Anthony Joseph, MD and Ehsan Rahimy, MD
Early Research
Vascular endothelial growth factor (VEGF) was officially discovered in 1983 as vascular permeability factor (VPF) by Senger and colleagues at Beth Israel Hospital in Boston.1 However, the notion of such a biologic mediator and the significance of angiogenesis existed long before its physical isolation. As far back as the late 1800s, several German pathologists, including the renowned Rudolph Virchow, observed increased vascularity in some human tumors, suggesting that new vessels played a role in these cancers.2 Over the ensuing decades, scientists continued to describe the varying vascular patterns of different tumors3 until 1939, when Gordon Ide and colleagues noted the correlation between tumor growth and blood vessel formation using a rabbit model and transplanted carcinoma.4 Their observation of a marked angiogenic response associated with tumor transplantation led them to hypothesize the existence of a vascular growth factor produced by tumor cells. Algire and a group at the National Cancer Institute built on this work in 1945, demonstrating that transplanted tumor tissue in rats led to increased vessel formation while transplanted normal tissue did not. This finding again suggested the presence of a vascular growth factor associated with tumor tissue.5
Some time passed for these postulations on angiogenic factors to translate into experimental evidence of their existence. In 1968, two different groups published research supporting the release of these factors. Both Greenblatt and Shubi6 as well as Ehrmann and Knoth7 showed that melanoma and choriocarcinoma cells in a hamster model stimulated the growth of blood vessels in a host even when filter paper physically separated the tumor cells from the host. These findings strongly suggested the presence of a diffusible factor promoting vascular proliferation produced by tumor cells.
As interest continued to grow in the role of angiogenesis factors in cancer, Judah Folkman published his seminal work on the topic in 1971 with the novel idea that vascular proliferation was instrumental for tumor progression, and accordingly, that inhibition of angiogenesis could provide a potential approach to treating malignancy.8 Along with his colleagues, he also began work to isolate a tumor angiogenesis factor (TAF) from human and animal tumors that would incite blood vessel proliferation in a rat model.8,9 He coined the term anti-angiogenesis referring to prevention of vascular proliferation into early tumors through inhibition of such angiogenesis factors, giving rise to a new field of research into therapies for cancer and eventually vascular diseases of the eye.
In the decade following Folkman’s report, research accelerated to isolate and identify different angiogenesis factors. Even so, after isolating a specific biologic mediator, progress was limited by the time required to purify and subsequently reproduce sufficient amounts of the compound, a task that could take years in the era before gene sequencing.2 Once again, Folkman’s group provided a stimulus to the field with their description of a method for the production of pure capillary endothelial cells.10 These cells could be used to study angiogenesis factors in vitro, and as a result, many researchers began to study the effects of different biologic factors on endothelial cell proliferation. Epidermal growth factor, transforming growth factor (-α and-β), tumor necrosis factor, and angiogenin were shown to stimulate endothelial cell proliferation either directly or indirectly through the action of inflammatory cells.2,11 Special consideration was also given to acidic fibroblast growth factor (aFGF) identified by Maciag and colleagues in 197912 and basic fibroblast growth factor (bFGF) identified by Gospodarowicz in 197413 as they were shown to stimulate both endothelial cells and a variety of other cell types. Even so, early trials to correlate FGF with tumor angiogenesis yielded negative results.2 More recently, antibodies against bFGF were not found to hinder tumor growth in mouse models,14 and mouse models with both defective aFGF and bFGF genes did not demonstrate vascular defects. In the meantime, researchers continued to hunt for angiogenesis factors more directly related to tumorigenesis.
Isolation of Vascular Endothelial Growth Factor
In 1983, more than a decade after Folkman published his research on the therapeutic implications of tumor angiogenesis, Senger and Dvorak, along with their colleagues at Beth Israel Hospital, found that ascites fluid associated with tumors in guinea pigs, hamsters, and mice had activity that increased microvascular permeability. They partially purified the active protein from a guinea pig line and referred to this mediator as VPF.1 The authors recognized that VPF appeared to play a role in the hyper-permeability of tumor vasculature, but they were seemingly unaware of its angiogenic properties. Furthermore, since the protein was not completely purified and sequenced, its identity remained unknown for several years.
Then, in 1989, two groups simultaneously reported findings on VEGF and VPF in back-to-back articles in the same issue of Science.15,16 Napoleone Ferrara and his group at Genentech used a media conditioned by bovine pituitary follicular cells to purify a biologic factor that induced angiogenesis in vivo.16 The isolated protein was termed vascular endothelial growth factor, and given its great angiogenic activity, the authors hypothesized that it was a secreted factor different from aFGF, bFGF, and platelet-derived growth factor (PDGF), which were stored factors previously shown to exist in the same cells.17 Indeed, N-terminal amino acid sequencing conclusively showed that VEGF was a unique protein not described in any database.18
At the same time, Connolly and his group at Monsanto Company had worked to purify and sequence human VPF,15 building on Senger’s previous work. They found the protein to be active in increasing blood vessel permeability as well as endothelial cell growth and angiogenesis. In their research, Ferrara’s group had identified complementary DNA (cDNA) clones encoding for 3 different molecular species of VEGF containing 121, 165, and 189 amino acids. As it turned out, the cDNA clone identified by Connolly’s group encoded a protein identical to VEGF189, revealing that VEGF and VPF were indeed the same factor, even though the former was isolated because of its angiogenic activity while the latter was isolated through its propensity to stimulate vascular permeability. Full sequencing of the gene encoding for VEGF demonstrated that it and PDGF shared similar genetic characteristics as well.2 Additionally, around the same time, various groups purified factors in other species that stimulated endothelial cell proliferation. These factors, such as vasculotropin in mice19 and VPF in guinea pigs,20 were found to be orthologues of VEGF, thus demonstrating the conservation of VEGF across species.2
Further examination of VEGF in the succeeding years demonstrated varied molecular properties of the different isoforms. VEGF165, a diffusible heparin-binding variant, was found to be the most abundant form.21 VEGF121 was noted to be freely diffusible while VEGF189 was largely bound to the cell surface and extracellular matrix.22,23 Consequently, VEGF could be directly secreted as its shorter isoforms or released by protease activation and cleavage of longer isoforms.2
In the following years, VEGF was found to play a role in many human physiologic processes. VEGF actually encompasses 5 major subtypes: VEGF-A, VEGF-B, VEGF-C, VEGF-D, and placental growth factor (PlGF). The original VEGF was later termed VEGF-A as the other family members were discovered. As described above, VEGF-A plays a prominent role in angiogenesis and vascular permeability, often with respect to tumor proliferation. Additionally, VEGF-A affects other angiogenic processes such as wound healing, ovulation, menstruation, and pregnancy.24 VEGF-B is less well understood, but is highly expressed in striated muscle, myocardium, and brown fat. Research suggests it plays a role in embryonic angiogenesis, especially with respect to the heart.25 VEGF-C is produced as a precursor protein and induces selective lymphangiogenesis without accompanying angiogenesis.26 It appears to play a role in lymphatic invasion of tumors and lymphatic metastasis.27 VEGF-D is closely related to VEGF-C and also plays a pivotal role in tumor lymphangiogenesis and lymphatic metastasis as well as angiogenesis.28 Finally, PlGF was originally discovered in the placenta but is also expressed in the heart and lungs.29 Loss of PlGF impairs angiogenesis in ischemia, wound healing, inflammation, and cancer.30 Other VEGF derivatives have also been discovered, including VEGF-E encoded by the Orf virus genome31 and VEGF-F identified from viper snake venom,32 which may have therapeutic implications down the road. Undoubtedly, the VEGF family of proteins plays a broad and significant role in human pathophysiology, a role eventually found to extend to ocular conditions as well.
Vascular Endothelial Growth Factor in the Eye
Just as the notion of a tumor-related angiogenesis factor existed long before its actual identification, eye researchers independently theorized the idea of VEGF decades before it was discovered. As early as 1948, Michaelson proposed the existence of a diffusible factor responsible for retinal vascular growth in various developmental and disease processes.33 This unknown factor would come to be known as Factor X, and George Wise later elaborated on the topic in his 1956 thesis suggesting that retinal neovascularization was stimulated by retinal tissue factor x, a mediator presumed to be related to tissue anoxia.34 Clinicians recognized the correlation between retinal injury and new blood vessel growth elsewhere in the eye, but it would be decades before Judah Folkman published his seminal paper on TAF, research that coincidentally relied on eye models in many cases.8,35
In 1980, just before Senger and Dvorak published their work on VPF, Glaser and D’Amore, along with their colleagues at Johns Hopkins University, recognized that the retina and vitreous might be potential sources of vasoproliferative factors in the eye.36–38 In another parallel to the search for a TAF, aFGF39 and later bFGF40 were suggested as possible candidates for Factor X after being identified as angiogenic mediators in the eye. But these compounds were not secreted, so the search for Factor X continued.35
In the meantime, Ferrara’s team and Connolly’s team had independently isolated VEGF,15,16 which soon became a leading candidate for Factor X. Studies using a glioblastoma tumor model confirmed that VEGF expression correlated with vascular proliferation and was induced by hypoxia.41,42 These findings were consistent with the suspected mechanism of neovascularization in various retinopathies and coincided with the original postulations about Factor X. Additionally, VEGF stimulated vascular permeability, another common feature in retinal vascular disorders in which Factor X was suspected to play a role.35,43
With these implications in mind, researchers began to investigate the potential role of VEGF in ocular disease. Adamis and Shima, along with D’Amore and Folkman, used in vitro experiments to demonstrate that human retinal pigment epithelial cells could synthesize VEGF.44 Shima and colleagues went on to establish that expression of VEGF by retinal cells was promoted by hypoxia45 and correlated spatially and temporally with the onset of angiogenesis in the eye.46 These findings suggested that induction of VEGF by hypoxia mediated the neovascular response to retinal ischemia seen in various retinal vascular conditions.
At the same time, Miller and collaborators at the Massachusetts Eye and Ear Infirmary became the first to confirm this hypothesis in vivo using aqueous samples collected from monkey eyes with laser-induced vein occlusion. The resultant retinal ischemia induced iris neovascularization, which was consistent with the presence of a diffusible molecule. Additionally, aqueous samples revealed that levels of VEGF and its associate messenger RNA (mRNA) were elevated both synchronously and proportionally to the extent of new blood vessel growth in the iris.47 The same monkey model was later used to establish that production of VEGF occurred in the inner retina with expression of the secreted VEGF121 and VEGF165 variants.48 These findings pushed VEGF to the front as the likely candidate for a retinal-derived vascular permeability and angiogenic factor.
The next step was to translate in vitro and animal model findings to humans. Aiello and collaborators measured the concentration of VEGF in aqueous or vitreous from patients undergoing intraocular surgery. They found VEGF was more prevalent in patients with ischemic retinal disease including diabetic retinopathy and retinal vein occlusions compared to those with no neovascular disorders.49 Adamis’s group had similar findings using vitreous samples from patients with proliferative diabetic retinopathy.50 These studies provided some of the most direct evidence yet regarding a key role for VEGF in neovascular eye disease.
Up until this point, researchers had correlated the presence and level of VEGF with ischemic eye disease and proliferative changes. They now looked to show a more causative link by establishing whether VEGF was sufficient to incite neovascularization in the eye. Using the primate model described previously, Miller and Tolentino demonstrated that intravitreal injections of recombinant human VEGF165 produced iris neovascularization with prolonged exposure leading to ectropion uveae and neovascular glaucoma.51 They used the same technique to further show that VEGF was sufficient to produce retinal ischemia and microangiopathy in normal eyes with VEGF165 injections leading to the development of hemorrhage, edema, venous beading, microaneurysms, capillary occlusion with ischemia, and vascular proliferation.52
At the same time, researchers examined VEGF inhibition to solidify the role of VEGF in ischemic ocular disease. Aiello and company used a soluble VEGF-receptor protein to suppress retinal neovascularization in vivo in animals with an oxygen-induced ischemic retinopathy.53 Robinson and Smith then used an anti-VEGF aptamer in a murine model of proliferative retinopathy to inhibit retinal neovascularization.54 Meanwhile, Adamis and Miller used their primate model to establish that injections of a monoclonal antibody to VEGF prevented iris neovascularization in eyes with retinal ischemia secondary to laser-induced vein occlusion.55 This antibody was the precursor to bevacizumab.35 It was 1996 by this time, and the clinical potential for VEGF inhibition in neovascular eye disease was evident. It would still be years before pharmaceutical companies would put the resources toward ophthalmic clinical trials necessary to bring anti-VEGF therapy to everyday practice, but this marked the beginning of a new era in treating previously blinding retinal disease.
References
1. Senger DR, Galli SJ, Dvorak AM, Perruzzi CA, Harvey VS, Dvorak HF. Tumor cells secrete a vascular permeability factor that promotes accumulation of ascites fluid. Science. 1983;219(4587):983-985.
2. Ferrara N. VEGF and the quest for tumour angiogenesis factors. Nat Rev Cancer. 2002;2(10):795-803.
3. Lewis WH. The vascular pattern of tumors. Johns Hopkins Hosp Bull. 1927;41:156-162.
4. Ide AG, Baker NH, Warren SL. Vascularization of the Brown Pearce rabbit epithelioma transplant as seen in the transparent ear chamber. Am J Roentgenol. 1939;42:891-899.
5. Algire GH, Chalkley HW. Vascular reactions of normal and malignant tissues in vivo. I. Vascular reactions of mice to wounds and to normal and neoplastic transplants. J Natl Cancer Inst. 1945;6:73-85.
6. Greenblatt M, Shubi P. Tumor angiogenesis: transfilter diffusion studies in the hamster by the transparent chamber technique. J Natl Cancer Inst. 1968;41(1):111-124.
7. Ehrmann RL, Knoth M. Choriocarcinoma. Transfilter stimulation of vasoproliferation in the hamster cheek pouch. Studied by light and electron microscopy. J Natl Cancer Inst. 1968;41(6):1329-1341.
8. Folkman J. Tumor angiogenesis: therapeutic implications. N Engl J Med. 1971;285(21):1182-1186.
9. Folkman J, Merler E, Abernathy C, Williams G. Isolation of a tumor factor responsible for angiogenesis. J Exp Med. 1971;133(2):275-288.
10. Folkman J, Haudenschild CC, Zetter BR. Long-term culture of capillary endothelial cells. Proc Natl Acad Sci U S A. 1979;76(10):5217-5221.
11. Folkman J, Klagsbrun M. Angiogenic factors. Science. 1987;235(4787):442-447.
12. Maciag T, Cerundolo J, Ilsley S, Kelley PR, Forand R. An endothelial cell growth factor from bovine hypothalamus: identification and partial characterization. Proc Natl Acad Sci U S A. 1979;76(11):5674-5678.
13. Gospodarowicz D. Localisation of a fibroblast growth factor and its effect alone and with hydro-cortisone on 3T3 cell growth. Nature. 1974;249(453):123-127.
14. Dennis PA, Rifkin DB. Studies on the role of basic fibroblast growth factor in vivo: inability of neutralizing antibodies to block tumor growth. J Cell Physiol. 1990;144(1):84-98.
15. Keck PJ, Hauser SD, Krivi G, et al. Vascular permeability factor, an endothelial cell mitogen related to PDGF. Science. 1989;246(4935):1309-1312.
16. Leung DW, Cachianes G, Kuang WJ, Goeddel DV, Ferrara N. Vascular endothelial growth factor is a secreted angiogenic mitogen. Science. 1989;246(4935):1306-1309.
17. Ferrara N, Schweigerer L, Neufeld G, Mitchell R, Gospodarowicz D. Pituitary follicular cells produce basic fibroblast growth factor. Proc Natl Acad Sci U S A. 1987;84(16):5773-5777.
18. Ferrara N, Henzel WJ. Pituitary follicular cells secrete a novel heparin-binding growth factor specific for vascular endothelial cells. Biochem Biophys Res Commun. 1989;161(2):851-858.
19. Plouët J, Schilling J, Gospodarowicz D. Isolation and characterization of a newly identified endothelial cell mitogen produced by AtT-20 cells. EMBO J. 1989;8(12):3801-3806.
20. Senger DR, Connolly DT, Van de Water L, Feder J, Dvorak HF. Purification and NH2-terminal amino acid sequence of guinea pig tumor-secreted vascular permeability factor. Cancer Res. 1990;50(6):1774-1778.
21. Houck KA, Ferrara N, Winer J, Cachianes G, Li B, Leung DW. The vascular endothelial growth factor family: identification of a fourth molecular species and characterization of alternative splicing of RNA. Mol Endocrinol. 1991;5(12):1806-1814.
22. Park JE, Keller GA, Ferrara N. The vascular endothelial growth factor (VEGF) isoforms: differential deposition into the subepithelial extracellular matrix and bioactivity of extracellular matrix-bound VEGF. Mol Biol Cell. 1993;4(12):1317-1326.
23. Houck KA, Leung DW, Rowland AM, Winer J, Ferrara N. Dual regulation of vascular endothelial growth factor bioavailability by genetic and proteolytic mechanisms. J Biol Chem. 1992;267(36):26031-26037.
24. Otrock ZK, Makarem JA, Shamseddine AI. Vascular endothelial growth factor family of ligands and receptors: review. Blood Cells Mol Dis. 2007;38(3):258-268.
25. Claesson-Welsh L. VEGF-B taken to our hearts: specific effect of VEGF-B in myocardial ischemia. Arterioscler Thromb Vasc Biol. 2008;28(9):1575-1576.
26. Jeltsch M, Kaipainen A, Joukov V, et al. Hyperplasia of lymphatic vessels in VEGF-C transgenic mice. Science. 1997;276(5317):1423-1425.
27. Fujimoto J, Toyoki H, Sato E, Sakaguchi H, Tamaya T. Clinical implication of expression of vascular endothelial growth factor-C in metastatic lymph nodes of uterine cervical cancers. Br J Cancer. 2004;91(3):466-469.
28. Stacker SA, Caesar C, Baldwin ME, et al. VEGF-D promotes the metastatic spread of tumor cells via the lymphatics. Nat Med. 2001;7(2):186-191.
29. Persico MG, Vincenti V, DiPalma T. Structure, expression and receptor-binding properties of placenta growth factor (PlGF). Curr Top Microbiol Immunol. 1999;237:31-40.
30. Carmeliet P, Moons L, Luttun A, et al. Synergism between vascular endothelial growth factor and placental growth factor contributes to angiogenesis and plasma extravasation in pathological conditions. Nat Med. 2001;7(5):575-583.
31. Lyttle DJ, Fraser KM, Fleming SB, Mercer AA, Robinson AJ. Homologs of vascular endothelial growth factor are encoded by the poxvirus orf virus. J Virol. 1994;68(1):84-92.
32. Suto K, Yamazaki Y, Morita T, Mizuno H. Crystal structures of novel vascular endothelial growth factors (VEGF) from snake venoms: insight into selective VEGF binding to kinase insert domain-containing receptor but not to fms-like tyrosine kinase-1. J Biol Chem. 2005;280(3):2126-2131.
33. Michaelson IC. The mode of development of the vascular system of the retina, with some observations on its significance for certain retinal diseases. Trans Ophthalmol Soc U K. 1948;68:137-180.
34. Wise GN. Retinal neovascularization. Trans Am Ophthalmol Soc. 1956;54:729-826.
35. Miller JW. The Harvard angiogenesis story. Surv Ophthalmol. 2014;59(3):361-364.
36. Glaser BM, D’Amore PA, Lutty GA, Fenselau AH, Michels RG, Patz A. Chemical mediators of intraocular neovascularization. Trans Ophthalmol Soc U K. 1980;100(3):369-373.
37. Glaser BM, D’Amore PA, Michels RG, et al. The demonstration of angiogenic activity from ocular tissues. Preliminary report. Ophthalmology. 1980;87(5):440-446.
38. Glaser BM, D’Amore PA, Michels RG, Patz A, Fenselau A. Demonstration of vasoproliferative activity from mammalian retina. J Cell Biol. 1980;84(2):298-304.
39. D’Amore PA, Glaser BM, Brunson SK, Fenselau AH. Angiogenic activity from bovine retina: partial purification and characterization. Proc Natl Acad Sci U S A. 1981;78(5):3068-3072.
40. Sivalingam A, Kenney J, Brown GC, Benson WE, Donoso L. Basic fibroblast growth factor levels in the vitreous of patients with proliferative diabetic retinopathy. Arch Ophthalmol. 1990;108(6):869-872.
41. Plate KH, Breier G, Welch HA, Risau W. Vascular endothelial growth factor is a potential tumour angiogenesis factor in human gliomas in vivo. Nature. 1992;359(6398):845-848.
42. Shweiki D, Itin A, Soffer D, Keshet E. Vascular endothelial growth factor induced by hypoxia may mediate hypoxia-initiated angiogenesis. Nature. 1992;359(6398):843-845.
43. Kim LA, D’Amore PA. A brief history of anti-VEGF for the treatment of ocular angiogenesis. Am J Pathol. 2012;181(2):376-379.
44. Adamis AP, Shima DT, Yeo KT, et al. Synthesis and secretion of vascular permeability factor/vascular endothelial growth factor by human retinal pigment epithelial cells. Biochem Biophys Res Commun. 1993;193(2):631-638.
45. Shima DT, Deutsch U, D’Amore, PA. Hypoxic induction of vascular endothelial growth factor (VEGF) in human epithelial cells is mediated by increases in mRNA stability. FEBS Lett. 1995;370(3):203-208.
46. Shima DT, Adamis AP, Ferrara N, et al. Hypoxic induction of endothelial cell growth factors in retinal cells: identification and characterization of vascular endothelial growth factor (VEGF) as the mitogen. Mol Med. 1995;1(2):182-193.
47. Miller JW, Adamis AP, Shima DT, et al. Vascular endothelial growth factor/vascular permeability factor is temporally and spatially correlated with ocular angiogenesis in a primate model. Am J Pathol. 1994;145(3):574-584.
48. Shima DT, Gougos A, Miller JW, et al. Cloning and mRNA expression of vascular endothelial growth factor in ischemic retinas of Macaca fascicularis. Invest Ophthalmol Vis Sci. 1996;37(7):1334-1340.
49. Aiello LP, Avery RL, Arrigg PG, et al. Vascular endothelial growth factor in ocular fluid of patients with diabetic retinopathy and other retinal disorders. N Engl J Med. 1994;31(22):1480-1487.
50. Adamis AP, Miller JW, Bernal MT, et al. Increased vascular endothelial growth factor levels in the vitreous of eyes with proliferative diabetic retinopathy. Am J Ophthalmol. 1994;118(4):445-450.
51. Tolentino MJ, Miller JW, Gragoudas ES, Chatzistefanou K, Ferrara N, Adamis AP. Vascular endothelial growth factor is sufficient to produce iris neovascularization and neovascular glaucoma in a nonhuman primate. Arch Ophthalmol. 1996;114(8):964-970.
52. Tolentino MJ, Miller JW, Gragoudas ES, et al. Intravitreous injections of vascular endothelial growth factor produce retinal ischemia and microangiopathy in an adult primate. Ophthalmology. 1996;103(11):1820-1828.
53. Aiello LP, Pierce EA, Foley ED, et al. Suppression of retinal neovascularization in vivo by inhibition of vascular endothelial growth factor (VEGF) using soluble VEGF-receptor chimeric proteins. Proc Natl Acad Sci U S A. 1995;92(23):10457-10461.
54. Robinson GS, Pierce EA, Rook SL, Foley E, Webb R, Smith LE. Oligodeoxynucleotides inhibit retinal neovascularization in a murine model of proliferative retinopathy. Proc Natl Acad Sci U S A. 1996;93(10):4851-4856.
55. Adamis AP, Shima DT, Tolentino MJ, et al. Inhibition of vascular endothelial growth factor prevents retinal ischemia-associated iris neovascularization in a nonhuman primate. Arch Ophthalmol. 1996;114(1):66-71.
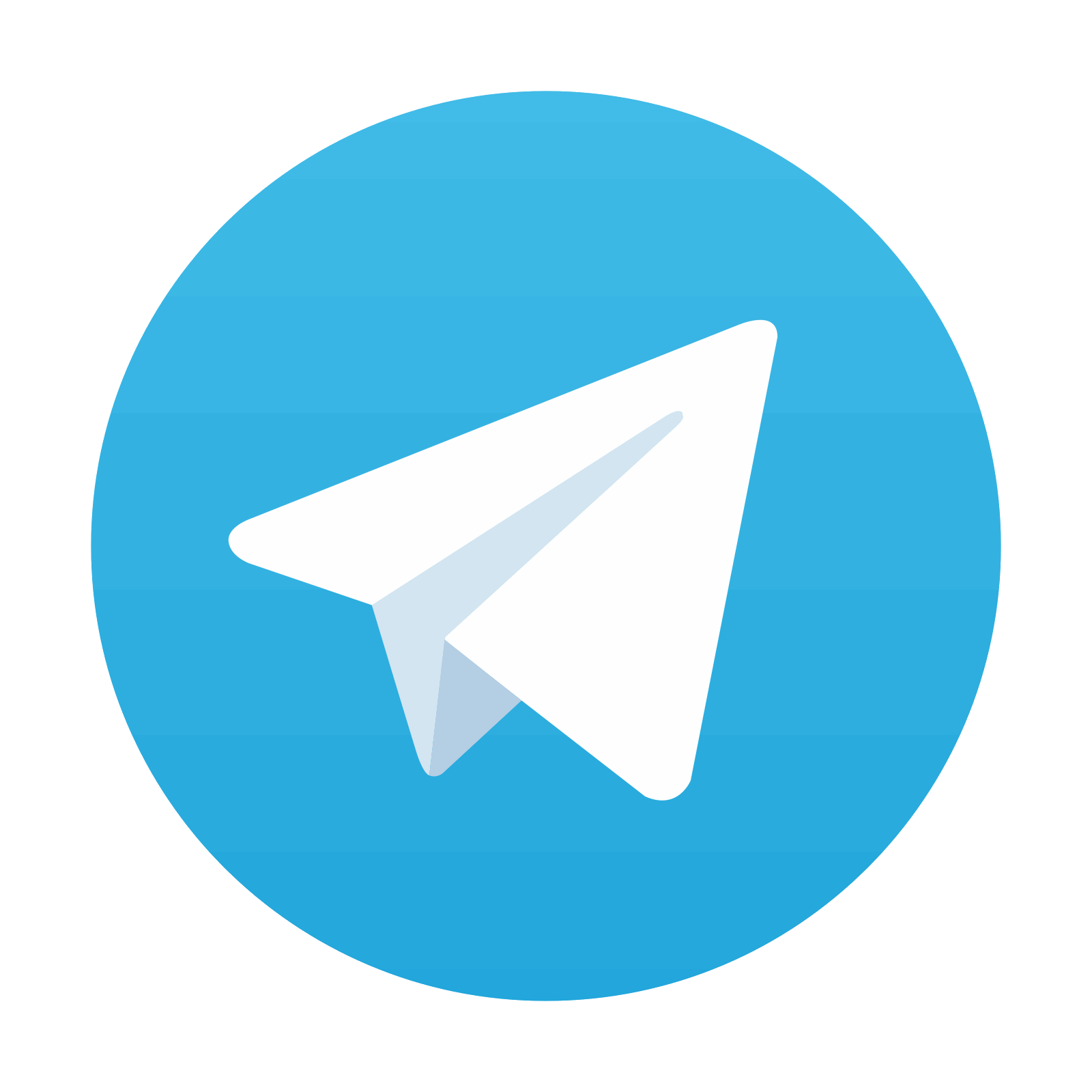
Stay updated, free articles. Join our Telegram channel

Full access? Get Clinical Tree
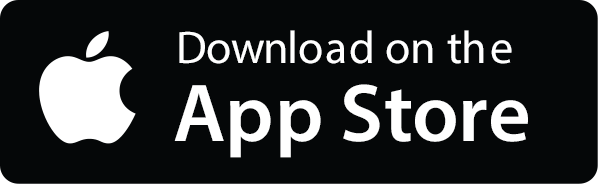
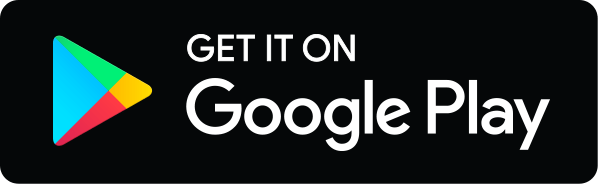