Lipids
The prostaglandins, thromboxanes, and leukotrienes are eicosanoids, which are metabolic products of 20-carbon arachidonic acid (Fig. 28.1). After the prostaglandins are synthesized, they are released and transported out of cells by transporters (1). The prostaglandins that reach the systemic circulation are inactivated in the lung and liver (2). The earliest physiologic effect observed for prostaglandins was contraction of the human uterus after exposure to seminal fluid (3). The initial ocular observation of miosis in the cat was noted after exposure to iris extracts (4). In rabbits, the topical application of 25 to 200 µg of prostaglandins caused an initial rise in intraocular pressure (IOP), followed by pressure reduction for 15 to 20 hours, whereas a 5-µg dose produced ocular hypotension without an initial pressure rise (5). Early studies on relatively large doses of topical prostaglandins revealed inflammation with conjunctival hyperemia and breakdown of the blood–aqueous barrier (6). Subsequent studies indicated that smaller amounts of prostaglandins lowered IOP, which led to the development of this drug class for the medical management of glaucoma.
Figure 28.1 Overview of the products derived from arachidonic acid metabolism.
Several important chemical modifications were made to improve the bioavailability and to make it a more selective FP-receptor agonist (Fig. 28.2) (7). The addition of a phenyl ring to the omega chain (i.e., latanoprost, travoprost, and bimatoprost) improved the selectivity for the FP receptor. To improve solubility, the C-1 carboxyl group was modified with an ethyl amide in the case of bimatoprost or an isopropyl ester for latanoprost, travoprost, and unoprostone. This modification at the C-1 carboxyl group creates a lipophilic prodrug, which is hydrolyzed by the cornea into the free acid drug form (8).
Figure 28.2 Representative structures for prostaglandinrelated agents. For the prostaglandin (PG) nomenclature, the last letter refers to the ring structure chemical modifications, the subscripted number is the number of double bonds, and the Greekletter subscript refers to the orientation of the hydroxyl group.
MECHANISMS OF ACTION
The prostaglandins have a mixed pharmacologic response because of the diversity of the receptors. The prostaglandin or prostanoid receptors include four subtypes (EP, FP, IP, and TP) of receptors for the endogenous prostaglandins, PGD2, PGE2, PGF2α, and PGI2 or TXA2, respectively (9). The prostanoid receptors are in the family of the G protein–coupled receptors (10). The prostanoid FP receptor exists in two forms: type A for the full-length receptor and type B for the splice variant, which is truncated or shortened compared with the full-length form (11). Both FP receptor forms couple to phospholipase C, which triggers the release of the second messenger inositol phosphate production and subsequently activates a molecular transduction cascade that leads to IOP reduction.
The prostanoid receptors are distributed widely in ocular tissues, which accounts for the diverse biologic effects of prostaglandins on the eye (12). The expression and distribution of the prostanoid receptors in the human eye have been determined with radioligand receptor binding studies and a variety of molecular methods (13–15). In most animal and human studies, the ocular hypotensive effect of prostaglandins, or hypotensive lipids, was not explained by reducing aqueous production, reducing episcleral venous pressure, or increasing conventional aqueous outflow (16–19). After binding and activating the FP receptors in the ciliary smooth muscle, the precise mechanism by which prostaglandins improve uveoscleral outflow (see Chapter 2) is not fully understood. Two possible mechanisms that have been studied are relaxation of the ciliary muscle and remodeling the extracellular matrix of the ciliary muscle.
In monkey studies, pilocarpine, which causes contraction of the ciliary muscle (see Chapter 32) and is known to reduce uveoscleral outflow, antagonized the PGF2α-induced ocular hypotension (20). The in vitro studies of ciliary muscle response to PGF2α have been conflicting. Prostaglandin F2α consistently relaxed carbachol-precontracted fresh ciliary muscle strips from monkey eyes (21). Similarly, trabecular and ciliary muscle contraction induced by endothelin was blocked by unoprostone, a docosanoid, which is a metabolite of docosahexaenoic acid (22). Studies in monkeys suggest a dual action of PGF2α on the ciliary muscle, involving a short-onset, long-lasting relaxation and narrowing of the muscle fiber bundles and a slowly developing, shorter-duration dissolution of the intermuscular connective tissue (23). It is apparent from these and other studies that the effects depend on the species studied and the effect of aging (24). The clinical use of pilocarpine and latanoprost is discussed in the section “Drug Interaction.”
There is greater evidence to support the mechanism of remodeling the extracellular matrix of the ciliary muscle. In cultured smooth muscle cells, PGF2α activates the FP receptors and initiates a signal transduction cascade that leads to the induction of the nuclear transcription factor and c-FOS (25). The c-FOS transcription factor binds to a special AP-1 transcription regulatory element in the promoter of certain genes, which leads to transcription of those particular genes (26). One gene class that is regulated by the AP-1 transcription regulatory element is the matrix metalloproteinase family (27). Specific molecules of the matrix metalloproteinase family degrade extracellular matrix substrates, such as certain collagens, fibronectin, or laminin. In ciliary muscle cultures, certain prostaglandin-like agents increase matrix metalloproteinases (28,29). Collagen types I, III, and IV, laminin, fibronectin, and hyalurons were reduced in cultured human ciliary muscle treated by the free acid of latanoprost and PGF2α (30). This prostaglandin-mediated increase in certain matrix metalloproteinases and change in extracellular matrix molecules seen in vitro in the cultured cells were observed in vivo.
In monkeys, immunohistochemical methods have identified increased expression of certain matrix metalloproteinases in ciliary muscle, iris root, and sclera (31). Both collagen type IV and myocilin (MYOC), formerly known as trabecular meshwork–inducible glucocorticoid response gene (TIGR) (see Chapter 8), appear to be colocalized in ciliary muscle, and topical PGF2α-isopropyl ester treatment decreases MYOC expression in monkey eyes (32). Prostaglandin-mediated changes in extracellular matrix metalloproteinases have been identified in ciliary muscle (33), which correlates with the reduction in collagen molecules within the uveoscleral outflow pathways (34). The collective evidence of data supports that the prostaglandins enhance uveoscleral outflow by remodeling the extracellular matrix in the uveoscleral outflow pathway, with possible contributions from some relaxation of the ciliary muscle and change in cell shape by cytoskeletal alteration.
Other possible effects of this class of drugs have also been studied. The evidence on the potential effect of the prostaglandin agents on ocular blood flow in the various vascular beds of the anterior segment, retinal, choroidal, and retrobulbar hemodynamics is not well understood (35). The interaction between prostaglandin agents and adrenergic pathways has been suggested based on the observation that adrenergic antagonists blocked the PGE2-induced increase in total outflow facility (36). It appears that the PGE class of prostaglandins has an additive effect in combination with latanoprost in lowering IOP in glaucomatous monkey eyes by enhancing trabecular outflow (37,38).
SPECIFIC AGENTS
Latanoprost
Approved for use in 1996, latanoprost was the first clinically practical prostaglandin for the treatment of glaucoma. Latanoprost, 0.005%, given once daily, has been compared with timolol, 0.5%, used twice daily in 6-month trials enrolling ocular hypertensive and glaucoma patients. Three studies included a total of 829 volunteers. In one of these trials, the diurnal IOP reduction at 6 months was 27% with timolol, 31% with latanoprost applied in the morning, and 35% with latanoprost used in the evening (39). In the other two comparative trials, latanoprost was given in the evening, with one study reporting 6-month diurnal IOP reductions of 32.7% and 33.7% for timolol and latanoprost, respectively, and the other reporting 6-month IOP reductions of 4.9 ± 2.9 mm Hg and 6.7 ± 3.4 mm Hg for timolol and latanoprost, respectively (40,41). Unlike timolol, latanoprost lowers the IOP at night and during the day, providing uniform, around-the-clock IOP reduction when administered once daily, alone or in combination with timolol (42).
The effect of latanoprost after 2 years of treatment was evaluated in 532 patients (496 and 113 were treated for 6 and 24 months, respectively) (43), who continued latanoprost monotherapy treatment as part of an open-label trial from the initial 6-month phase III study in Scandinavia and the United Kingdom (39). Over all, there was a similar mean IOP reduction at 2 years, with a decrease of 8.9 mm Hg (34%), compared with 6 months, with a decrease of 8.2 mm Hg (32%). Of the 532 total patients, 20% were withdrawn from treatment because of adverse events based on increased iris color, ocular adverse events, nonocular adverse events, high risk for increased iris color, or IOP treatment failure. For the patients with IOP treatment failure, insufficient IOP control was more common in persons with open-angle glaucoma than in those with ocular hypertension. Patients who initially started with higher baseline IOPs had a higher risk of IOP treatment failure with latanoprost monotherapy.
In addition to its effectiveness to treat ocular hypertension and open-angle glaucoma, latanoprost has also been examined in pediatric glaucoma. In general, latanoprost appears to be safe but tends to be less effective in lowering IOP in children, who have diverse forms of glaucoma treated with concomitant medical antiglaucoma therapies, compared with adults (44). Among the various forms of glaucoma in the pediatric population, it appeared that the older children and those with juvenile-onset open-angle glaucoma showed a better response to this drug (45).
Several prospective studies have examined the efficacy of latanoprost in lowering IOP in patients with chronic angle-closure glaucoma. A meta-analysis was conducted to evaluate the efficacy of prostaglandin analogs in 1090 patients from nine randomized clinical trials with chronic angle-closure glaucoma treated with latanoprost, bimatoprost, or travoprost monotherapy (46). The difference in absolute IOP reduction between the prostaglandin analogs and timolol varied from 0.4 to 1.6 mm Hg during the diurnal curve, 0.9 to 2.3 mm Hg at peak, and 1.3 to 2.4 mm Hg at trough. For latanoprost, the relative IOP reduction was 31% during the diurnal curve, 34% at peak effect, and 31% at trough effect. For timolol, the relative IOP reduction was 23% during the diurnal curve, 24% at peak, and 21% at trough. Based on this meta-analysis, latanoprost is at least as effective as timolol at reducing the IOP efficacy in eyes with chronic angle-closure glaucoma.
Another prospective observational case series of 137 Asian patients with chronic angle-closure glaucoma, which was defined as trabecular meshwork not visible for at least 180 degrees on gonioscopy, were treated with latanoprost (47). After 12 weeks of treatment, latanoprost reduced IOP from 25.0 ± 5.5 to 17.5 ± 5.0 mm Hg. The percentage change in IOP was not affected by the degree of angle narrowing or extent of synechial angle closure.
Three smaller studies also reported on the IOP-lowering effect of latanoprost in patients with chronic angle-closure glaucoma. In a study of 14 Korean patients with 360 degrees of peripheral anterior synechiae on gonioscopy, latanoprost, 0.005%, once daily reduced the IOP from 30.3 ± 4.5 mm Hg at baseline to 21.5 ± 5.9 mm Hg after 3 months of treatment (48). In a study of 36 Japanese patients with chronic angle-closure glaucoma treated by laser iridotomy, the effects of latanoprost alone versus the unfixed combination therapy of timolol maleate, 0.5%, and dorzolamide hydrochloride, 1%, were compared (49). After 12 weeks of treatment, latanoprost reduced the IOP by 33%, from 22.2 ± 2.0 to 14.8 ± 1.9 mm Hg. Timolol maleate and dorzolamide hydrochloride reduced IOP by 24%, from 22.5 ± 2.2 to 17.1 ± 2.7 mm Hg. Finally, in 32 patients who had previous peripheral iridectomy and inadequate IOP, half of the group was randomly assigned to latanoprost and the other half to timolol (50). Latanoprost lowered the IOP by 8.8 mm Hg (34% reduction from a baseline of 25.7 mm Hg), compared with 5.7 mm Hg for timolol (23% reduction from a baseline of 25.2 mm Hg). Thus, when IOP elevation persists after peripheral iridectomy in patients with primary angle-closure glaucoma, latanoprost is effective in lowering the IOP.
Unoprostone
Unlike the 20-carbon molecular skeleton of arachidonic acid, unoprostone, also known as UF-021, may be considered a docosanoid, which is a 22-carbon molecule (Fig. 28.2). Unoprostone has been in clinical use in Japan since 1994 in a 0.12% formulation. Unoprostone, 0.15%, became available for clinical use in the United States in 2000. Studies in Japan have shown that unoprostone, 0.12%, administered two times daily lowered IOP by 11% to 23% from baseline (51–53).
Comparison studies between unoprostone and timolol have shown that unoprostone is not as effective as timolol at decreasing the IOP (54,55). The effectiveness of unoprostone has also been compared with latanoprost. In a comparative parallel-group study in a total of 108 patients, there was a mean IOP reduction of 6.7 mm Hg in the group treated with latanoprost, compared with 3.3 mm Hg lowering in the unoprostone-treated group (56). Similar results showing better efficacy of latanoprost compared with unoprostone were shown in a smaller study of patients with ocular hypertension (57).
A recent study demonstrated that unoprostone increased activity of certain tissue inhibitors of matrix metalloproteinase in cultured human ciliary body smooth muscle cells, compared with bimatoprost and latanoprost (58). It is proposed that this differential effect of the prostaglandin agents may explain the lower clinical efficacy of unoprostone compared with the other agents.
Travoprost
Travoprost, also known as AL-6221, was approved for clinical use in the United States in 2001 (59). The effectiveness of travoprost has been compared with that of timolol and latanoprost.
In a 9-month clinical trial with 573 patients, travoprost showed a 30% to 33% decrease in IOP from baseline compared with a 25% to 29% decrease in IOP in patients using timolol, 0.5%, twice daily (60). In a 6-month comparative study of travoprost and timolol involving 605 patients, the IOP decrease from baseline ranged from –6.5 to –8.0 mm Hg for travoprost, 0.004%, and –5.2 to –7.0 mm Hg for timolol (61). In a 12-month comparative clinical trial of travoprost, latanoprost, and timolol involving 801 patients, the IOP-lowering effect of travoprost was greater than the timolol and similar to that of latanoprost (62). In another clinical trial of 426 patients with uncontrolled IOP on timolol alone, travoprost led to further, significant IOP reduction after 6 months of treatment (63).
Travoprost has also been shown to be effective in lowering IOP in patients with chronic angle-closure glaucoma (46,64).
Travoprost was observed to be more effective in lowering IOP compared with latanoprost in blacks compared with nonblack patients (62,65). However, subsequent studies have not reproduced this observation. In a small, randomized, investigator-masked multicenter study, 83 patients with open-angle glaucoma were self-identified as white or other (i.e., African, East Indian, Asian, or Hispanic) and were randomly assigned to receive one of three prostaglandin–prostamide drugs (66). After 24 weeks of treatment, there was a significant decrease in IOP compared with baseline. In this study, there were no differences in treatment effect between the three drugs or between the two ethnic groups, and there was no interaction between race and drug. The results from this smaller study confirmed the observation in the larger Ocular Hypertension Treatment Study (67). The IOP response to prostaglandin analogs was slightly greater in self-declared African American participants compared with whites, but this difference was not statistically significant. The greater IOP reduction was associated with a higher baseline IOP and a thinner central corneal measurement.
Bimatoprost
Bimatoprost, which was also known as AGN 192024, was approved for clinical use in the United States in 2001. Its chemical structure differs from PGF2α and the other prostaglandin analogs (Fig. 28.2) with an amide ethyl group at the C-1 position. There is growing evidence to support the designation of this agent as a prostamide, given the identification of the cyclooxygenase-2 (COX-2)–derived oxidation products of the endocannabinoids and the recent discovery and development of prostamide antagonists (68–70).
Bimatoprost is hydrolyzed by the cornea to a lesser extent than with latanoprost, unoprostone, and travoprost into the active free acid form of the drug (8). The IOP lowering of bimatoprost (30.4% lowering with once-daily dosing) is greater than that of timolol (26.2% lowering with twice-daily dosing) (71). Similar treatment results have been shown in other clinical studies comparing bimatoprost and timolol (72,73). Bimatoprost has been compared with latanoprost and showed a similar IOP-lowering effect (74–76). In a community-based “switch study” involving 1283 patients who were changed from latanoprost to bimatoprost treatment, there was a mean IOP reduction of 3.4 mm Hg after 2 months of bimatoprost treatment (77). In such a “switch” study, however, the observed IOP reduction may simply reflect a regression to the mean with repeated IOP measurements over time (78).
Bimatoprost has also been shown to lower IOP in patients with chronic angle-closure glaucoma. In a meta-analysis of nine randomized clinical trials enrolling a total of 1090 patients, bimatoprost lowered the diurnal IOP curve by 26%, the peak IOP by 28%, and the trough IOP by 27% (46). Timolol lowered the diurnal IOP curve by 23%, the peak IOP by 24%, and the trough IOP by 21%. Other studies also showed that bimatoprost was effective compared with latanoprost in lowering IOP in chronic angle-closure glaucoma (79,80).
There is some evidence to support that bimatoprost has both FP-receptor agonist activity and possibly another mechanism of action related to trabecular outflow and an alternative signaling pathway based on an assay in feline iris (70,81,82).
ADMINISTRATION
Latanoprost, 0.005%, travoprost, 0.004%, and bimatoprost, 0.03%, are administered similarly as one drop daily to the eye. More frequent dosing of these particular agents resulted in reduced efficacy of the drug (71,83). In contrast, unoprostone, 0.15%, is administered as one drop two times daily to the eye.
The dosing regimen for this drug class is unusual given that the pharmacokinetic data indicate a very short plasma elimination half-life (t1/2 life of 9.2 minutes after intravenous administration and 2.3 minutes after topical administration for latanoprost; t1/2 life of 45 minutes after intravenous administration for bimatoprost) (84–86). This molecule is dosed at what appears to be homeopathic levels, but at this dose, it has profound clinical effects on IOP reduction in most patients. The explanation for this dosing regimen and the efficacy is not fully understood on the basis of our present knowledge of the pharmacology and mechanism of action of this drug class (as discussed in the earlier section).
The issue of the effect of storage temperature has been raised with these agents because one study reported that latanoprost exhibits thermal and ultraviolet instability (87). This raises issues related to chemical stability of the molecule and to the role of the composition of the dispensing system on bioavailability of the drug. A comparative study with the other agents in this drug class has not been reported. It has been suggested that unopened bottles of latanoprost be stored under refrigeration. Once opened, latanoprost may be stored at room temperature (as high as 25°C, or 77°F) for up to 6 weeks. The other agents are recommended to be stored at room temperature (15°C to 25°C, or 59°F to 77°F, for bimatoprost; 2°C to 25°C, or 36°F to 77°F, for travoprost and unoprostone).
DRUG INTERACTION
Given the additional 13% to 37% IOP lowering by combining a uveoscleral outflow drug together with the aqueous humor inflow suppressant timolol (88,89), fixed combinations of the prostaglandin agents with timolol have been studied. In other countries, the fixed combination of latanoprost, 0.005%, and timolol, 0.5%, was approved for clinical use in Europe during the summer of 2001 and has been shown to be effective and comparable with each component given separately (90–92). The fixed combination of travoprost, 0.004%, and timolol, 0.5%, and bimatoprost and timolol, 0.5%, are also available in other countries (93–97). At present, none of these agents are approved for use in the United States.
The combination of the prostaglandin agents in addition to oral and topical carbonic anhydrase inhibitors (CAIs) have been examined. In 24 patients with glaucoma, a mean IOP of 19.5 mm Hg was achieved while on treatment with acetazolamide (250 mg twice daily), which decreased to 16.8 mm Hg, which was a 15% IOP reduction, after 15 days of combination treatment with latanoprost (0.005% once daily) and acetazolamide (250 mg twice daily) (98). Topical CAIs were also shown to have additional IOP-lowering effect in combination with latanoprost (99). A similar effect was observed with travoprost (100,101).
The combination of the prostaglandin agent bimatoprost and the α2-adrenergic agonist brimonidine has been compared with the combination of timolol and latanoprost in 28 patients (102). The mean IOP at baseline was 24.8 mm Hg. There was a decrease of 8.5 to 9.0 mm Hg after treatment with bimatoprost combined with brimonidine, and 7.5 to 7.7 mm Hg after treatment with latanoprost combined with timolol. Another study compared combination therapy of brimonidine and latanoprost to the fixed combination of timolol and dorzolamide in patients with glaucoma or ocular hypertension (103). The brimonidine and latanoprost combination was associated with a mean IOP reduction of 9.2 mm Hg. This IOP decrease with use of the combination of the prostaglandin-related drugs and brimonidine is greater than the decrease of 6.7 mm Hg with use of the timolol–dorzolamide fixed combination.
Initially, the interaction of latanoprost with miotic agents, such as pilocarpine, was not clear based on the early observation that pilocarpine antagonized PGF2α-induced ocular hypotension in monkeys (20). This observation was not surprising because pilocarpine contracts the ciliary muscle, which can both decrease uveoscleral outflow and increase trabecular outflow facility by pulling on the scleral spur (see Chapter 32). In contrast, latanoprost lowers IOP by improving uveoscleral outflow, possibly through relaxation of the ciliary muscle, but primarily through remodeling the extracellular matrix (see “Mechanisms of Action”). This is consistent with an ultrasound biomicroscopy study of 36 healthy young Japanese persons showing that pilocarpine, 2%, increased the ciliary body thickness by 8.3% and latanoprost, 0.005%, decreased the thickness by 3.3% (104).
However, in clinical trials, it became apparent that pilocarpine did not impair the IOP-lowering effect of latanoprost (105), and it was demonstrated that these drugs were additive by fluorophotometric, pneumotonometric, and venomanometric methods (106). In a study of 20 patients with ocular hypertension who were initially treated with pilocarpine, 2%, three times daily or latanoprost, 0.005%, twice daily, the IOP reduction at the end of 1 week was 14.3% on pilocarpine alone and 23.4% on latanoprost alone (107). When pilocarpine was then added to the latanoprost, the additional IOP reduction was 7.4%, compared with 14.2% when latanoprost was added to pilocarpine. In patients who were on maximal medical therapy that included miotics, latanoprost had an additive effect, with additional IOP lowering (108,109). Although the use of miotic therapies has declined with the availability of other drug classes with fewer ocular side effects and less frequent dosing, the miotic agents are expected to have an additive effect in combination with the prostaglandin-related drugs.
In general, there are few studies to support switching within the class of the prostaglandin agents. It has been suggested that unoprostone may have additive IOP lowering to latanoprost on the basis of a study of 41 patients (110). This additive effect was not supported in another study of 52 patients in which half the patients were initially treated with latanoprost for 6 weeks followed by adding unoprostone, and the other half of patients were treated initially with unoprostone and later adding latanoprost (111). The combination of unoprostone with latanoprost did not result in further IOP lowering compared with latanoprost alone. It appears that unoprostone is not likely very useful as an adjunctive prostaglandin agent in patients who are already on another prostaglandin agent.
There is awareness that a small number of patients are nonresponders to latanoprost (112,113), which would also be expected for the other prostaglandin-related agents. In 15 patients who were classified as nonresponders to latanoprost, which was defined as less than 10% IOP lowering after 6 to 8 weeks of latanoprost, 0.005%, administered once daily, bimatoprost (18.2 mm Hg on bimatoprost treatment) was more effective than latanoprost (24.1 mm Hg on latanoprost treatment) (114). The mechanism to explain the variation in IOP response to latanoprost versus bimatoprost in the same group of patients is not understood.
SIDE EFFECTS
Several side effects have been reported for the prostaglandin class of glaucoma medications that appear unique to this drug class compared with the other types of glaucoma medications. Most of these side effects are associated with use of latanoprost, because clinical experience has been longer with this agent than with unoprostone, bimatoprost, or travoprost. In general, these side effects are not common and a causal relationship has not been established. Although some of these side effects were not apparent in the well-designed glaucoma pharmacology clinical trial, it should be appreciated that new side effects or unanticipated effects are observed in the postmarketing use of a drug in individual patients who do not reflect the average participant in the clinical trials.
In general, based on the various clinical trials, the prostaglandin-related agents were associated with greater conjunctival hyperemia compared with timolol-treated eyes (39–41,60,71). The hyperemia frequency rates in the product labeling are 5% to 15% for latanoprost, 0.005%; 15% to 45% for bimatoprost, 0.03%; 35% to 50% for travoprost, 0.004%; and 10% to 25% for unoprostone, 0.15%. The tremendous variation in the frequency of this side effect among the clinical trials depended on the particular dosing regimen used and the method used to ascertain this clinical finding, which may be elicited by patient report, by discontinuation rate for hyperemia, by grading based on clinical photographs, or by investigators’ severity scoring (115). Several studies have compared the prostaglandin-related drugs to each other, and in general, all the prostaglandin-related agents were associated with some degree of conjunctival hyperemia (57,62,75,116).
The effect of latanoprost on conjunctival fibroblasts and conjunctiva has been examined. In rabbits treated with topical latanoprost, there was upregulation of matrix metalloproteinase type 3 compared with control and timolol-treated eyes (117). Latanoprost increased the number of fibroblasts that stained for proliferating cell nuclear antigen, a marker for proliferating cells, compared with control treated rabbit eyes (118). With 6 months of latanoprost treatment in patients, the drug appeared to have transient effects on goblet cell density and was associated with a decrease in conjunctival epithelial cell size based on impression cytology staining comparing the status before and after treatment (119). A control patient population was not examined in that study. The effect of these agents on conjunctival biology and function is not fully understood regarding the impact of glaucoma drugs on the tissues and outcome of filtration surgery.
The main concern for corneal toxicity associated with prostaglandin treatment is an eye with a history of herpes simplex keratitis. There are case reports of reactivation of herpes simplex keratitis in patients treated with latanoprost or bimatoprost (120,121). In a rabbit model, topical latanoprost increased the severity and recurrence or herpetic keratitis (122), and unoprostone did not (123). In a patient with a history of herpetic keratitis, an alternative drug class would be prudent for initial drug treatment for glaucoma. Superficial epithelial lesions of the cornea have been reported after latanoprost treatment (40,124). In a 1-year clinical trial comparing latanoprost, fixed-combination latanoprost–timolol, and timolol, all three treatments had similar long-term corneal effects with little change in corneal endothelial cell density and corneal thickness (125).
The intraocular inflammatory effects of aqueous cell and flare and miosis, which result from the administration of large doses of prostaglandins, were not seen in animal or human eyes in the doses associated with the ocular hypotensive response (126–128). The absorptive transport systems of the ciliary processes appear to prevent topically applied prostaglandins and other eicosanoids from causing retinal toxicity (129). Although, in general, this class of drug is well tolerated in a large number of patients, a few patients developed anterior uveitis while on latanoprost or cystoid macular edema after treatment with latanoprost (130–134). Similarly, there are reported cases of cystoid macular edema associated with use of unoprostone, travoprost, and bimatoprost (135). Cautious use of this class of drugs is advised in eyes with risk factors for cystoid macular edema (135,136).
The most visible side effect of the prostaglandin drug class is increased pigmentation in the periocular skin and iris and alterations in the eyelid cilia. Increased periocular skin pigmentation has been reported with the use of the prostaglandin agents (137). Instructing patients to wipe off any excess drops on the periocular skin area is important to minimize exposure of this area to this effect, which is reversible upon cessation of the drug exposure. Darkening of the iris was seen in 10% of the patients in one study (39), and also with the other prostaglandin agents (138). This was reported to occur more commonly in eyes with mixed green-brown or blue–gray-brown iris color (Fig. 28.3) but has also been reported in a brown iris (39,139). The mechanism appears to be similar in the iris and epidermal melanocytes with upregulation of tyrosinase activity in melanocytes (140,141). In the pigmented rabbit model of lighter colored iris from sympathetic denervation, treatment of this eye with latanoprost darkened the iris in the denervated eye (142). Both light and electron microscopy studies indicate that the melanin content is increased in iris stromal melanocytes without proliferation of cells (143–145). Because the alteration in iris color relates to iris stromal melanocytes, this is not expected to be related to open-angle glaucoma related to pigment dispersion (see Chapter 17), which in general is related to release of intraocular pigment by disruption of the pigmented epithelium on the posterior surface of the iris.
Figure 28.3 Increased pigmentation of the iris in a patient taking prostaglandin therapy for glaucoma for 10 months. This side effect occurs primarily in patients with green-brown or blue–gray-brown iris color. (From Watson P, Stjernschantz J. A six-month, randomized, double-masked study comparing latanoprost with timolol in open-angle glaucoma and ocular hypertension. The Latanoprost Study Group. Ophthalmology. 1996;103(1):126–137, with permission.)
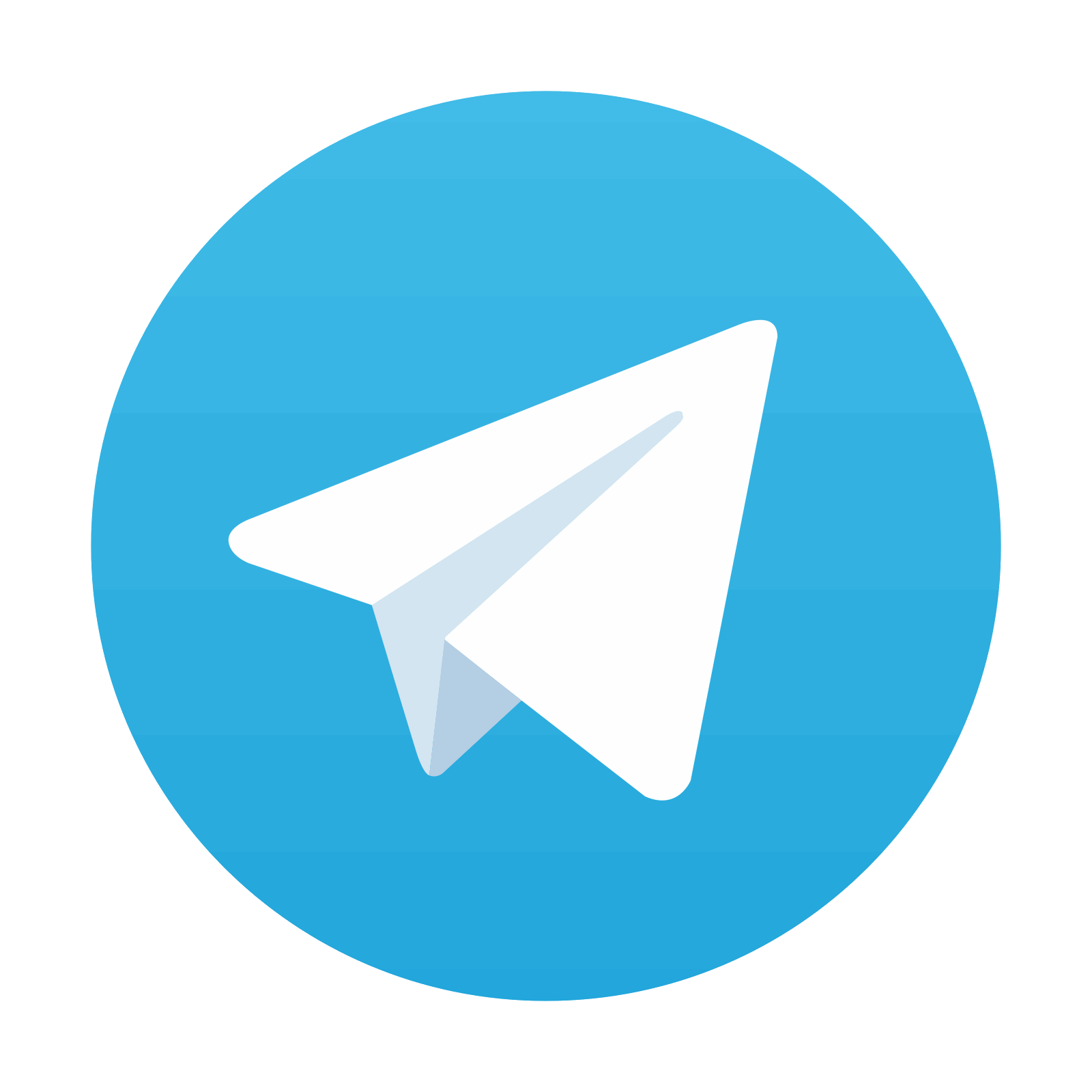
Stay updated, free articles. Join our Telegram channel

Full access? Get Clinical Tree
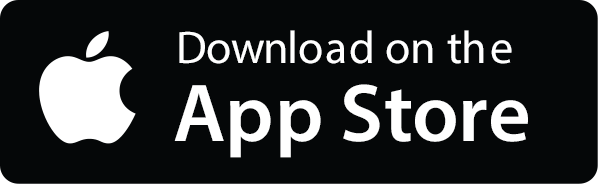
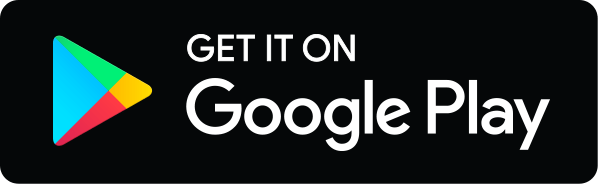