Chronic Open-Angle Glaucoma and Normal-Tension Glaucoma
TERMINOLOGY
Chronic Open-Angle Glaucoma
As discussed in Chapter 7, the glaucomas have traditionally been classified according to primary and secondary forms. Within the former group, and indeed among all the glaucomas, by far the most prevalent condition has been commonly referred to as primary open-angle glaucoma. Continued research, however, has shown the concept of primary and secondary glaucomas to be arbitrary and one that should probably be abandoned. Research has also suggested that the view of primary open-angle glaucoma as a single entity is no longer valid. An alternative term, which we have elected to use in this text, is chronic open-angle glaucoma (COAG). Other synonymous terms that may also appear in the literature include chronic simple glaucoma, idiopathic open-angle glaucoma, and open-angle glaucoma. COAG is typically characterized by (a) an open, normal-appearing anterior chamber angle and increased intraocular pressure (IOP) without any apparent ocular or systemic abnormality that might account for the elevated IOP and (b) typical optic nerve head damage or glaucomatous visual field damage (as described in Chapters 4 and 5, respectively). A proposed definition of COAG (modified from the American Academy of Ophthalmology Preferred Practice Guidelines, 2005 (1)) is a multifactorial optic neuropathy in which there is characteristic atrophy of the optic nerve. Although abnormally elevated IOP had long been considered part of the definition, it is now considered a risk factor for COAG.
Ocular Hypertension or Glaucoma Suspect
Patients who have an IOP above 21 mm Hg for which there is no apparent cause but whose optic nerve heads and visual fields are normal are commonly said to have ocular hypertension (2,3). Chandler and Grant (4) suggested the term “early open-angle glaucoma without damage” for this condition, whereas Shaffer (5) preferred the term “glaucoma suspect” (see Chapter 10). The latter term may also include other factors that make the possibility of glaucoma more likely, such as suspicious optic nerve heads or visual fields. Whatever term one chooses to use for this condition, the most important point is that both physician and patient be fully aware of its potential consequences.
Normal-Tension Glaucoma
At the other end of the spectrum with regard to susceptibility to high IOP are patients with open, normal-appearing anterior chamber angles who have glaucomatous optic nerve head and visual field damage despite pressures that have never been documented above 21 mm Hg. These patients are said to have normal-tension glaucoma (NTG). The term “low-tension glaucoma” has also been used, although the IOP in these individuals is usually “normal” or “high normal” and is rarely “low normal.” The criteria used to define NTG over the past 25 years have been highly variable (6). Some investigators believe that NTG is a variant of COAG, whereas others believe that the mechanism of optic atrophy in the two conditions is different (7). Although a number of differences between the two disorders have been described (see later text), COAG and NTG appear to represent a continuum of glaucomas in which the mechanism of the glaucomatous optic neuropathy shifts from predominantly elevated IOP in the former to additional IOP-independent factors in the latter, with considerable overlap of causative factors.
Chronic Open-Angle Glaucomas with Associated Abnormalities
Some forms of open-angle glaucoma, such as pigmentary glaucoma and the exfoliation syndrome, have been identified as distinct entities because of a partial understanding of associated, causative abnormalities and mechanisms of aqueous outflow obstruction. (These conditions are discussed in this section of the book.) Here, the focus is on those open-angle glaucomas for which laboratory and clinical findings have yet to clarify the glaucoma mechanisms and in which IOP plays a variable role. As the search continues into the causes and mechanisms of the open-angle glaucomas, especially in the field of molecular biology, an ever-increasing number of separate entities will likely be recognized within this spectrum of disorders.
EPIDEMIOLOGY
Significance of Intraocular Pressure
The commonly used IOP level of 21 mm Hg is based on the concept that two standard deviations above the mean within a Gaussian distribution for the white population represents the upper limit of “normal” for that biological parameter. However, because the distribution of IOP in the general population is skewed to the right, or to higher pressures, this principle provides only a rough approximation of the normal limits. More important, many eyes will not develop glaucomatous optic atrophy or visual field loss, at least not for long periods of time, despite having IOP well above 21 mm Hg, whereas others will have progressive glaucomatous damage at pressures that are never observed to exceed this level. These latter observations have brought into question the role of IOP in the mechanism of COAG. Even though many studies have confirmed a correlation between the level of IOP and the rate of visual field loss in some groups of patients with COAG, this correlation is not seen in all cases (8–10). Other causative factors figure into the formula for glaucomatous damage, which appears to explain the lack of absolute correlation between IOP and the development of COAG. In any case, this discrepancy between IOP level and glaucomatous damage has led to the use of additional terms within the general category of COAG, and these are reviewed hereunder.
Frequency among the Glaucomas
COAG is clearly the most common single form of glaucoma, although it is difficult to precisely establish the proportion of individuals with this disorder to the total number of patients with all forms of glaucoma. In a British survey of 4231 individuals between the ages of 40 and 75 years, one third of the glaucoma population and 0.28% of the general population had COAG (11). However, in a study of 8126 individuals in Japan who were at least 40 years of age, COAG accounted for 73% of the glaucomas detected (exclusive of patients with ocular hypertension), of which most were NTG (12). These epidemiologic surveys will obviously be influenced by the population being studied as well as the methods and criteria used to identify patients with glaucoma.
Prevalence in General Populations
Several large surveys have been conducted to determine the number of patients with ocular hypertension and COAG (or glaucoma in general) within a population at a given time (reviewed in Chapters 9 and 10). The prevalence of glaucoma in persons older than 40 years is between 1% and 2% in most studies, although reports again vary considerably according to the population studied and the diagnostic criteria and screening techniques used (13,14).
Natural History of Visual Field Loss in Chronic Open-Angle Glaucoma
Leydhecker (15) studied the distribution of IOP and glaucomatous visual field loss in a large population survey. When persons with pressures higher than 20 mm Hg and those with definite glaucomatous field defects were plotted against their age, the two slopes were parallel and separated horizontally by 18 years, which led to the notion that 10 to 20 years may elapse between the onset of ocular hypertension and the development of visual field loss. Lichter and Shaffer (16), however, found that field loss in a population of 378 patients with ocular hypertension, observed for an average of 12.75 years, occurred earlier than Leydhecker suggested, even though most were being treated during that time. The level of IOP appears to influence the rate of visual field loss. In one study of 177 untreated patients with COAG comparing the mean age of presentation with the degree of field loss, it was estimated that untreated disease is likely to progress from early to end-stage visual field loss in 14.4 years at pressures of 21 to 25 mm Hg, in 6.5 years at pressures of 25 to 30 mm Hg, and in 2.9 years at pressures greater than 30 mm Hg (17). Furthermore, once field loss has occurred, further damage tends to progress more rapidly than in the fellow undamaged eye exposed to the same IOP, which appears to reflect the increased susceptibility of the damaged eye (18–20).
The “natural” course of NTG was evaluated in the Collaborative Normal-Tension Glaucoma Study (CNTGS) during the time before randomization and in patients assigned not to receive treatment (21). About one third of patients showed confirmed localized visual field progression at 3 years, and about one half showed further deterioration at 7 years. The change was typically small and slow, often insufficient to measurably affect the mean deviation index, and there was tremendous variability in progression rates, with women, older individuals, or those with a disc hemorrhage, or history of migraine having a greater risk for progression. In the Early Manifest Glaucoma Trial, 76% of patients demonstrated progression on specific optic nerve or visual field endpoints after an average 4 years of follow-up (22).
Identifying Patients and Those at Increased Risk
COAG has no associated symptoms or other warning signs before the development of advanced visual field loss. It is for this reason that public and family physician awareness programs are needed to ensure that high-risk patients receive glaucoma assessment examinations by eye care specialists. Such programs must use the systemic and ocular risk factors discussed in Chapter 9, which are commonly associated with the disease, to identify those segments of the population requiring the closest attention. In addition, once a patient has been found to have persistent IOP elevation (the most significant risk factor) but no apparent optic nerve head or visual field damage, the additional risk factors must be considered by the physician when trying to decide which of these individuals require closer observation or the initiation of therapy before definite damage occurs. (In Chapters 9 and 10, the risk factors for developing COAG and the use of these factors in determining the frequency of periodic eye examinations for detection of glaucoma are discussed.)
CLINICAL DIFFERENCES BETWEEN NTG AND COAG
Chronic Open-Angle Glaucoma
Intraocular Pressure
Measured IOP greater than 21 mm Hg before treatment is generally considered elevated. Even though an elevated IOP is only one of several risk factors for COAG, it is a causative risk factor, and most studies agree that it is the single most important risk factor.
Central Corneal Thickness
The cornea is typically normal in COAG. Measurement of central corneal thickness with ultrasonic or optical methods is helpful in interpreting the accuracy of applanation tonometry readings as well as in assisting with estimating the risk for progression. Published evidence regarding the value of central corneal thickness for prognostic information is strong in the case of patients with ocular hypertension but is considerably weaker in patients with established glaucoma (23). Hence, in patients with established COAG, a thinner cornea (if structurally normal) signifies that the “true” IOP is higher than measured but that the risk for progression may or may not be higher.
Anterior Chamber Angle
By traditional definition, the anterior chamber angle in eyes with COAG is open and grossly normal on gonioscopic examination (Chapter 3). Preliminary studies, however, suggest that these patients may have more iris processes, a higher insertion of the iris root, more trabecular meshwork pigmentation (24), and a greater-than-normal degree of segmentation in the pigmentation of the meshwork (25).
Optic Nerve Head
The appearance of the optic nerve head and peripapillary retina is the single most important clinical feature in establishing the presence of glaucomatous damage. A helpful early finding is defects in the retinal nerve fiber layer, which may be a sign of glaucomatous optic atrophy before apparent changes are seen in the nerve head (26). Other early findings include enlargement of the optic dose cup, thinning or saucerizing of the neural rim, disc hemorrhages, and peripapillary atrophy (as explained in Chapter 4).
Visual Abnormalities
Central visual acuity, as measured by standard clinical tests, typically remains normal until there is marked visual field loss within the central visual field. How little remaining central visual field is necessary to retain excellent visual acuity is often remarkable. Therefore, in cases where visual acuity is reduced while significant portions of the central 5 to 10 degrees are retained, other nonglaucomatous causes for visual acuity loss should be considered. Preliminary evidence, however, suggests that more subtle measures of vision dysfunction, such as contrast sensitivity, color vision, and motion perception (discussed in Chapter 6), may one day be useful as early indicators of visual dysfunction before the development of typical visual field loss. Once typical glaucomatous damage to the visual field has been documented in one eye, there is a high incidence of subsequent field loss in the fellow eye. The latter was reported to be 29% in 31 patients followed up for 3 to 7 years (27), and 25% of 104 individuals after 5 years of follow-up in another series (28).
Normal-Tension Glaucoma
As noted earlier, some investigators consider NTG to be clearly distinguishable from the high-tension form of COAG, but others do not. COAG likely is a spectrum of disorders in which elevated IOP is the most influential causative factor at one end, whereas other IOP-independent factors that influence glaucomatous optic atrophy predominate at the other end. In any case, clinical differences between NTG and COAG are considered here.
Optic Nerve Head
Some investigators have found the neural rim to be significantly thinner in patients with NTG, especially inferiorly and inferotemporally, than in other patients with COAG who have similar total visual field loss (7). Other studies have revealed less striking differences, with considerable overlap between high-tension glaucoma and NTG. A study of morphologic characteristics of the optic nerve head in high-tension glaucoma and NTG eyes showed no significant difference in any parameter as measured by laser scanning ophthalmoscopy (29).
Some studies have found that optic disc hemorrhages were more prevalent in the group with NTG, raising the possibility of vascular disease as another causative factor in these patients (7). The retinal nerve fiber layer has also been compared between patients with NTG and those with COAG, with the former having more localized defects, closer to the macula, and the latter more diffuse defects (30–32).
Visual Fields
Differences have also been reported in the nature of visual field loss between patients with NTG and those with COAG who have similar optic nerve damage. In general, patients with NTG appear to have deeper, more localized scotomas (33). There are also conflicting reports regarding the proximity of scotomas to fixation between the two groups, which may relate to the testing methods. One study found a significantly greater rate of progressive visual field loss in NTG (34), and another revealed a difference in the pattern of the progression, with the patients with high-tension glaucoma initially increasing mainly in area and later in depth, whereas the increases in area and depth remained in constant proportion in patients with NTG (35).
Intraocular Pressure
Although NTG, by definition, is distinguished from high-tension COAG by an IOP that is never recorded to exceed 21 mm Hg, the pressures do tend to be higher than those in the general healthy population (36). A number of studies have revealed a significant influence of IOP on the progression of visual field or neuroretinal rim damage in NTG (37,38), although another study showed no significant difference in IOP between patients with and those without field progression (39). In some studies of patients with NTG and asymmetric IOP, the visual field loss was typically worse in the eye with the higher pressure (40,41). However, a more rigorous, prospective evaluation of 190 patients with NTG in the Low-Pressure Glaucoma Treatment Study found IOP asymmetry to be unrelated to visual field asymmetry (42).
A randomized trial of treated versus untreated patients with NTG has convincingly shown that an IOP reduction of at least 30% is associated with protection of visual field and nerve status, thus validating the concept that IOP is a contributory factor in the optic neuropathy of NTG. The bulk of the evidence, therefore, suggests that IOP in the high-normal range is a causative factor in NTG, although other factors are also involved. In making the diagnosis of NTG and in the management of these patients, it is important to know the diurnal variation in IOP to confirm that their pressures are consistently below 21 mm Hg before therapy and are staying within the target level while on treatment. One study suggested that patients with NTG have wider diurnal fluctuations than the general healthy population (36), although other investigators found no significant difference in diurnal variation of IOP or of aqueous humor flow or resistance to outflow (43,44).
It is also important to note that IOP spikes may occur at night, and therefore IOPs measured during office hours may miss nocturnal spikes in many patients (7). The concomitant changes of nocturnal orbital blood pressure and IOP may affect blood perfusion to the optic nerve head differently in glaucomatous eyes, compared with healthy eyes, and this also might affect the susceptibility of the optic nerve to damage. When a patient has progressive visual field loss or optic disc or retinal nerve fiber layer damage in the presence of an apparently well-controlled IOP during the day, it is appropriate to consider that the nocturnal IOP (during sleep) may be elevated (45). Obtaining 24-hour IOP measurements is often difficult if not impossible. Furthermore, even if this were possible, whether the nighttime readings reflect the true IOP during sleep is unclear.
Ocular Vascular Abnormalities
As noted earlier in this section, additional causative factors may relate to the architecture of the lamina cribrosa and the vascular perfusion of the optic nerve head. Drance and coworkers (46,47) described two forms of NTG: (a) a nonprogressive form, which is usually associated with a transient episode of vascular shock, and (b) a more common progressive form, which is believed to result from chronic vascular insufficiency of the optic nerve head. Various cardiovascular and hematologic abnormalities have been described, which might account for both forms (48). Reported associated findings include hemodynamic crises, reduced diastolic ophthalmodynamometry levels and ocular pulse amplitudes, bilateral complete occlusion of the internal carotid artery with reversed ophthalmic artery flow, focal arteriolar narrowing around the optic nerve, and increased vascular resistance of the ophthalmic artery by color Doppler analysis (46,47,49–52). It is wise to consider untreated glaucomatous optic neuropathy to be a progressive rather than a quiescent process.
Systemic Vascular Abnormalities
Reports of alterations in systemic blood pressure are conflicting. However, patients with NTG have significantly greater nocturnal blood pressure drops than healthy persons (53), as well as elevated diastolic blood pressure (54). Twenty-four–hour electrocardiographic monitoring has shown significantly greater asymptomatic myocardial ischemia in patients with NTG (45%) than in healthy individuals (5%), with many ischemic episodes occurring during the night (55). Visual-evoked responses during stepwise artificially increased IOP were significantly different between patients with NTG and those with high-tension COAG, suggesting a greater lack of autoregulation of optic nerve head circulation in the former group (56). In patients with NTG whose disease is progressing despite seemingly normal IOP, it may be appropriate to request 24-hour blood pressure monitoring, where available, to look for dips in nocturnal blood pressure and alterations of perfusion pressure to the optic nerve.
Patients with NTG were noted to have an increased frequency of headaches with or without migraine features (57). Another study failed to confirm this association (58), whereas a third investigation found that patients with NTG and headaches had significantly lower IOP than patients with NTG and no headaches, suggesting a subset within this group of patients (59). An abnormally reduced blood flow in the fingers, especially in response to exposure to cold, has also been reported (60,61). Other investigators again found two subsets of COAG: (a) a smaller one with vasospastic finger blood flow measurements and a highly positive correlation between visual field loss and IOP and (b) a larger group with disturbed coagulation and biochemical measurement, suggestive of vascular disease, with no correlation between field and highest IOP (62). A study of peripheral vascular endothelial function in patients with NTG found impaired acetylcholine-induced peripheral endothelium-mediated vasodilation in comparison with healthy age- and sex-matched controls (63), and a polymorphism of the endothelin receptor type A gene has been associated with NTG (64). The bulk of the observations, therefore, suggest that vasospastic events are involved in the mechanism of at least some forms of NTG. (The therapeutic implications of this are discussed at the end of this chapter.)
Hematologic abnormalities reported to be associated with NTG include increased blood and plasma viscosity and hypercoagulability (e.g., increased platelet adhesiveness and euglobulin lysis time) (41,46,65). Other studies, however, have revealed no statistically significant abnormalities in coagulation tests or in vascular or rheological profiles (66,67). Hypercholesterolemia is reported to be higher among patients with NTG (68). Magnetic resonance imaging in patients with NTG has revealed an increased incidence of diffuse cerebral ischemia, which may be further evidence for a vascular etiology (69,70).
There is also some evidence that immune mechanisms may play a role in the mechanism of NTG. In one study, 30% of the patients had one or more immune-related diseases, compared with 8% in a matched group of patients with ocular hypertension (71). Additional support for an immune mechanism includes the increased incidence of paraproteinemia and autoantibodies, such as antirhodopsin antibodies and anti–glutathione-S transferase (a retinal antigen) antibodies, in patients with NTG (72–74). There is also a report of postmortem histopathologic findings in a patient with NTG who had monoclonal gammopathy and serum immunoreactivity to retinal proteins. Immunoglobulin G and A deposition was noted in the ganglion cells and in inner and outer nuclear layers of the retina, and evidence of apoptotic cell death was noted in the ganglion cell and inner nuclear layers of the retina (75).
DIFFERENTIAL DIAGNOSIS OF NORMAL-TENSION GLAUCOMA
The differential diagnosis of NTG is summarized in Table 11.1 and should include wide diurnal IOP fluctuations in which high pressures are occurring at times when they are not being recorded. Other patients may have once had high pressures that caused damage that have since spontaneously normalized. One example of this is pigmentary glaucoma, in which the IOP often improves with increasing age or where a significant exposure to steroid medications in the past was associated with undiagnosed secondary glaucoma–produced damage that stabilized once steroid use was stopped (76). Another situation to distinguish from NTG is the case of advanced optic atrophy and visual field loss, in which even mid-to-low pressures may be associated with or can cause further progressive damage. It is also important to rule out nonglaucomatous causes of disc and field changes (discussed in Chapters 4 and 5 and summarized in Table 11.1), and to consider the clinical scenarios when one may want to order neuroimaging (computed tomography or magnetic resonance imaging of the orbit and chiasm) or other studies (e.g., carotid Doppler, orbital B scan) to rule out these disorders (Table 11.2).
Adjunctive Tests
Numerous tests have been studied to find additional prognostic indicators of COAG. Although none of these has yet been clearly proven to be of clinical value, the physician should be familiar with some of the more frequently discussed adjunctive tests.
Tonography
This procedure and its limitations as a clinical tool in the diagnosis of COAG are discussed in Chapter 3.
Provocative Tests
These tests are largely of historical value, and the interested reader is referred to the fourth edition of this text for a more detailed discussion with references.
Water Provocative Test
Drinking a large quantity of water in a short period of time will generally lead to a rise in the IOP. On the basis of the theory that glaucomatous eyes have a greater pressure response to water drinking, a “provocative” test was developed for the early detection of COAG. The test has little diagnostic value.
Dilatation Provocative Tests
These tests are used primarily in eyes suspected of having potentially occludable anterior chamber angles (see Chapter 12). However, cycloplegics and mydriatics have also been studied with regard to their influence on open-angle forms of glaucoma. These studies have not provided clinically useful diagnostic tests, but a few points can be gleaned from them. Patients with COAG are more likely to have a significant rise in IOP with strong cycloplegics (such as cyclopentolate, 1%; atropine, 1%; homatropine, 5%; or scopolamine, 0.25%) if they are undergoing long-term miotic therapy. The mechanism of the pressure response to strong cycloplegics is thought to include inhibition of the miotic effect and direct inhibitory action on the ciliary muscle. In some cases, the postdilation IOP spike can be marked.
The mydriatic action of cycloplegic–mydriatic agents or a mydriatic, such as phenylephrine, is also thought to be a cause of elevated IOP in some eyes with open anterior chamber angles that are not treated with miotics. This occurs only when an associated shower of pigment is in the anterior chamber. The mechanism is thought to be temporary obstruction of the trabecular meshwork by pigment granules. This occurs predominantly in eyes with the exfoliation syndrome or pigmentary glaucoma but may also occur in some cases of COAG with heavy pigmentation in the anterior chamber angle.
Other Adjunctive Tests
The tendency of patients with COAG, as well as a certain percentage of the general population, to respond to topical steroid therapy with an IOP rise has also been evaluated as a predictive test for COAG. However, steroid responsiveness has been found to correlate poorly with risk for COAG.
PROPOSED MECHANISM OF COAG
Mechanisms of Obstruction and Aqueous Outflow
As with virtually all forms of glaucoma, elevation of the IOP in COAG is due to obstruction of aqueous outflow. However, the precise mechanisms of outflow obstruction in this condition remain poorly understood despite having been studied intensively.
Histopathologic Observations
The most likely source for the eventual explanation of aqueous outflow obstruction in COAG lies in the study of histopathologic material and molecular biology (see Chapters 1 and 10). However, the interpretation of histopathologic findings must consider additional influences, such as age, the secondary effects of prolonged IOP elevation, the alterations that medical and surgical treatment of the glaucoma might have induced, and artifacts created by tissue processing.
Influence of Aqueous Humor
Growing evidence shows that abnormal constituents of the aqueous humor may adversely affect the outflow structures, increasing resistance to outflow. Transforming growth factors (TGFs) are a family of multifunctional polypeptides with several cellular regulatory properties, including inhibition of epithelial cell proliferation, induction of extracellular matrix protein synthesis, and stimulation of mesenchymal cell growth. The aqueous humor of patients with COAG has a significantly greater amount of TGF-β2 than that of healthy individuals (77). Abnormal levels of TGF-β2 in the aqueous of patients with COAG may decrease the cellularity of the trabecular meshwork and promote a buildup of excessive amounts of extracellular matrix materials with subsequent increased resistance to aqueous outflow.
Alterations of the Trabecular Meshwork
Grant demonstrated that the largest proportion of resistance to aqueous outflow in enucleated human eyes could be eliminated by incising the trabecular meshwork (78). Since then, a number of studies have demonstrated that the site of maximum resistance in the meshwork appears to be the juxtacanalicular tissue and inner wall of the Schlemm canal (79). The most characteristic structural change in the juxtacanalicular tissue is an increase in extracellular matrix and an accumulation of “plaque material.” This material derives from thickened sheaths of elastic fibers, although the exact composition remains unknown (77).
Stress-Response Markers
Myocilin, the first gene to be identified as mutated in COAG, appears to be produced in the eye in greater amounts during times of stress. It is present in increased amounts in organ and cell culture experiments after undergoing dexamethasone treatment, oxidative stress, stretching, and treatment with TGF-β (80). Another class of stress-induced proteins studied is heat-shock proteins, such as αB-crystallin. A study of donor eyes with COAG demonstrated differences in staining of two potential stress-response markers, αB-crystallin and myocilin, in trabecular meshwork of glaucomatous eyes (COAG, exfoliative glaucoma, NTG) in comparison with age-matched controls (81). These proteins localized to many more regions of the meshwork and appeared more intense than in healthy eyes, regardless of the type or clinical severity of glaucoma.
Endothelial cells lining the trabeculae appear to be more active in COAG than in normotensive eyes and are reported to show proliferation with foamy degeneration and basement membrane thickening (82). The cellularity of the trabecular meshwork in eyes with COAG is lower than that in nonglaucomatous eyes, but the rate of decline with age is similar in the two groups (83). A reduced frequency of actin filaments (contractile proteins) in trabecular endothelium has also been demonstrated in eyes with COAG (84). Cross-linked actin networks, which alter the function of trabecular cells, were found in higher levels and increased more in response to dexamethasone in glaucoma eyes than in healthy control eyes in tissue culture (85).
Intertrabecular spaces, as might be anticipated from the general thickening of the trabeculae, are narrowed (86). In addition, they may contain red blood cells, pigment, and dense amorphous material (82). Glycosaminoglycans are reported to be more abundant in the meshwork of human eyes with COAG (87,88). However, hyaluronic acid has been shown to be decreased in the trabecular meshwork of eyes with COAG, and the loss of its surface-active properties may influence aqueous outflow resistance (89). Perfusion of cationized ferritin in enucleated eyes of patients with COAG suggests that the outflow obstruction is segmental (90).
Several observers have noted that juxtacanalicular connective tissue just beneath the inner wall endothelium of the Schlemm canal contains a layer of amorphous, osmophilic material (91). This has been described as moderately electron-dense, nonfibrillar material with characteristics of basement membrane and curly collagen and cytochemical properties of chondroitin sulfate protein complex (92,93). However, the concentration of electron-dense materials, although significantly higher than that of a healthy control participant, is not thought to be enough to account for the outflow reduction characteristic of COAG (91). Matrix vesicles, representing extracellular lysosomes, a sheath material from subendothelial elastic-like fibers, the extracellular glycoprotein fibronectin, and elastin have also been found in abnormal amounts in the juxtacanalicular connective tissue of eyes with COAG (94–97). These patients also differ from the healthy population in their collagen binding of plasma fibronectin (98). A study of glycosaminoglycan composition in the juxtacanalicular connective tissue of COAG eyes and age-matched healthy eyes demonstrated that the juxtacanalicular connective tissue in healthy eyes is stratified with hyaluronic acid as the predominant glycosaminoglycan in layers closest to the endothelium of the Schlemm canal (99). In COAG eyes, hyaluronic acid was depleted in all layers within the juxtacanalicular connective tissue, and accumulation of chondroitin sulfate was significantly higher in the juxtacanalicular connective tissue, possibly accounting for the increased outflow resistance in these eyes.
Pores and giant vacuoles are found in the inner wall endothelium of the Schlemm canal in healthy eyes and are thought to be related to aqueous transport. In eyes with COAG, the giant vacuoles have been found in most studies to be decreased or absent. Pore density has also been shown to be reduced and more unevenly distributed in COAG eyes than in healthy eyes (100). In addition, cul-de-sacs, which are described as terminations of aqueous channels, are markedly reduced in eyes with COAG (101).
In a study of the trabecular meshwork and optic nerve from 26 eyes of 14 donors, increasing severity of optic nerve damage (as quantitated by axon counts) was significantly correlated with an increase in the amount of sheath-derived plaque material in the juxtacanalicular connective tissue (102).
Collapse of the Schlemm Canal
Collapse of the Schlemm canal will also increase resistance to aqueous outflow and has been proposed as a mechanism of outflow obstruction in COAG. The collapse represents a bulge of trabecular meshwork into the canal, which might result from alterations in the meshwork or relaxation of the ciliary muscle. In support of this theory, some histopathologic studies have revealed a narrowed Schlemm canal with adhesions between the inner and outer walls (82). A mathematical model of the Schlemm canal, however, tentatively suggests that resistance to aqueous outflow is in the inner wall of the canal and is not caused by a weakening of the trabecular meshwork with a resultant collapse of the Schlemm canal alone (103).
In interpreting the histologic findings in the juxtacanalicular connective tissue and Schlemm canal, it is advisable to take into account a certain amount of segmental variability and to examine at least three quadrants per eye (104).
Alterations of the Intrascleral Channels
Alterations of the intrascleral channels could also be a mechanism of increased resistance to aqueous outflow in COAG. Histopathologic observations have revealed attenuation of the channels, which may be due to a swelling of glycosaminoglycans in the adjacent sclera (105). Krasnov (106) suggested that intrascleral blockage may be the mechanism of outflow obstruction in approximately half of the eyes with COAG. However, this theory was not supported by a study in which removal of tissue overlying the Schlemm canal failed to improve outflow facility until the canal was actually entered (107).
Corticosteroid Sensitivity
As previously noted, there is evidence that patients with COAG are unusually sensitive to corticosteroids and that this steroid sensitivity may be related to the abnormal resistance to aqueous outflow. This discussion first considers the evidence for the increased sensitivity and then looks at theories of how this may influence outflow.
Topical Corticosteroid Response
General population studies have been performed in which a potent topical corticosteroid, such as betamethasone, 0.1%, or dexamethasone, 0.1%, was given three to four times daily for 3 to 6 weeks. These studies found that a substantial proportion of individuals respond with variable degrees of IOP elevation. The studies have differed considerably, however, with regard to many important aspects of this pressure response. For example, the distribution of pressure responses in the general population was found in some studies to be trimodal, with approximately two thirds of participants having a low response (usually defined as an increase in IOP of less than 5 mm Hg), one third showing an intermediate response (increase of 6 to 15 mm Hg), and 4% to 5% having an increase greater than 15 mm Hg (108–110). Another study, however, could not confirm the trimodal concept (111), and when the topical corticosteroid test was repeated in the same population, individuals did not always have the same response each time (112).
COAG populations have more individuals with a high IOP response to topical corticosteroids. The actual reported percentage of high responders, however, varies according to the criteria used to define this group. Reports also differ as to whether patients with ocular hypertension do or do not have a greater incidence of high response than the general population (113,114). The topical corticosteroid response, however, has not been found to be a useful prognostic indicator for COAG (115,116).
Inheritance of the topical corticosteroid response and how this may relate to COAG have been matters of particular controversy. Becker postulated an autosomal recessive mode for the corticosteroid response and suggested that the gene is closely related or identical to that for COAG, which he thought had an autosomal recessive inheritance (108,117). Armaly agreed that the two conditions might be genetically related but proposed a polygenetic inheritance for COAG, with the gene for the topical corticosteroid response being one of the genes involved (110). Results of additional studies were consistent with a genetic basis for the topical corticosteroid response but could confirm neither the recessive mode nor even a relationship to glaucoma (114,118). Still other investigators could not even substantiate that the corticosteroid response was entirely genetic. A twin-heritability study of monozygotic and like-sex dizygotic twins revealed a low estimate of heritability that did not support a predominant role of inheritance in the response to corticosteroids and suggested that nongenetic factors play the major role (111,119–121). A study that further confuses the role of steroids in COAG found that eyes with unilateral angle-closure glaucoma or angle recession also respond to topical corticosteroids with a higher pressure rise in the involved eye than in the fellow eye, which had not had angle closure or trauma (122,123). These observations suggest that topical corticosteroid responsiveness is multifactorial.
Relationship of Intraocular Pressure to Corticosteroid Sensitivity
Investigators have tried to explain if or why patients with COAG are unusually sensitive to corticosteroids.
Hypothalamic–Pituitary–Adrenal Axis Theory
An abnormal response of the hypothalamic–pituitary–adrenal axis in patients with COAG, and possibly in other forms of glaucoma, may be related to alterations in aqueous humor dynamics in response to corticosteroids (124,125).
Cyclic–Adenosine Monophosphate Theory
It may be that corticosteroids influence the IOP by altering cyclic–adenosine monophosphate. Corticosteroids have a permissive effect on the β-adrenergic stimulation of adenyl cyclase, the enzyme responsible for the synthesis of cyclic–adenosine monophosphate (126). How this relates to aqueous humor dynamics is uncertain, although patients with COAG and high topical steroid responders appear to be unusually sensitive to cyclic–adenosine monophosphate.
Glycosaminoglycans Theory
It has also been proposed that IOP elevation associated with corticosteroid sensitivity may be related to glycosaminoglycans in the trabecular meshwork (127). When polymerized, glycosaminoglycans become hydrated, swell, and obstruct aqueous outflow. Catabolic enzymes, released from lysosomes in the trabecular cells, depolymerize the glycosaminoglycans. Corticosteroids stabilize the lysosome membrane, preventing release of these enzymes and thereby increasing the polymerized form of glycosaminoglycans and the resistance to aqueous outflow.
Phagocytosis Theory
The effect of steroids on IOP may be related to the phagocytic activity of endothelial cells lining the trabecular meshwork. These cells are normally phagocytic, and they may function to “clean” the aqueous of debris before it reaches the inner wall endothelium of the Schlemm canal. Failure to do so might result in a buildup of material that could account for the amorphous layer in the juxtacanalicular connective tissue (as previously described). Corticosteroids suppress phagocytosis, and it may be that the trabecular endothelium in patients with COAG is unusually sensitive, even to endogenous corticosteroids (128).
Mechanism of Optic Neuropathy
Histopathologic Observations
Axon loss in eyes with COAG has been reported to be associated with increasing connective tissue in the septa and surrounding the central retinal vessels, including increased amounts of types IV and VI collagen (129). The total number of capillaries and the density of capillaries decreased with loss of axons. Arteriosclerotic changes were more common in glaucomatous eyes than in age-matched control eyes.
Immunologic Studies
A number of reports suggest an immunoregulatory mechanism in the pathogenesis of COAG, at the level of the meshwork, ganglion cell bodies and optic nerve axons, retinal vessels, and lamina cribrosa. The roles of the immune system in glaucoma have been described as either neuroprotective or neurodestructive. It has been proposed that a critical balance between beneficial protective immunity and harmful sequelae of autoimmune neurodegenerative injury (such as heat-shock proteins) determines the ultimate fate of retinal ganglion cells in response to various stressors in patients with glaucoma. The Canadian Glaucoma Study reported that an elevated anticardiolipin antibody is associated with progression of COAG (130). Anticardiolipin antibody is one of the antiphospholipid antibodies found in elevated levels in patients with acquired thrombotic syndromes (131,132).
Blood Flow
Abnormalities of blood flow to the posterior segment of the eye in COAG have been shown by using color Doppler imaging, fluorescein angiography, laser Doppler flowmetry, and pulsatile ocular blood flow measurements (133–141). Rheological studies have also demonstrated differences in red cell aggregability, increased plasma viscosity, and activation of the clotting system in patients with COAG compared with controls (65,142,143). Altered autoregulation of blood flow in the optic nerve and retinal circulation has also been demonstrated (144,145).
Changes in the retrobulbar hemodynamics also appear to occur with age. Color Doppler imaging analysis of the ophthalmic, central retinal, and nasal and temporal posterior ciliary arteries in healthy men and women demonstrated age-related alterations in hemodynamics, similar to those seen in patients with glaucoma, suggesting that these age-related changes may contribute to an increased risk for glaucoma (146).
There is also evidence that the choroidal circulation is compromised in COAG (147,148), which is supported by electroretinographic data demonstrating outer retinal damage in eyes with glaucoma (149).
Apoptotic Susceptibility of Ganglion Cells
Ganglion cells appear to die by apoptosis in experimental glaucoma (150). This may relate to a multiplicity of factors (Fig. 11.1). Clinically, some evidence is related to excitotoxic cell death from accumulation of glutamate and an imbalance of proteases that modulate the extracellular matrix milieu in the retina (151,152).
Figure 11.1 Diverse insults can lead to retinal ganglion cell death. These include IOP-related and non–IOP-related factors. (Reproduced from Libby RT et al. Complex genetics of glaucoma susceptibility. Annu Rev Genomics Hum Genet. 2005;6:15–44, with permission).
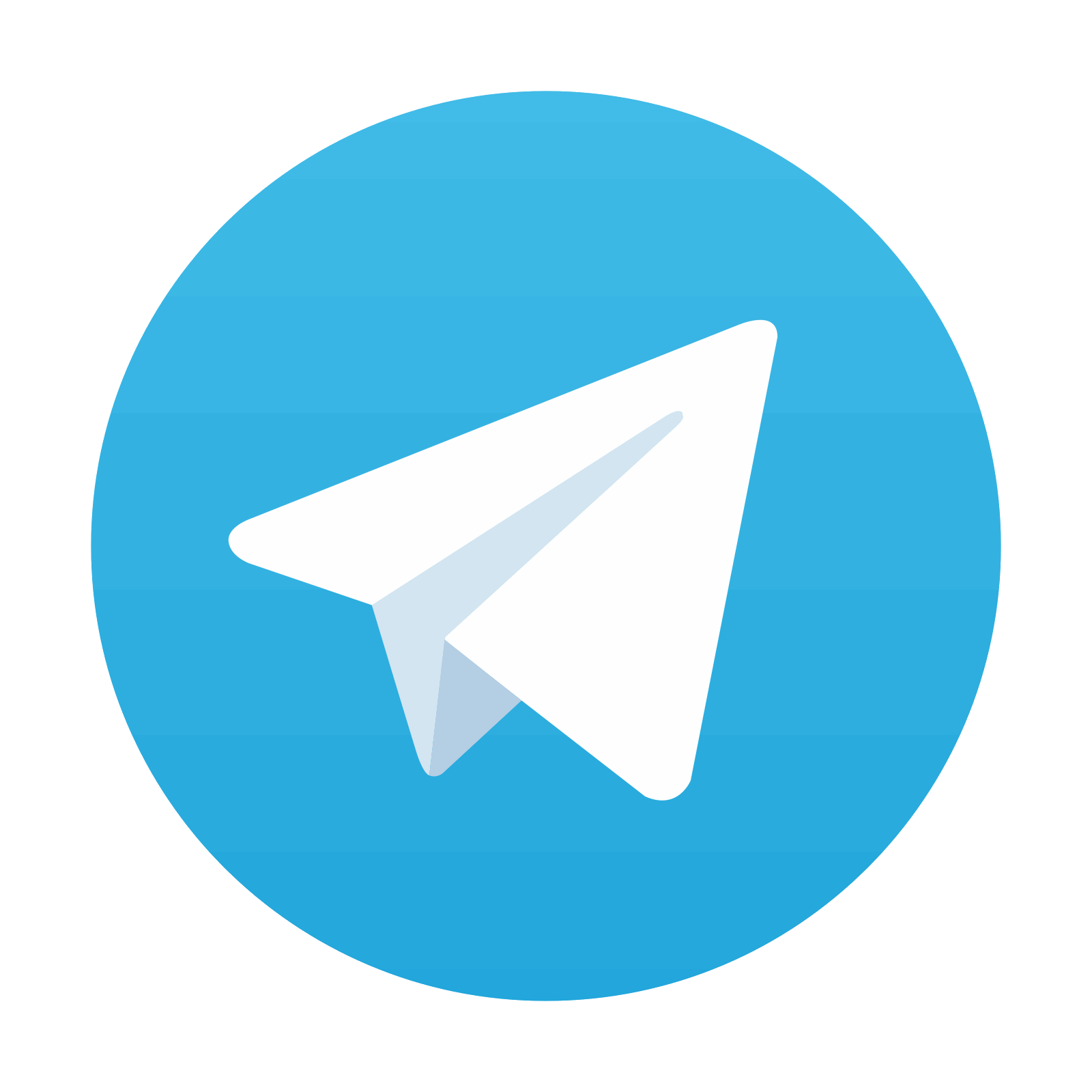
Stay updated, free articles. Join our Telegram channel

Full access? Get Clinical Tree
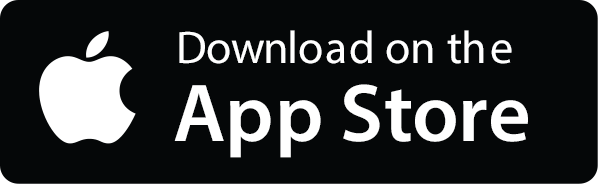
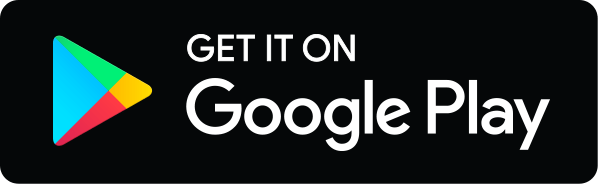