sustentacular cells (type II). These clusters are interspersed by a web of blood vessels. The equivalent structures seen in paragangliomas are termed Zellballen, which retain part of this structural arrangement. The sustentacular cells do not secrete catecholamines and are similar in nature to the Schwann cells enveloping autonomic ganglia. The chief cells have abundant secretory granules, a characteristic of secreting cells. The biochemistry of paraganglia chief cells is characterized by catecholamine production (7). This sequence is characterized by enzymatic conversion of tyrosine to dopamine, norepinephrine, and epinephrine. Extra-adrenal paraganglia lack methyltransferase that is required for the conversion of norepinephrine to epinephrine. The metabolic breakdown of catecholamines to metanephrine (from epinephrine) and normetanephrine (from norepinephrine) as well as vanillylmandelic acid (VMA) can be detected in the urine. Actively secreting paragangliomas can be thus detected with appropriate urine and serum analysis (Fig. 127.4).
TABLE 127.1 CLASSIFICATION OF VASCULAR TUMORS OF THE HEAD AND NECK | |||||||||||||||||||||||||||||||||||||||||||||||||||||||||||||||
---|---|---|---|---|---|---|---|---|---|---|---|---|---|---|---|---|---|---|---|---|---|---|---|---|---|---|---|---|---|---|---|---|---|---|---|---|---|---|---|---|---|---|---|---|---|---|---|---|---|---|---|---|---|---|---|---|---|---|---|---|---|---|---|
|
living, a ninefold increase for those living between 2 and 3 km and 12-fold increase for those living between 3 and 4.5 km above sea level. Patients forming these tumors at higher altitude show a lower rate of bilaterality and a lesser degree of familial history of paragangliomas (12,13,14).
SDHD (cybS, 12 kDa, 159 amino acids) subunits are hydrophobic and provide membrane anchor and the binding site for ubiquinone. SDHA (flavoprotein, 70 kDa, 664 amino acids) and SDHB (iron-sulfur protein, 27 kDa, 280 amino acids) are hydrophilic with the former involved in substrate binding and oxidation and the latter in electron transfer. Both the SDHB (35.4 kb, 8 exons) and the SDHC (50.3 kb, 6 exons) genes are located on chromosome 1, the short and long arm respectively. The SDHD gene located on 11q23.1 spans 8.9 kb and contains 4 exons while SDHA lies on the short arm of chromosome 5 (5p15) and is composed of 15 exons spread in a genomic region of 38.4 kb. While homozygote germline mutations affecting the SDHA gene cause Leigh syndrome, a subacute necrotizing encephalomyelopathy during infancy, SDHD, SDHB, and SDHC heterozygous mutations cause a genetic predisposition to head and neck paragangliomas and adrenal/extra-adrenal pheochromocytomas (23). This inherited tumorigenic predisposition is transmitted in an autosomal dominant fashion with age-dependent and incomplete penetrance. However, for SDHD located on chromosome 11q, a parent-of-origin effect is apparent as the disease is manifest almost exclusively when the mutation is transmitted from the father. A maternal imprinting has therefore been postulated but despite the pattern of inheritance, SDHD shows bi-allelic expression in normal tissues and neural crest-derived tissues (12,18).
Patients with PGL develop tumors at a younger age than sporadic cases
Other tumor syndromes: Neurofibromatosis type 1, MEN type 2 and von Hippel-Lindau predispose to paragangliomas
In PGL 1, 2, and 3, the genetic transmission is autosomal dominant, with highly variable expressivity and reduced penetrance. Genomic imprinting is seen: the paternally transmitted genes lead to tumor development and the maternally transmitted gene gives carrier status without developing tumors
PGL1 and PGL4 show multicentricity and pheochromocytomas
PGL4 shows a marked increase in malignant paragangliomas
PGL3 shows exclusively benign paragangliomas with no multifocality and no association to pheochromocytomas.
and their pattern of growth follows the pathways of least resistance in the temporal bone and surrounding skull base structures. In their later stages, both types produce similar symptoms including cranial nerve deficits, while in the early stages tympanic and jugular paragangliomas differ in presentation.
TABLE 127.2 SHAMBLIN CLASSIFICATION OF CAROTID BODY TUMORS | ||||||
---|---|---|---|---|---|---|
|
on detailed patterns of progressive disease extension, and the Glasscock-Jackson classification system, which treats tympanic and jugular paragangliomas differently (15,28) (Tables 127.3 and 127.4).
TABLE 127.3 FISCH CLASSIFICATION OF JUGULOTYMPANIC PARAGANGLIOMAS | ||||||||||||||||||||||||||
---|---|---|---|---|---|---|---|---|---|---|---|---|---|---|---|---|---|---|---|---|---|---|---|---|---|---|
|
TABLE 127.4 GLASSCOCK-JACKSON CLASSIFICATION OF JUGULOTYMPANIC PARAGANGLIOMAS | ||||||||||||||||||||
---|---|---|---|---|---|---|---|---|---|---|---|---|---|---|---|---|---|---|---|---|
|
cavity. Characteristic patterns of bone destruction occur with jugulotympanic paragangliomas. These include erosion of the jugulotympanic spine, enlargement of the jugular foramen with bone destruction, and involvement of the middle ear and mastoid (31,32). Multiplanar reconstruction of high-resolution CT scans in the axial, sagittal, and coronal planes is of great assistance in the planning of operative management of these tumors. Middle ear and/or eustachian tube involvement will create postobstructive fluid accumulation in the aerated spaces of the temporal bone, including the mastoid (Fig. 127.8).
diagnosis of head and neck paragangliomas but is of paramount importance in preoperative planning for resection of these tumors. Angiography is essential in providing a detailed map of tumor blood supply and venous drainage, demonstrating the tumor flow dynamics and detailing the general vascular anatomy of the head and neck and intracranial space. Four-vessel cerebral angiography allows for qualitative and quantitative blood flow studies of the cerebral circulation. Preoperative preparation through superselective embolization of the feeding arterial supply to the tumor decreases the risk of intraoperative blood loss.
or where a planned internal carotid resection is contemplated. An angiographic balloon occlusion test involves the threading of a transfemoral catheter to the internal carotid and involves temporarily occluding the internal carotid artery, usually at the carotid siphon within the cavernous sinus to determine whether there will be neurologic deficit (9,11). Several modalities for neurologic monitoring during balloon occlusion testing of the internal carotid artery are available. In order of decreasing sensitivity, they are clinical neurologic examination, electroencephalography (EEG), and quantitative blood flow examination through xenon CT concurrent examination. Xenon CT angiography is a precise quantitative study with the best sensitivity but is cumbersome and rarely available (37,38) (Fig. 127.11).
for assessing adequate contralateral cerebral circulation. Xenon CT scanning with concurrent balloon occlusion is a quantitative analysis of cerebral flow and is the most precise method of cerebral circulation evaluation, though it is cumbersome and not readily available (37,38). Temporary balloon occlusion of the carotid with monitoring of the clinical neurologic examination in the presence of a patent circle of Willis is easier to perform and also very reliable. EEG monitoring can be performed during balloon occlusion as well as hypotensive challenge during the period of balloon occlusion. Approximately 93% of patients can tolerate sacrifice of the carotid artery based on angiographic evaluation. It should be noted that intraoperative conditions may be different in terms of cerebral delivery of oxygen than those in the angiographic suite (11). Tolerance of temporary internal carotid occlusion does not predictably avoid the possibility of a delayed postoperative cerebrovascular ischemic event. The venous outflow of the ipsilateral and contralateral sigmoid and jugular systems should be noted, especially in jugulotympanic paragangliomas. Since there are anatomic variants, a contralateral hypoplastic or absent jugular system would be a contraindication for surgery as this would raise the possibility of a postoperative venous stroke unacceptably (42,43,44) (Fig. 127.13).
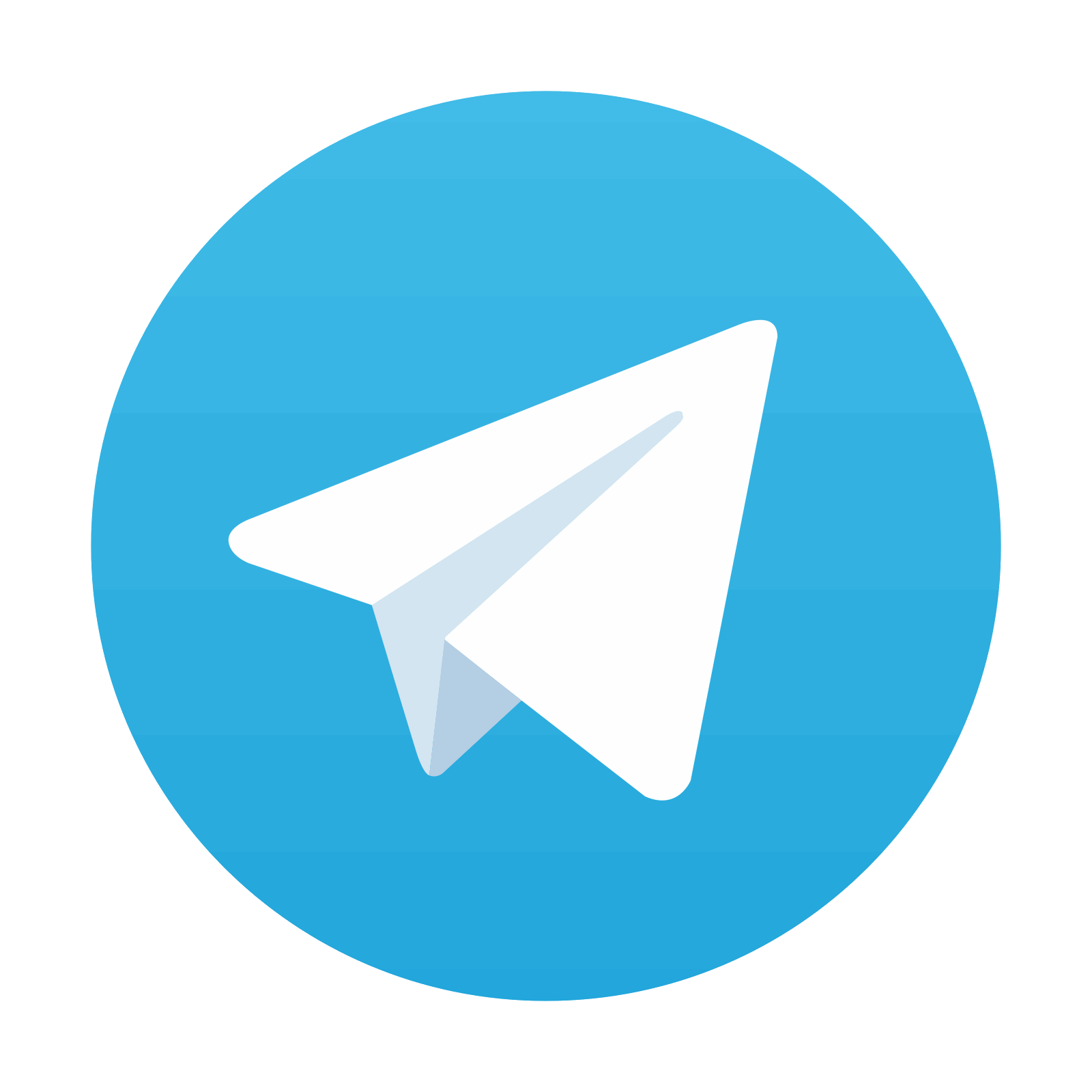
Stay updated, free articles. Join our Telegram channel

Full access? Get Clinical Tree
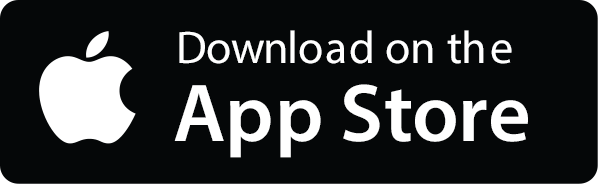
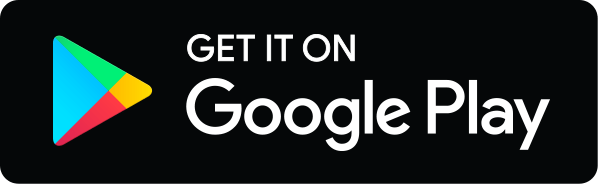