HIGHLIGHTS
- •
Vertical retinal vessel (RV) position is related to the visual field defect (VFD) site.
- •
Eyes with RV in the superior optic nerve head (ONH) region tend to form superior VFDs.
- •
Eyes with RV in the inferior ONH region tend to form inferior VFDs.
- •
RV position is derived from its original position before development of glaucoma.
- •
Original RV position is useful in predicting the initial site of VFD in the glaucoma.
Purpose
The purpose of this study was to investigate the association between the vertical position of the central retinal vessel (CRV) within the optic nerve head (ONH) and the site of visual field defects (VFDs) in glaucoma.
Design
Cross-sectional study.
Methods
The vertical position of the CRV was identified in 134 glaucoma eyes and 61 normal eyes at the point at which CRV exited the lamina cribrosa (LC) onto the ONH surface, by using spectral-domain optical coherence tomography (exit position). The position was also identified at the entry point into the LC from the retrolaminar ONH region (entry position), which was little influenced by glaucomatous LC deformation, therefore close to the original position before the glaucoma development. Positions were compared among glaucoma eyes with different sites of VFDs, and between glaucoma and normal eyes.
Results
In glaucoma eyes, the entry position of the CRV was in the superior ONH region in 63.0% of eyes with superior VFDs and in the inferior ONH region in 97.8% of eyes with inferior VFDs ( P < .0001). The exit position exhibited a similar percentage. The vertical CRV positions were not significantly different between glaucoma and normal eyes, both at the entry and exit positions.
Conclusions
Eyes with CRVs in the superior ONH region were significantly more likely to form VFDs in the superior hemifields and vice versa. The vertical position of the CRV was little altered by the development of glaucoma. The original position of the CRV before the development of glaucoma may influence regional susceptibility to glaucomatous stress and may be useful in predicting initial sites of VFDs.
T he optic nerve head (ONH) presents with characteristic deformations in glaucoma, and ocular structures that consist of the ONH change their shape along with it. The lamina cribrosa (LC) is one of these structures, and it is reported to exhibit deformations such as posterior displacement, , posterior migration at its insertion point, , and thinning. Enlargement of the ONH area has also been reported in glaucoma, including expansion of the Bruch’s membrane opening (BMO) and the anterior scleral canal opening. These are global deformations observed in the overall ONH structure; however, glaucomatous visual field defects (VFDs) do not always occur evenly across the VF but usually first form as a localized scotoma in either the superior or inferior hemifield. , Kim and associates reported regional morphological difference of the LC which corresponded with the location of retinal nerve fiber layer defects (RNFLDs) and suggested the importance of assessing the ONH morphology on a regional basis.
The central retinal vessel (CRV) is located around the central area of the ONH. As known from fundus examination, the position of the CRV on the ONH varies among individuals, and it often decenters into the superior or inferior region of the ONH. The ONHs of newborns are reported to present with very similar, vertically oval shapes; and the CRV is located in the center of the ONH in most eyes. The variations in the positions of the CRV on the ONH in adults is considered to be acquired during eyeball growth. It is possible that a decentered CRV may be related to an uneven structure of the LC through which the CRV passes and, thus, regional variations in the susceptibility to the glaucomatous stress. In fact, Jonas and associates reported the association between the position of the CRV on the LC surface identified on fundus photographs and the location of neuroretinal rim loss or β-zone parapapillary atrophy (PPA).
Recently, these authors investigated passage of the CRV within the LC in glaucomatous eyes by using spectral-domain optical coherence tomography (SD-OCT) (Supplemental Figure). It was found that the CRV changed its direction from perpendicular to nasal when it enters the LC from the retrolaminar ONH region, takes a nasally angled passage within the LC, and exits the LC at its nasal region on the ONH surface, which corresponds to the nasal position of the CRV on the ONH in glaucoma. The angle of the CRV within the LC was very similar to that of the surrounding LC pores, suggesting that the CRV altered its angle along with the LC deformation, which might have occurred during eyeball growth in adolescence or development of glaucoma. It is possible that the position of the CRV on the LC surface evaluated in the previous studies , was already influenced by the LC deformation after passing through the deformed LC. On the other hand, its entry point into the LC is least affected by the LC deformation and thus considered to be close to its original position. Based on these finding, the authors hypothesized that the influence of the position of the CRV to the susceptibility to glaucoma stress, if it existed, could be better evaluated using the CRV entry position into the LC rather than the exit position from it.
Therefore, the present study aimed to explore the association between the position of the CRV within the ONH identified using SD-OCT and location of the VFD in glaucomatous eyes by focusing on the entry position of the CRV into the LC in addition to its exit position from it.
METHODS
SUBJECTS
This cross-sectional study was approved by the institutional review and ethics board of the Akita University Graduate School of Medicine, Akita, Japan, and followed the tenets of the Declaration of Helsinki. Written informed consent was obtained from all participants.
We recruited consecutive patients who visited the glaucoma clinic of the Akita University Graduate School of Medicine between May 2018 and July 2020. All participants underwent a comprehensive ophthalmic assessment, including best-corrected visual acuity, central corneal thickness, and axial length by pachymetry (SP-3000 model; Tomey Corp, Nagoya, Japan), Goldmann applanation tonometry, slit-lamp biomicroscopy, gonioscopy, dilated fundus stereoscopic examination, fundus color stereo photography (Canon, Tokyo, Japan), standard automated perimetry (Humphrey Field Analyzer II model 750; 24-2 Swedish interactive threshold algorithm; Carl Zeiss Meditec, Dublin, California, USA), OCT angiography (Plex Elite 9000 model; Zeiss, Oberkochen, Germany), and SD-OCT (Spectralis, Heidelberg Engineering GmbH, Heidelberg, Germany). SD-OCT was performed within 3 months of the VF test.
This study included eyes with open-angle glaucoma and angle-closure glaucoma that presented with glaucomatous optic disc changes such as localized or diffuse rim thinning and RNFLDs and glaucomatous VFDs corresponding to the glaucomatous structural changes. Glaucomatous VFDs were defined by the presence of at least 3 continuous test points within the same hemifield, on the pattern deviation plot at P <5%, with at least 1 of these points at P <1%, which was confirmed by 2 consecutive reliable tests (fixation loss rate: ≤20%; and false-positive and false-negative error rates: ≤15% each). Exclusion criteria were as follows: 1) myopic eyes with an axial length ≥24.0 mm, in order to eliminate the effects of myopic deformation of the ONH, as myopia alone is reported to cause ONH deformations that may affect position of the CRV ; 2) eyes with poor-quality OCT images (quality score ≤15) for more than 5 sections in serial ONH scans; 3) retinal or neuro-ophthalmologic disease that may affect the VF; and 4) congenital ONH abnormalities and suspected anomalies. Normal eyes were included as controls when they exhibited an intraocular pressure ≤21 mm Hg, an open iridocorneal angle, an optic disc that appeared normal, and no VFDs.
SD-OCT was performed using the enhanced depth imaging (EDI) technique, which improves the visualization of deep structures. , The magnification error was corrected using the formula provided by the manufacturer according to autorefraction keratometry results and the focus setting during image acquisition. The scale of the OCT images was corrected to 1:1 μm before evaluation. Measurements were performed by an experienced glaucoma specialist (Y.S.) who was masked to the clinical information of the participants.
IDENTIFYING THE ENTRY POINT OF THE CENTRAL RETINAL VESSEL INTO AND ITS EXIT POINT FROM THE LAMINA CRIBROSA
The path of the CRV within the LC was identified using EDI OCT by the following process. First, horizontal scans of the ONH were acquired by aligning with the line connecting the fovea and the BMO center ( Figure 1 A). The horizontal scans were set 30 μm apart, and each B-scan was constructed using 35 frames. , , Based on those horizontal scans, the point at which the CRV exited the LC and emerged on the surface of the ONH was identified ( Figure 1 C, red star). The point was plotted at the corresponding position on the ONH in the infrared (IR) fundus image ( Figure 1 D).

Next, radial scans of the ONH centered on the point at which the CRV exited the ONH, as identified in the horizontal scans, were acquired ( Figure 1 E). The radial scans included 48 B-scan images, 3.75 degrees apart, with each B-scan constructed using 42 frames. Based on these radial scans, a scan line that captured the path of the CRV within the LC was obtained ( Figure 1 F). This line passes through the central hump of the LC, which is a thick, mounded area at the center of the LC that embeds the CRV. , In the B-scan image obtained at this line, the point at which the CRV entered the LC from the retrolaminar ONH region was identified as the point at which the CRV changed its direction from perpendicular to nasal ( Figure 1 G, blue star). The point was plotted at the corresponding position on the ONH in the IR image ( Figure 1 H, blue star). In Figure 1 H, the yellow arrow indicates displacement of the CRV during its passage through the deformed LC from the entry position into the LC to the exit position from it.
The CRVs consist of the central retinal artery and vein. Although the retinal artery was easily identified throughout the LC in most eyes, retinal vessels sometimes appeared collapsed and thin, and its entire path was difficult to identify in some eyes. Therefore, in the present study, we assessed the position of the central retinal vessel as the position of the central retinal artery.
ASSESSMENT OF THE COORDINATE OF THE ENTRY AND EXIT POSITIONS OF THE CENTRAL RETINAL VESSEL IN THE OPTIC NERVE HEAD
The coordinates of the position of the CRV in the ONH were assessed vertically and horizontally ( Figure 2 ).

The vertical coordinate of the entry position of the CRV into the LC was assessed relative to the fovea-BMO center line ( Figure 2 B, yellow arrow). Serial vertical OCT scans were obtained perpendicular to the fovea-BMO center line, and a scan line passing through the entry position of the CRV was identified among them ( Figure 2 B, blue arrow). Superior and inferior BMOs were identified in the B-scan image obtained at the scan line and plotted on the corresponding locations in the IR fundus image ( Figure 2 B, white dots on the blue arrow), which was defined as the ONH margin. The vertical coordinate of the entry position of the CRV (V-coordinate entry ) was calculated by the following formula:
V−coordinateentry=(DistancebetweentheentrypositionandthesuperiorBMO/DistancebetweenthesuperiorandinferiorBMO)×100(%).
Therefore, if the entry position was in the superior (inferior) region of the BMO, the V- coordinate entry was presented as <50% (≤50%). The vertical coordinate of the exit position of the CRV from the LC was obtained in the same way, using the scan line passing through the exit position ( Figure 2 B, red arrow).
The horizontal coordinate of the entry position of the CRV (H-coordinate entry ) was assessed using OCT scan lines parallel to the fovea-BMO center line ( Figure 2 C, yellow arrow). Among them, a scan line passing through the entry position of the CRV was identified ( Figure 2 C, blue arrow). Temporal and nasal BMOs were identified in the B-scan image obtained at the scan line, and they were plotted on the corresponding locations in the IR fundus image ( Figure 2 C, white dots on the blue arrow). The H-coordinate entry was calculated by the following formula:
H−coordinateentry=(DistancebetweentheentrypositionandthetemporalBMO/DistancebetweenthetemporalandnasalBMO)×100(%).
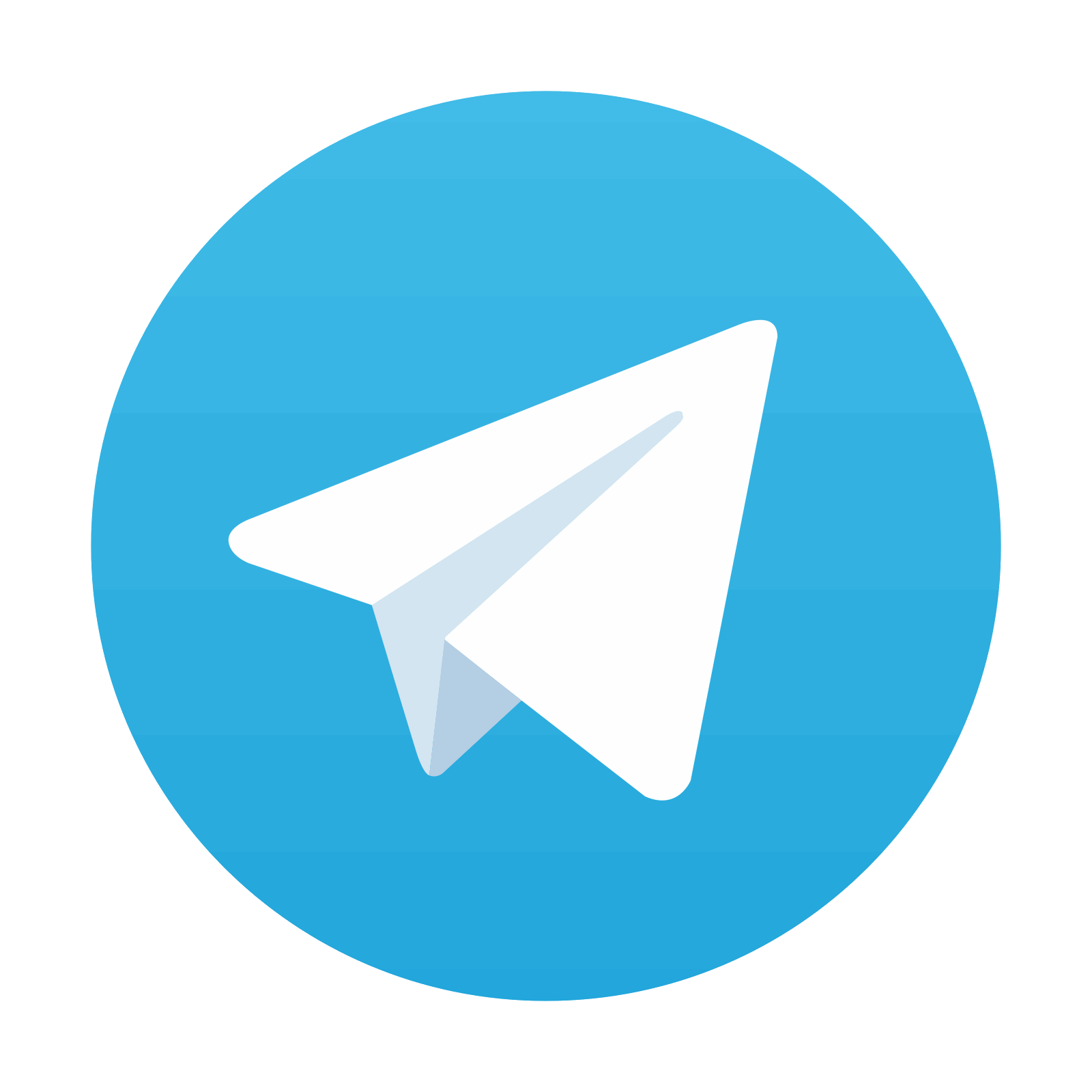
Stay updated, free articles. Join our Telegram channel

Full access? Get Clinical Tree
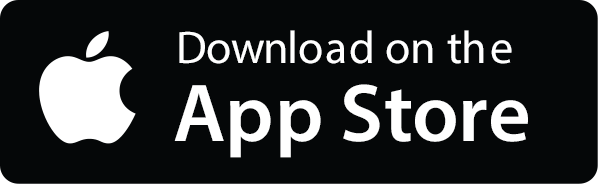
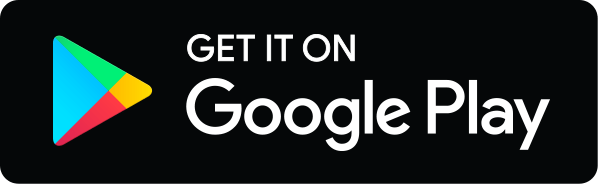
