Visual Fields and Their Relationship to the Optic Nerve
Joshua D. Stein, MD, MS and R. Rand Allingham, MD
The 2 most important factors to consider when determining whether a patient has glaucoma and the severity of disease are the structural appearance of the optic nerve and the presence of functional damage as detected by visual field testing. Evaluation of the optic disc for glaucomatous damage was covered in detail in Chapter 8. This chapter will describe the methods of checking for functional damage, including the different types of visual field tests available, characteristics of normal and glaucomatous visual field loss, and how to correlate structural damage to the optic nerve or nerve fiber layer with functional damage detected on visual field testing. Correlating the information obtained from careful examination of the optic nerve with functional damage detected by perimetric testing is far superior to relying on either piece of information alone.
VISUAL FIELD
Normal Visual Field
The visual field can be defined as that area of vision seen with eyes open. The dimensions of the field of vision are defined relative to central fixation. The normal visual field extends approximately 60 degrees nasal and superior, 70 degrees inferior, and 90 to 100 degrees temporal to fixation. The blind spot in the field of vision corresponds to the area defined by the optic nerve head (ONH)and is typically located 15 degrees temporal to fixation. It varies in size according to optic disc size and extent of peripapillary retinochoroidal atrophy.
Traquair1 described the visual field in 3 dimensions, as “an island of vision surrounded by a sea of blindness.” This important concept reflects the addition of retinal sensitivity to the 2-dimensional area of visual field. The most sensitive region in the normal visual field is the portion of the field detected by the fovea. The retinal sensitivity drops rapidly within 15 degrees of the foveal peak. From this point, there is a gradual reduction in retinal sensitivity that extends to the periphery where it abruptly drops off. In cross-section, the blind spot appears on Traquair’s island of vision as a well with steep sides.
The size and contour of the visual field can be influenced by a multitude of factors. In addition to glaucomatous or neuro-ophthalmic conditions, other factors that can affect visual field performance include facial structure, eyelid anatomy, pupil size, ocular media (clarity of the cornea, lens, and vitreous), refractive error, testing artifact, as well as patient experience, cognitive status, reliability, and fatigue.
Glaucomatous Visual Field
Glaucomatous optic nerve damage produces characteristic changes in the contour and shape of the visual field.
Generalized depression of the visual field is considered the most common change noted in glaucoma.2 However, this finding is nonspecific and can be produced by a variety of other conditions as well, including cataract, corneal disease, fatigue, and incorrect refractive correction, among others.
Nerve fiber bundle defects as a result of glaucomatous optic nerve damage produce visual field changes that are considered to be characteristic of glaucoma. These changes reflect retinal and optic nerve anatomy. Retinal nerve fibers radiate from the ONH and are distributed in an arcuate manner around the foveal region (Figure 11-1). Therefore, glaucomatous damage to the ONH produces visual field defects in the region subserved by the affected nerve fibers. The visual field defects produced from retinal nerve fiber bundle defects include localized paracentral scotomas, arcuate defects, nasal steps, and temporal defects (Figure 11-2). The most common location of visual field defects occur within an arcuate area commonly referred to as Bjerrum’s area (or region), which includes that portion of the arcuate region that extends from the blind spot to the median raphe, where it extends 10 to 20 degrees nasally from fixation.3
Figure 11-1. Superotemporal and inferotemporal retinal nerve fibers fol low an arcuate path around the fovea, meeting at the median raphe. Damage to this portion of the nerve fiber layer is responsible for arcuate field defects seen in glaucoma. (Reprinted with permission from Heijl A, Patella VM. Essential Perimetry: The Field Analyzer Primer. 3rd ed. Dublin, CA: Carl Zeiss Meditec; 2002:71.)
Paracentral scotomas are discrete defects in the visual field caused by localized reduction in retinal sensitivity. These may be relative or absolute and are frequently found in Bjerrum’s region.4 Initially, these may appear intermittently as one or more shallow depressions detected sporadically on repeat examination prior to developing into permanent visual field defects.5,6 With progression, paracentral scotomas become deeper and larger and may gradually coalesce, forming an arcuate or Bjerrum scotoma.
A nasal step occurs when there is a difference in the rate or location of visual field loss above and below the horizontal raphe nasal to fixation. This produces a distinct step that may occur along any, but not necessarily all, isopters.7,8
Temporal visual field defects are produced by damage to the nasal neuroretinal rim. Nerve fibers radiate without deviation from the nasal optic nerve; therefore, wedge defects develop. Temporal defects are usually found as a late manifestation of glaucomatous visual field loss.9
Figure 11-2. Arcuate visual field defects. (A) Bjerrum’s region extends from the blind spot to the median raphe in an arcuate path that encompasses 10 to 20 degrees nasally from fixation. (B) Seidel scotoma. (C) Paracentral scotomas. (D) Arcuate or Bjerrum scotoma. (E) Double arcuate scotoma. (F) Temporal wedge defects are produced by damage to the nasal neuroretinal rim and are not arcuate in nature. (Reprinted with permission from Shields MB. Textbook of Glaucoma. 3rd ed. Baltimore, MD: Williams & Wilkins; 1992.)
METHODS OF PERIMETRY
Representation of the Visual Field
Typically, the results of visual field testing are represented in 2 dimensions. The actual features of the field are determined by the method of testing.
Kinetic visual field testing is performed, as the name implies, with a moving test object. The object, usually a light of variable size and intensity projected on an evenly illuminated background, is moved from a nonseeing area toward a seeing area. The location is recorded when the patient is able to see the object. The process is repeated until a boundary of seeing and nonseeing is determined. This boundary line is called an isopter (Figure 11-3). Several isopters are usually obtained using test objects of different sizes or intensities.
Static visual field testing involves the use of nonmoving test objects. Test objects are presented to the patient for a short period of time. A record of the patient’s response is then made (seen or not seen) at the specific location. There are several different testing strategies for static perimetry. A suprathreshold test uses test objects that have a higher intensity than those that are normally seen at specific locations within the visual field. Threshold testing uses several test objects of increasing or decreasing intensity at each location. Based on patient response to such stimuli, the testing determines the threshold stimulus, or that stimulus that is seen 50% of the time in each location. The result is typically recorded as a symbol, numeric value, or graphically in gray scale (Figure 11-4).
CONFRONTATIONAL VISUAL FIELDS
During the initial evaluation of all new patients, eye care providers should routinely perform confrontation visual field testing as a first step. A confrontation field can often be performed even with the most uncooperative patient in whom one can quickly identify gross field defects. The confrontation field may be of great value and the only practical method for patients who have limited visual acuity due to dense media opacities, such as cataracts or corneal disease. It is also of considerable practical value in determining the location and approximate size of a small residual field in individuals with advanced glaucoma. In some cases, a visual field has been recorded as a central island in patients in whom visual acuity was less than 6/60, due not to media opacity or macular change but to glaucoma itself. In these cases, the true temporal location of the field defect can be quickly determined by confrontational visual field testing.
Tangent Screen
This device consists of an evenly illuminated flat black screen with a central fixation target mounted on a wall. The patient is seated 1 or 2 meters away facing the screen. The central 30 degrees of the visual field can be tested using both kinetic and static methods employing test objects of different size or color.
Although infrequently used today, the tangent screen, when used in experienced hands, can be an extremely valuable method to detect the relative size and location of visual field defects in patients with glaucoma and neuro-ophthalmic disorders. However, similar to confrontation fields, a limitation of using the tangent screen in caring for patients with glaucoma is that it is not possible to detect small progressive changes in the visual field, which is essential to the management of the patient with glaucoma.
Figure 11-3. Normal kinetic and static visual field.
Manual Perimetry
Manual perimetry (visual field testing) involves the use of a perimetrist, an individual skilled in the science (and art) of performing perimetry, and an instrument used by a perimetrist to perform and usually record the result. Several different instruments are available, although some are primarily of historical interest.
Manual Bowl Perimetry
The prototype instrument for most modern perimetry is the Goldmann perimeter. It consists of an evenly illuminated bowl (31.6 apostilbs) with a radius of approximately 300 mm. A chin rest supports the patient’s head. A lens holder is used to provide correct refraction for central visual field testing.
The Goldmann perimeter is used to assess the central and peripheral visual field by employing illuminated test objects of variable size, intensity, and transmission. This instrument can be used for kinetic or static perimetry.
Automated Perimetry
Since the results of manual perimetry can be heavily influenced by the skill and technique of the perimetrist, a great deal of effort has been expended to reduce this variable by auto mating perimetry. Several computer-driven automated instruments are available.
Most automated perimeters use static targets and employ suprathreshold or threshold strategies. Test tar gets can be projected onto the bowl or are integrally placed in the bowl as light-emitting diodes or with fiber optics. Fixation can be monitored with a telescope, verified by periodically testing the blind spot or by monitoring the corneal light reflex. Testing strategies are controlled by a computer, and the results are recorded on a computer printout.
Figure 11-4. Normal (static) automated full-threshold visual field (Humphrey Field Analyzer, Central 24-2).
Despite the implication that these units are fully automated, there is still a critical need for skilled technical support before and during visual field testing with these units to maximize patient performance on these tests (Table 11-1). Prior to administering the test, technicians should check the size of the patient’s pupils. Although relatively uncommon today, patients who are taking miotics or who have pupils with a diameter less than 3 mm may require dilation prior to perimetric testing to prevent artifactual depression of the visual field. Similarly, it is important to check manifest refraction and correct any refractive error present. Patients should be properly positioned so that they are comfortable during perimetric testing, and to optimize performance, careful instruction regarding the process and rationale of the test should be reviewed with the patient prior to initiating the test. The importance of fixation throughout the test should be emphasized to the patient. Simple encouragement can be very helpful for patients, as these tests are often protracted, tiring, and anxiety provoking. Ultimately, the reliability and usefulness of the test result is very dependent on the manner in which the test is conducted.
Reliability indices help the clinician evaluate patient performance. Those indices most commonly used are short-term fluctuation, false-positive responses, false-negative responses, and fixation losses.
Short-term fluctuation represents intratest variability. During the test, several locations are retested, and the differences in threshold values at these locations are determined. Short-term fluctuation is the square root of the variance obtained from the retest values. Some short-term fluctuation is expected. However, when this value is abnormally high, it may represent a lack of attention or reliability on the part of the patient. Alternatively, high amounts of short-term fluctuation may occur as a result of extensive visual field damage.
False-positive errors occur when a patient gives a response when no target is presented (though a sound may be made by the machine). False-positives may result from “trigger happy” patients or those who are trying to “help” the test. The anxiety of not seeing stimuli that are infrathreshold can be substantial. Patients may feel that their condition may be getting worse, and their worry may cause them to respond to stimuli that do not exist. Reassuring the patient before and during the test that it is normal not to see some of the targets can reduce this problem.
False-negative errors occur when the patient fails to respond to a stimulus that is of greater intensity than one seen previously in the same location. False-negatives may represent inattentiveness, a shift in head position, or may be a byproduct of searching for tar gets. Careful reinstruction often remedies this problem.
Fixation losses reduce reliability of visual field testing. Patients may alter fixation as a natural response to stimuli in the peripheral field, a desire to “help” the examiner or, in some cases, due to vision loss when the patient is unable to see the fixation target. Generally, careful instruction during testing will remedy the first 2 of these issues. Some perimeters have a group of fixation targets surrounding central fixation, which helps the patient center his or her gaze when loss of central vision is the primary problem. For patients who are unable to suppress their desire to look around during testing, manual perimetry with constant observation by the perimetrist may be required.
TESTING STRATEGIES
Automated perimeters present stimuli randomly within a fixed pattern. This eliminates the patient’s ability to anticipate the location of the next stimulus and may help reduce fixation losses from this source. Stimulus intensity is adjusted to “fit” the visual field contour, which helps reduce testing time.
Suprathreshold testing involves the use of stimuli that are of greater intensity than the presumed threshold one should be able to visualize at each location. Some instruments present a second high-intensity stimulus at locations where the first stimulus is not detected to differentiate between relative and absolute visual field defects. Suprathreshold testing may be useful for glaucoma screening; however, this test strategy does not precisely delineate the depth of visual field abnormalities and is therefore not suitable for baseline or follow-up examinations of patients known to have glaucoma.
Full-threshold testing is a strategy that quantifies the patient’s retinal threshold for correctly reporting a stimulus 50% of the time. A stimulus is presented at increasing intensity levels of 4 decibels until it crosses the patient’s retinal threshold for detection. Next, the stimulus intensity is reduced in 4-decibel increments until it is unable to be visualized. Finally, this process is repeated using 2- decibel intensity increments to further define the threshold value measured. The visual field pattern typically consists of 70 or more test locations distributed within the central 24 to 30 degrees.
TABLE 11-1. COMMON CAUSES OF VISUAL FIELD ARTIFACT
Source | Comment |
Refractive error | Check refraction |
Improper near correction | Always use full correction according to perimeter manual for patients with pseudophakia, aphakia, and after cycloplegia |
Lid artifact | May need to gently elevate lid with tape |
Lens rim artifact | Reposition patient or corrective lens |
Fatigue or stress | Patient reassurance, rest between visual fields, use SITA-Fast or FASTPAC algorithms, only perform testing on one eye per visit |
Patient movement | — |
Miotic pupil | — |
Cognitive decline or impairment | Reposition patient |
Dilate pupil before testing | Frequent reminders during testing, may require testing using manual perimetry |
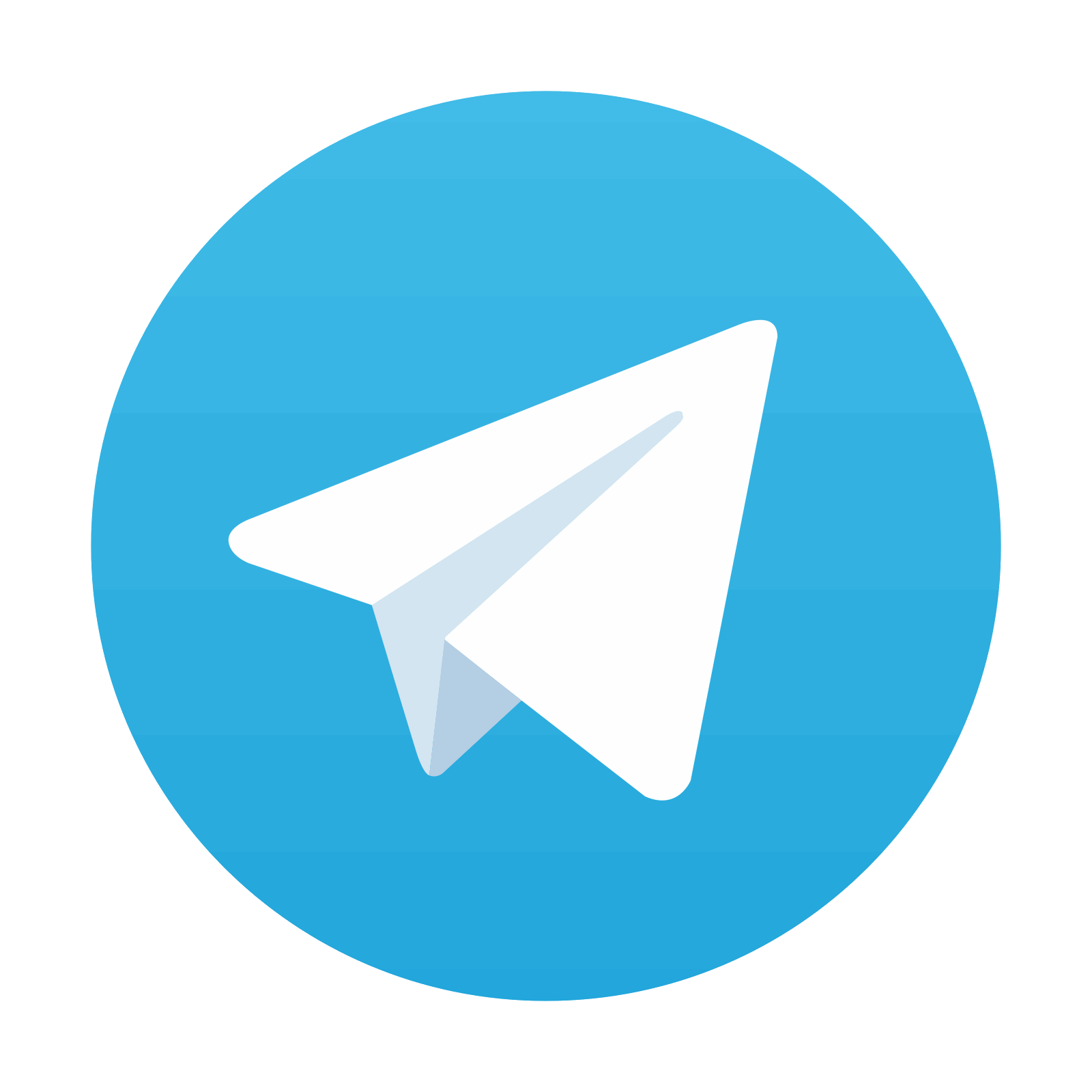
Stay updated, free articles. Join our Telegram channel

Full access? Get Clinical Tree
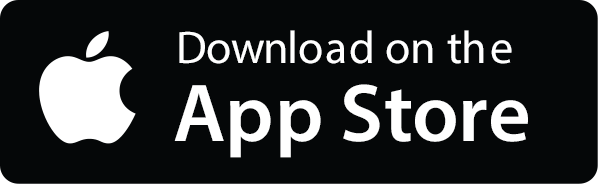
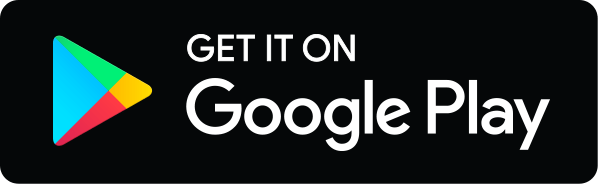