Overview and clinical context
By the mid-1980s, many uveal melanoma patients were electing treatment by vision-sparing radiation therapy, avoiding surgical removal of the affected eye (enucleation). The Collaborative Ocular Melanoma Study eventually demonstrated no significant difference in survival between patients treated by radiation therapy and enucleation. Although fine-needle aspiration biopsies (FNAB) were used in selected cases to help to discriminate between uveal melanomas and lesions that clinically simulated melanoma (such as metastases to the eye), such procedures were not typically used to grade the melanoma, to assign risk of metastasis to the patients.
At this time, the only parameter of risk that could be assessed from tumor cells extracted by FNAB was cell type, assigned by the Callender classification. The Callender classification of uveal melanomas had been known to be strongly associated with outcome, but the assignment of risk based on the morphological assessment of cells was challenging for two reasons: (1) Callender classification had been shown to be poorly reproducible between pathologists; and (2) epithelioid cells – those cells most strongly associated with adverse outcome – were distributed heterogeneously throughout these tumors. Discrepancies between FNAB-based cytological classifications and the assignment of cell type on the subsequent enucleation were reported, and in one case, the FNAB needle track was traced through the tumor and was found to have missed a pocket of epithelioid cells, leading to an erroneous classification on the FNAB sample. Discrepancies were also reported between the more objective assignments of risk based on the morphometric measurements of nucleolar size between FNAB samples and matched enucleation specimens.
Some experts began to question why it was necessary to assign patients into risk categories if no effective treatments were available to administer to patients with metastatic uveal melanoma. Sadly, even to this day, there is no effective regimen to treat patients with metastatic uveal melanoma. The development of new classification schemes to assign risk was justified then, and is justifiable today, on the basis of two observations: (1) many patients simply want to know the risk to their life expectancy so that they can either make plans to put their affairs in order, or to make longer-term financial and personal commitments ; and (2) there is hope that with intensive research into the molecular basis of metastasis in uveal melanoma, new treatment strategies will emerge and, when this happens, it will be helpful to be able to stratify patients into risk categories to design meaningful clinical trials. Vasculogenic mimicry was therefore discovered in the context of a search to develop a noninvasive substitute for biopsy of uveal melanomas.
In 1992 a pilot study reported the association between death from metastatic uveal melanoma and the presence of histological patterns that stained positive with the periodic acid–Schiff (PAS) reagent. This was followed by a description of nine patterns, including the incorporation of pre-existing choroidal vessels, incomplete circles (arcs) around packets of tumor cells, arcs that bifurcated (arcs with branching), circles around packets of tumor cells (loops), back-to-back loops (three back-to-back loops were designated as networks), parallel linear patterns, and parallel patterns that cross-linked. A subsequent study of the association of these patterns and outcome disclosed that the detection of loops and networks – and the parallel with cross-linking pattern – were associated independently with death from metastatic melanoma in multivariate statistical models. Most patients whose tumors lacked these patterns were long-term survivors of uveal melanoma after enucleation, and fewer than half of the patients whose tumors contained these patterns survived disease-free after enucleation. These patterns, when detected histologically, were interpreted as markers of the risk for metastasis. The association between the detection of these patterns and metastasis was confirmed by multiple independent laboratories and the patterns were subsequently identified in cutaneous melanoma and other cancers.
Investigators eventually discovered a number of important associations between the detection of these patterns and other markers of metastatic behavior, including the presence of epithelioid cells, location in the ciliary body, monosomy 3, and gene expression profiles. Because many markers – cytological, molecular, and cytogenetic – are distributed heterogeneously throughout tumors, a healthy skepticism began to surface about the use of FNAB to extract material from which tumors could be graded on the basis of these markers. Thus, attention was directed to the detection of PAS-positive patterns by noninvasive means as a surrogate marker for more sensitive molecular and cytogenetic markers.
Two approaches were taken to image PAS-positive patterns clinically. A strong association was demonstrated between the analyses of raw radiofrequency ultrasound data and the histological detection of PAS-positive patterns, but the equipment to image and analyze these patterns was never made available commercially. Using indocyanine green angiography and laser scanning confocal ophthalmoscopy, perfusion channels, corresponding to the histologically detected PAS-positive patterns could be imaged clinically for tumors in the posterior pole, and the detection of these patterns was found to be predictive of growth of indeterminate-sized lesions in a prospective trial. Nevertheless, the practice of imaging tumors to detect these patterns clinically by noninvasive means never became part of the clinical ophthalmic oncology workup. However, the detection of fluid within these patterns through angiography strengthened the suspicion that PAS-positive patterns were part of the microcirculation.
Indeed, in histological sections, PAS-positive patterns were associated with blood vessels and red blood cells were detected within the patterns. It was assumed that these patterns were remodeled blood vessels, and the patterns were initially designated as “microcirculatory patterns.” No one had any reason to challenge this assumption until the histogenesis of these patterns was studied in vitro.
The histogenesis of PAS-positive patterning: vasculogenic mimicry
Because PAS-positive patterns were thought to represent remodeled blood vessels, the histogenesis of these patterns was first explored in vitro in co-cultures of uveal melanoma cells, endothelial cells, and fibroblasts. However, looping patterns, identical to those seen in tissue sections, were generated by highly invasive uveal melanoma cells, in the absence of endothelial cells and fibroblasts in three-dimensional culture conditions. Interestingly, poorly invasive uveal melanoma cells did not generate these patterns under any culture condition, thus establishing a functional relationship between the in vitro observations and the association between the identification of these patterns in tissue sections and death from metastatic melanoma. Moreover, these patterns – formed in vitro exclusively by highly invasive uveal melanoma cells – conducted fluid after direct injection and after iontophoresis, strengthening the hypothesis that these patterns conducted fluid in vivo. The histogenesis of these patterns was described as vasculogenic mimicry – vasculogenic because, although these pathways do not form from pre-existing vessels, they distribute plasma and may contain red blood cells; and mimicry because the pathways are not blood vessels and merely mimic vascular function by functioning as a “fluid-conducting meshwork.”
Questions asked about vasculogenic mimicry
Are vasculogenic mimicry patterns blood vessels?
Looping PAS-positive patterns are not blood vessels. The patterns do not stain with endothelial cell markers and they are composed ultrastructurally of sheets of electron-dense material in which tumor cells are embedded. The patterns are composed of laminin, fibronectin, collagens IV and VI, and possibly heparan sulfate proteoglycan. Three-dimensional reconstructions have shown these patterns to represent sleeves of extracellular matrix material wrapped around branching cylindrical projections of melanoma cells. Plasma and some red blood cells are conducted by the patterned extracellular matrix which connects focally to blood vessels. Thus, vasculogenic mimicry patterns are not blood vessels by composition, ultrastructure, or topology, although they do conduct fluid.
Investigators have described the formation of tubes by melanoma cells and have identified tubular structures in tissue sections of melanomas and other tumors that are lined by tumor cells and not endothelial cells. One group has advanced the hypothesis that highly invasive and genetically dysregulated melanoma cells undergo transdifferentiation into an endothelial cell genotype (because of the upregulation of genes such as VE-cadherin). There are several challenges to this approach. First, vascular spaces in tissue may be formed by tumor cells replacing endothelial cells – a “complete” manifestation of mosaic tumor vessels. Second, even if transdifferentiation does provide a mechanism for the generation of tubes of tumor cells mimicking the appearance of blood vessels, the transdifferentiation is incomplete because tumor cells do not form cobblestone monolayers in vitro as do endothelial cells and because angiogenesis inhibitors do not block the formation of tumor cell-generated cords.
The generation of the highly patterned fluid-conducting meshwork has been designated as vasculogenic mimicry of the patterned matrix type, and the formation of tubes by tumor cells has been called vasculogenic mimicry of the tubular type. The material that follows in this chapter refers only to vasculogenic mimicry of the patterned matrix type.
Are vasculogenic mimicry patterns a stromal response to the tumor (i.e., are these patterns fibrovascular septa)?
Before the histogenesis of PAS-positive patterns was described, some investigators assumed that these patterns represented fibrovascular septa. Even after it had been shown that tumor cells generated these patterns, some investigators persisted in describing these patterns as stromal response to the tumor. Although fibrovascular septa have been identified in uveal melanomas, their prevalence is low and fibrovascular septa do not have any prognostic associations with outcome. Perhaps the most convincing evidence in support of the tumor cell generating these patterns comes from a set of experiments in which human uveal melanoma cells were injected into the livers of immunosuppressed mice. Polyclonal antibodies to laminin, not species-specific, labeled vasculogenic mimicry patterns generated by the tumor cells as well as mouse liver structures. However, a monoclonal antibody that was species-specific for human laminin labeled only vasculogenic mimicry patterns within the tumor and not the mouse liver. Thus, the laminin within the tumor was not co-opted from the mouse stroma and vasculogenic mimicry patterns are therefore not a stromal response by host tissue to the tumor.
Does fluid flow through vasculogenic mimicry patterns?
It is clear from animal model studies that intravenous tracers co-localize to vasculogenic mimicry patterns. In a recent clinical study, patients with posterior uveal melanomas were injected with indocyanine green in the antecubital vein of one arm, and shortly after injection, blood was phlebotomized from the contralateral arm while a confocal angiogram was being taken. The blood removed after injection continued to fluoresce weeks after the injection, while fluorescence within intratumoral vasculogenic mimicry patterns was extinguished within 15 minutes after injection, thus demonstrating indirectly that fluid flows through vasculogenic mimicry patterns. It is possible that leaky intratumoral vessels permit blood to enter into the tumor cell-generated extracellular matrix, but that once in the matrix, plasma and red blood cells circulate throughout the patterns.
What is the relationship between vasculogenic mimicry and angiogenesis?
In the early 1990s, an association was demonstrated between increased microvascular density in breast cancer and adverse outcome, and a large series of papers then followed demonstrating similar associations in other cancers, including uveal melanoma. Although it was intuitive that increased risk of metastasis should accompany angiogenesis, these associations were somewhat paradoxical because highly invasive cancers are typically destructive of the host microenvironment: by what mechanisms would new blood vessels be able to penetrate into the cellular compartment of highly malignant tumors when these tumors were simultaneously elaborating a variety of substances leading to the degradation of stroma? Indeed, a careful examination of angiogenesis in breast cancer revealed that angiogenic blood vessels were situated in the fibrous connective tissue surrounding tumor cells and were not in direct contact with the tumor cell compartment, consistent with the notion of the tumor stroma representing a form of scar tissue. Furthermore, when endothelial cells were co-cultured with highly invasive uveal melanoma cells, the uveal melanoma cells destroyed the endothelial cells on contact.
It was known that highly invasive uveal melanoma cells are genetically dysregulated and express markers that are inappropriate for cells of neural crest lineage (like fetal cytokeratins and endothelial cells such as VE-cadherin ). Therefore, the relationship of microvascular density to adverse outcome in uveal melanoma was studied by double-labeling histological sections with CD34 (a nonspecific endothelial cell marker found by one group to provide for the highest microvascular density measurements), and with S100 protein (a nonspecific marker of cells of neural crest lineage such as melanocytes that do not label vascular endothelial cells). A high level of co-expression of CD34 and S100 protein was identified, and as “microvascular density” – as measured by CD34 labeling – increased, so did co-expression of this protein by melanoma cells. Therefore, the association between high microvascular density and metastasis in uveal melanoma may be explained on the basis of a population of highly invasive and genetically dysregulated tumor cells rather than angiogenesis. Aberrant expression of CD34 in cutaneous melanoma was discovered subsequently.
The microcirculation of uveal melanoma is therefore complex, including normal choroidal vessels that are incorporated into tumors, angiogenic vessels (especially next to zones of necrosis or in tumors previously treated by radiation therapy), mosaic vessels (lined by tumor cells and endothelial cells), and vasculogenic mimicry patterns. Vasculogenic mimicry patterns provide at least 11-fold increased perfusion surface area in comparison to incorporated tubular vessels or angiogenic vessels.
One might speculate that vasculogenic mimicry patterns facilitate metastasis, functioning like lymphatic channels in a location devoid of lymphatic vessels. However, there is no direct evidence implicating vasculogenic mimicry in the dissemination of tumor cells. Because vasculogenic mimicry patterns are formed by highly aggressive melanoma cells, it is possible that the detection of these patterns is merely a marker for the presence of an aggressive tumor phenotype and therefore has little or nothing to do with the actual spread of tumor.
Similarly, one might speculate that plasma and red blood cells circulating through vasculogenic mimicry patterns provide sufficient oxygen and nutrients to prevent necrosis. Most uveal melanomas lack zones of necrosis: the architecture of most uveal melanomas is far different from highly angiogenic retinoblastomas which feature zones of necrosis beyond a narrow cuff of tumor cells surrounding blood vessels. However, plasma flowing through vasculogenic mimicry patterns is not likely to be well oxygenated, and only scattered red blood cells – most often in a rouleaux formation – are identified histologically within these patterns. Is it possible, therefore, that uveal melanomas may not require a high degree of oxygenation? Might vasculogenic mimicry patterning serve a function other than perfusion or the facilitation of metastasis? Indeed, there is evidence that vasculogenic mimicry patterning regulates the behavior of tumor cells.
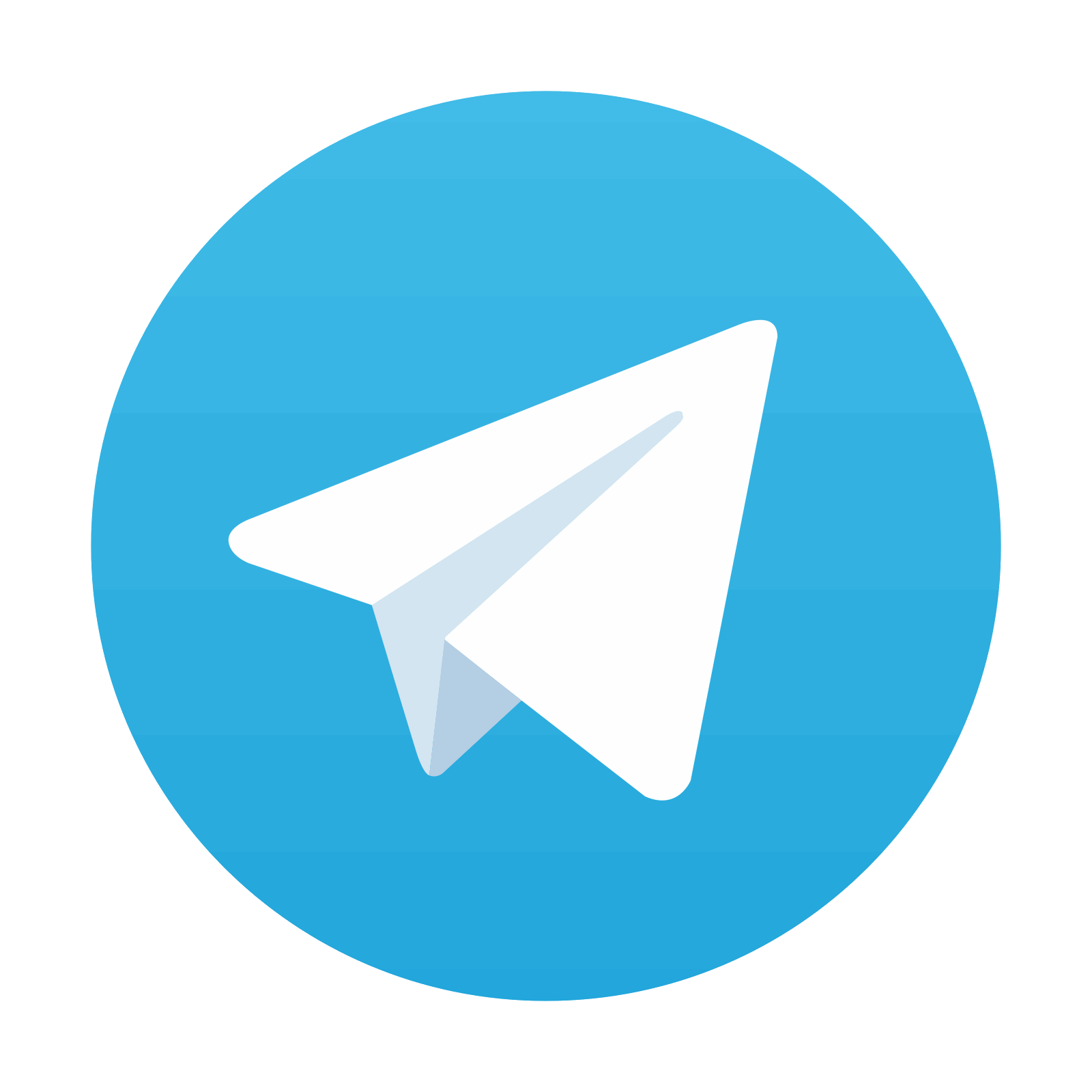
Stay updated, free articles. Join our Telegram channel

Full access? Get Clinical Tree
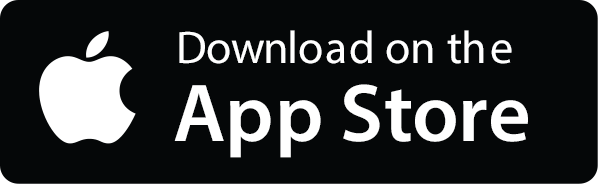
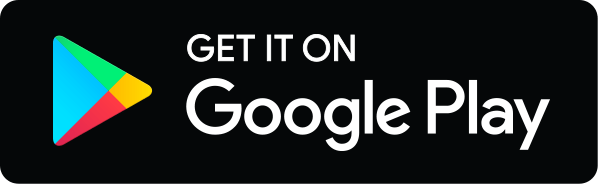