Purpose
To investigate the retinal vascular findings and associated cystoid edema in the central macula of eyes with retinal vein occlusion using volume-rendered angiographic and structural optical coherence tomography.
Study Design
Observational case series.
Methods
In this retrospective study 12 eyes of 12 consecutive patients, 3 with branch and 9 with central retinal vein occlusion, were imaged in 27 sessions with optical coherence tomography (OCT) using split-spectrum amplitude decorrelation. The structural OCT data were segmented for cystoid spaces and integrated into the angiographic data for subsequent volume rendering. The inner and deep vascular plexus were analyzed in relation to cystoid macular edema with retention of depth information.
Results
Retinal vascular flow abnormalities were demonstrated by flow voids with abnormal vascular morphology in the inner vascular layer and varying flow loss in the deep vascular plexus. Areas of cystoid edema were associated with topographically co-localizing flow voids in the deep vascular layer. Treatment with intravitreous anti–vascular endothelial growth factor injections resulted in resolution of edema but no change in flow patterns in either the inner or deep plexus. Recurrence of edema happened in the same areas of altered inner and absent deep vascular plexus flow signal.
Conclusions
Cystoid macular edema in retinal vein occlusion occurred in relation to altered inner plexus and absent deep vascular plexus flow. This pattern of cystoid fluid accumulation is similar to that seen in diabetic retinopathy and may represent an important underlying pathophysiologic foundation for cystoid macular edema in retinal vascular diseases.
A common complication of retinal vein occlusion is the accumulation of edema fluid in cystoid spaces within the macula. Intravitreous injection of either corticosteroids or agents directed against vascular endothelial growth factor (VEGF) is associated with a decrease or resolution of cystoid macular edema, but this effect is transitory. The edema in branch retinal vein occlusion occurs within the distribution of the vein, while the edema in central retinal vein occlusion typically involves the central macula with variable extension to more peripheral parts of the macula.
The abnormalities induced by veno-occlusive disease are typically evaluated by fluorescein angiography, but fluorescein angiography has not provided information as to why some areas are involved with cystoid macular edema while neighboring areas are not, why the cystoid edema preferentially occurs in the macular region, or why cystoid edema returns in retinal veno-occlusive disease. Optical coherence tomography (OCT) angiography is a method to visualize blood flow, and consequently blood vessels containing the flow, by using motion contrast techniques. Areas of stationary tissue produce little change in reflection over time, while moving elements produce local changes in reflectivity. OCT angiography of the inner vascular plexus of the eye with OCT angiography produces images similar to those obtained by fluorescein angiography. However, the deep vascular plexus, an interlocking meshwork of curvilinear capillaries bracketing the inner nuclear layer, is not imaged with fluorescein angiography, whereas it is readily seen with OCT angiography.
A common way to visualize the flow data obtained by OCT angiography is to use en face imaging. In this method the flow data are segmented according to the anatomy of the retinal layers and each slab of information is flattened for viewing. In eyes with edema, segmentation is difficult because the normal anatomy is distended and cystoid spaces appear. These segmentations are frequently incorrect in the layers being evaluated and the segmentation thickness often does not change in thickness even though the retinal layers do, as in the case of edema. In addition, en face imaging causes loss of depth information within imaged layers when the segmented region is collapsed to a 2-dimensional en face image. Cystoid spaces are 3-dimensional structures that cannot be placed into a 2-dimensional surface with accuracy or with retention of correct depth information. An alternate approach is to use volume rendering techniques, which do not use segmentation and do not lose depth interrelationships in the data prior to visualization. Since the structural OCT is used to generate angiographic OCT images, any architectural feature segmented from the structural OCT, such as cystoid spaces, can be directly integrated into the volume-rendered OCT angiographic image. Volume rendering has been used to visualize computed tomography or magnetic resonance imaging data for decades. Using volume-rendered OCT angiography in eyes with diabetic retinopathy, cystoid macular edema was seen to occur in areas that had an abnormal inner vascular plexus morphology associated with areas of absent flow signal in the deep vascular plexus. Given that it is possible that deep vascular plexus abnormalities also are important in veno-occlusive disease, eyes with branch and central retinal vein occlusion were evaluated with volume-rendering OCT angiography.
Methods
The patients evaluated in the study were seen in a community-based retinal referral practice of the author. This retrospective study was approved by the Western Institutional Review Board and complied with the Health Insurance Portability and Accountability Act of 1996. The patients imaged had veno-occlusive disease as established by previous examination. Eyes with media opacities, glaucoma, or history of tractional or rhegmatogenous macular detachment were excluded, as were eyes with a signal strength of 55 or less as supplied by the Optovue instrument or those who could not maintain fixation during the scanning process.
Optical Coherence Tomography Angiography
The instrument used for OCT angiography (OCTA) images is based on the Optovue RTVue XR Avanti (Optovue, Inc, Freemont, California, USA) to obtain amplitude decorrelation angiography images. This instrument has an A-scan rate of 70 000 scans per second, using a light source centered on 840 nm and a bandwidth of 45 nm. Each OCTA 3 × 3-mm volume contains 304 × 304 A-scans with 2 consecutive B-scans captured at each fixed position before proceeding to the next sampling location. Split-spectrum amplitude-decorrelation angiography was used to extract the OCT angiography information. Each OCTA volume is acquired in about 3 seconds and 2 orthogonal OCTA volumes were acquired in order to perform motion correction to minimize motion artifacts arising from microsaccades and fixation changes. The scanning procedure used in this study is identical to that used for typical en face imaging; the novel differences are how the data are evaluated after acquisition.
Extraction of Angiographic and Structural Information
The image processing techniques have previously been described. The choriocapillaris layer was selected in the Optovue ReVue software (version 2014.2.0.93, Optovue, Inc). Successive slabs 8–9 μm thick above the curve through the thickness of the retina were exported from the software; in addition to saving the angiographic image from that slice, the corresponding structural OCT image is also saved. The initialization file was modified to prevent the overprinting of the “Angio OCT” imprint on the resultant saved images. In the case of pseudocolor encoding of layers, the vascular layers were overlaid with color prior to volume rendering. The structural OCT images were segmented for a region of interest by simple thresholding techniques to select areas devoid of reflection corresponding to cystoid spaces, with cross-correlation to the fundus photograph to ensure no co-localizing intraretinal hemorrhages were present. Each plane of the image corresponded to 1 plane of angiographic data and 1 for the structural data. These 2 planes could be merged for use in the volume rendering. In actual practice, it was not necessary to actually merge the corresponding angiographic and structural data groups on a plane-by-plane basis if the vertical interplane resolution was reduced by a factor of 2. The OCT angiographic data were imported into the program MIPAV (Medical Image Processing, Analysis, and Visualization, version 7.2; US National Institutes of Health, Bethesda, Maryland, USA) for volume rendering. The opacity transfer and the look-up table functions were adjusted to improve visualization of the vasculature. The images shown are screen captures of the volume-rendered images.
Vascular Layers
The inner retinal vascular layer supplies the ganglion cell layer. The deep plexus is located external to the inner plexus and is formed by vessels that straddle the inner nuclear layer. These layers can be recognized in volume-rendered images based on their size and branching characteristics. The inner vascular plexus has a fractal branching pattern with increasing numbers of decreasing-diameter vessels, while the deep plexus is composed of an interconnected network of arciform small-diameter vessels. To aid in visual separation of the layers the inner vascular layer was colored blue and the deep layer red. In effect this is a segmentation, and was performed in the images shown in this report. In examining the images with a computer the images can be rotated in space, and using color coding is often not needed. An abnormality in either layer was thought to be present if there was a defect, excluding the expected foveal avascular zone greater than 250 μm in greatest linear dimension.
Results
There were 12 eyes of 12 patients with a mean age of 67.1 (range, 48–86) years. Branch retinal vein occlusion was present in 3 and a central retinal vein occlusion was seen in 9. The eyes were imaged a total of 27 times at stages during follow-up in relation to treatment with intravitreous injections of anti-VEGF agents. All patients were eventually treated; 2 were imaged starting prior to treatment while the remaining patients were treated for a mean of 65.5 (range, 6–110) months. The mean total anti-VEGF injections received was 28.7 per eye at the last OCT angiographic examination.
Despite the inclusion of both branch and central retinal vein occlusion, there were consistent findings seen in all eyes ( Figures 1–9 ). En face images of vascular layers, particularly the deep vascular plexus, were fraught with segmentation defects for both branch and central retinal vein occlusions and are shown in Figures 1, 4 , and 6 (along with corresponding volume-rendered angiographic and structural optical coherence tomography images in Figures 2, 5 , and 7 ). There were varying alterations of the inner plexus in the distribution of the affected vein, localized in branch ( Figures 1–3 ) and more generalized in central retinal vein occlusion ( Figures 4–9 ). There were regional areas where flow signal was not visualized in the deep plexus. Scattered small areas of signal discontinuity were seen under relatively normal areas of inner plexus morphology, while larger regions of severely decreased or absent flow signal were subjacent to abnormalities seen in the inner plexus of vessels. Regions of absent inner vascular plexus flow signal invariably were associated with absent deep plexus flow signal. Integration of the cystoid spaces as derived from the corresponding structural OCT showed that cystoid macular edema occurred in regions where the deep plexus demonstrated flow voids. In branch retinal vein occlusions these occurred under regions of compromised inner plexus flow ( Figures 2 and 3 ). These areas, in turn, only were seen in the distribution of the occluded vein. One eye had larger areas of inner plexus nonperfusion in the inner retina ( Figure 3 ) but did not have cystoid edema in the more ischemic areas. The cystoid edema was seen to accumulate in bordering areas where the inner plexus flow was disturbed but present, while the outer plexus flow signal was absent.
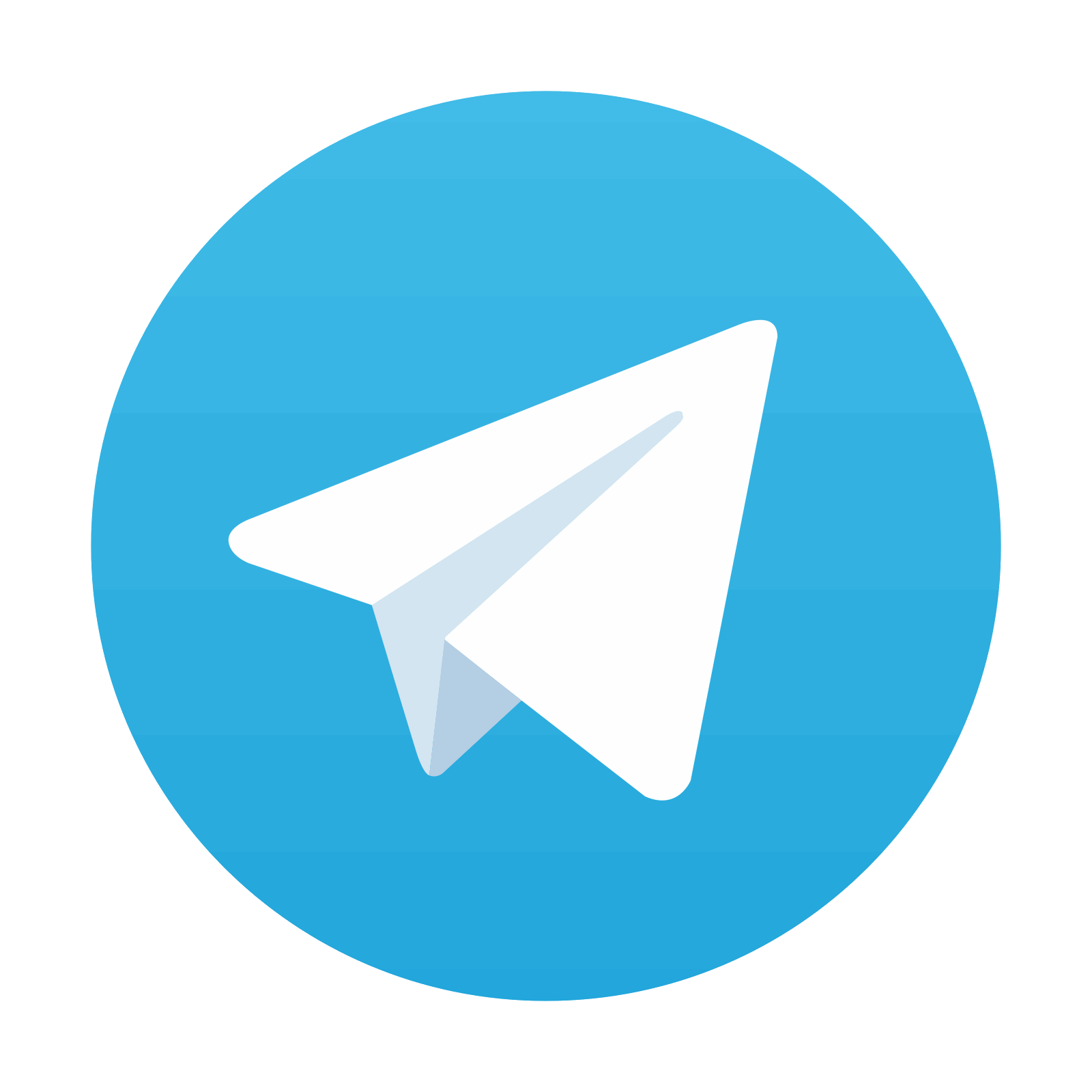
Stay updated, free articles. Join our Telegram channel

Full access? Get Clinical Tree
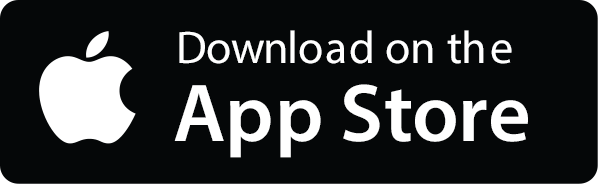
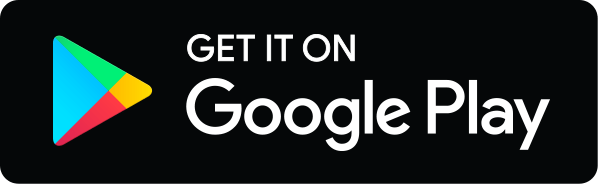
