where L is vocal fold length, x0 is the neutral glottal width, B is the damping coefficient, c is the mucosal wave velocity, T is the vocal fold thickness, and ρ is the density of air. In this model, the damping coefficient correlates to the stiffness of the vocal folds. For example, vocal fold scarring would increase the stiffness, thus increasing the damping coefficient and PTF [10]. A similar phenomenon is observed in the setting of benign mass lesions such as polyps or nodules [7]. Changes in pre-phonatory glottal width, as in the setting of glottic insufficiency, also affect PTF. An excised larynx experiment was conducted that showed PTF was more sensitive than phonation threshold pressure (PTP) to changes in glottal width [11].
MFR describes airflow during sustained phonation. Both PTF and MFR have been shown to differentiate between normal and pathological voice productions [10]. MFR is also one of the two parameters used to measure derived laryngeal resistance (RL), where RL is equal to subglottal pressure (Ps) divided by MFR.
Pressure

Resistance
In general, flow resistance is defined as the ratio of pressure to flow and is often measured in centimeters of water per liter per minute (cmH2O/L/min). Ideally, this ratio would remain constant regardless of pressure and flow, thus providing an invariant characteristic of the airway [14]. Airflow resistance provides information concerning the glottal size, configuration, and biomechanical properties [15]. Glottal resistance (Rg) is calculated using the pressure across the glottis and the airflow through the glottis [16]. Laryngeal resistance (RL) is similar but is calculated from subglottal pressure (Ps) and translaryngeal airflow [17]. In a comparison of Rg measurements, Netsell et al. found that females typically have higher resistances than males. As resistance depends on the size of the airway, this was attributed to females having smaller larynges [18]. However, resistance is dependent on other factors including degree of vocal fold adduction, roundness of glottal entry and exit, [19] and the speed of air particles moving through the glottis [20].
Power

Pathologies that increase the mass or stiffness of the vocal folds or increase the glottal width will increase the power required to start and maintain phonation [21]. In comparison of PTP, PTF, and PTW, PTW had the greatest ability to differentiate patients with mass lesions and vocal fold immobility compared to healthy subjects [13, 22].
Vocal Efficiency

Clinical Assessment Methods
The development of methods to accurately assess and quantify these aerodynamic parameters is a current area of research. Measurement of subglottal pressure is of particular interest as it describes the driving force for voice production. Previously utilized methods for measuring subglottal pressures include a transtracheal pressure transducer [17, 27]. While this was accurate, it is invasive and not feasible for routine assessment. Two approaches have been developed for noninvasive indirect subglottal pressure measurement, labial interruption and mechanical interruption.
The first noninvasive method of subglottal pressure assessment was introduced in 1981 by Smitheran and Hixon. Their method was based on the assumption that oral and subglottal pressures reach an equilibrium during the production of a stop-plosive (/pα/) [17]. The validity of this measurement was confirmed through the use of a trans-nasal transducer by Löfqvist et al. [27]. The labial interruption task has been adapted to also measure PTP. To do this, the subject phonates as quietly as possible while producing the stop-plosives [22]. Labial interruption has been shown to be a reliable assessment method for both adults [28] and children [29]; however, it can be difficult for the subject to master, leading to higher intrasubject variability [30].

Schematic of the airflow interruption system. The subject produces a sustained vowel into a mask or mouthpiece, and pressure is measured within the device during interruption of airflow by a balloon valve. (From Jiang et al. [37], with permission)
Compared to subglottal pressure, airflow measurement is simpler. An assumption is made that no air loss occurs into the tissues of the vocal tract and thus, airflow exiting the mouth is equal to that passing through the glottis. Devices such as the Rothenberg mask and other flow measurement methods work off the Ohm’s law analog mentioned previously. By measuring the pressure difference across a known resistance, flow can be calculated [33].
Current Clinical Equipment
The Phonatory Aerodynamic System (PAS) is currently used for clinical voice assessment. PAS model 6600 was developed in 2006 by the KayPENTAX Corp. to replace the Aerophone II model 6800 created by Kay Elemetrics Corp. The PAS can simultaneously capture sound intensity, intraoral pressure, airflow rate, and fundamental frequency and has an auxiliary port to allow for the collection of electroglottography. It also includes protocols for common phonatory measurements including vital capacity, air pressure screening, comfortable sustained phonation, vocal efficiency, and running speech analysis as well as normative data for pediatric and adult subjects to assist clinicians with interpreting results [34, 35]. The labial interruption technique is used to assess subglottal pressure with the PAS. In studies that examined the test-retest reliability of the PAS, the parameters of glottal power, efficiency, and resistance had substantial coefficients of variation in both men and women [36].
Mechanical Interruption Methods

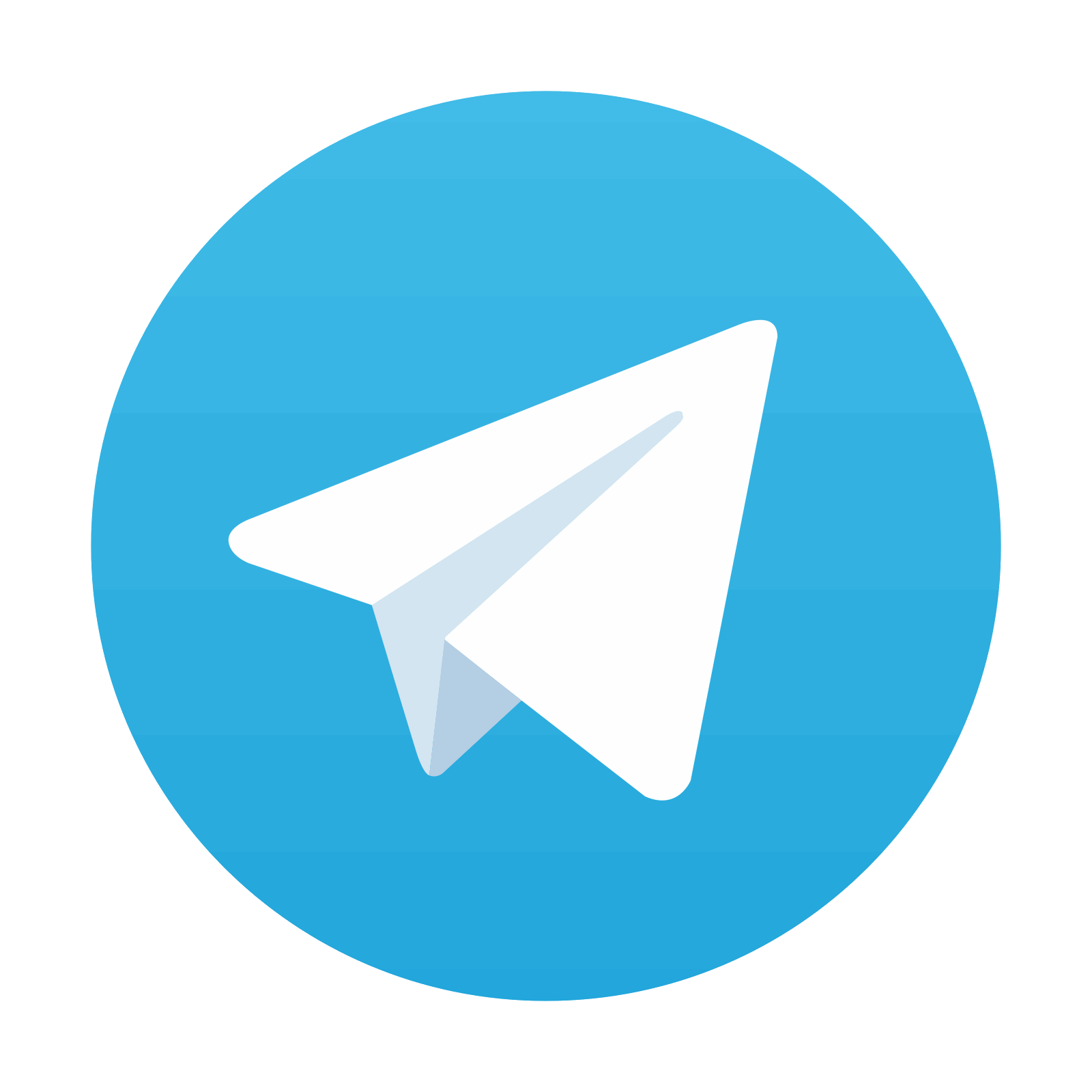
Stay updated, free articles. Join our Telegram channel

Full access? Get Clinical Tree
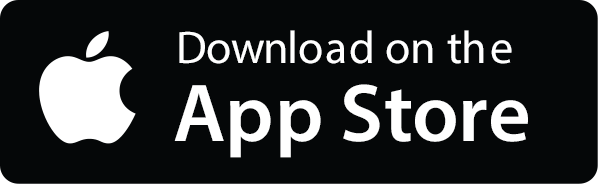
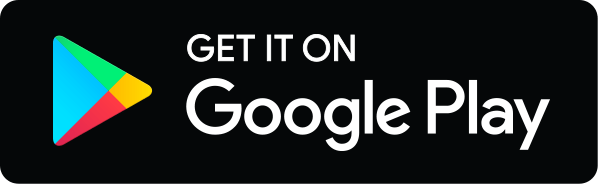