Purpose
To investigate the 20-year efficacy and safety of photorefractive keratectomy (PRK).
Design
Long-term observational case series.
Methods
In the setting of a university hospital, a study population of 42 patients (42 eyes) who had, as part of a randomized prospective trial, undergone PRK 20 years previously were studied. All had received −3.0 or −6.0 diopter (D) corrections with either 5.0 or 6.0 mm optical zones or a multizone treatment. The mean preoperative spherical equivalent refractive error (SEQ) was −5.13 D (range, −2.75 to −8.0 D). The outcome measures included visual acuity, refractive error, corneal topography and axial length.
Results
Between 1 and 20 years there was an increase in mean myopic SEQ of −0.54 D ( P < 0.02). In patients younger than 40 years of age at time of correction, this increase was −0.92 D ( P < 0.002) with an accompanying increase in variance ( P < 0.02), whereas in those older than 40 it was −0.08D ( P = 0.8). In female patients the change was −0.69D ( P < 0.01), while in males it was −0.26D ( P = 0.6). The efficacy index at 20 years was 0.49, and the safety index was 0.97. Corrected distance visual acuity improved between 1 and 20 years ( P < 0.01); 93% of corneas were clear at 20 years; 3 eyes had trace haze. There was an improvement in haze scores between 1 and 20 years ( P < 0.02). Cornea power remained unchanged between 6 months and 20 years ( P = 0.4). Axial length increased by a mean of 0.84 mm ( P < 0.0001). There was no ectasia.
Conclusions
There was a slight but significant increase in myopic SEQ after PRK between 1 and 20 years, particularly in those under 40 at the time of treatment and in female patients. Corneal power remained unchanged, but axial length increased. The procedure was safe, with no long-term sight-threatening complications and with improvements in corrected distance visual acuity and corneal transparency with time.
It has been 25 years since the first excimer laser refractive procedures were performed. Initial studies utilizing photorefractive keratectomy (PRK) were aimed at the correction of low to moderate myopia. The original algorithms used 4.0 and 5.0 mm optical zones on the basis of maximal conservation of stroma, given that less tissue removal is required for smaller ablation diameters in any given dioptric correction. Although these studies demonstrated the successful use of the excimer laser to achieve a refractive change, a number of problems were apparent. Initial overcorrection led to a delay in visual rehabilitation, and reduced predictability with high diopter corrections limited efficacy. In addition, night vision disturbances (haloes) could compromise driving, and the occurrence of transient loss of corneal transparency (haze) was not infrequent. To address these problems, especially haloes, clinical trials were undertaken to enlarge the optical zone. An increases to 6.0 mm not only reduced the problems associated with mesopic vision but also diminished the initial hyperopic overcorrection, decreased the variance in refractive outcome, and reduced haze. The development of large optical zone treatments with smooth ablation profiles through flying-spot technology and the modulation of wound healing using mitomycin-C for high corrections has resulted in excellent refractive and visual outcomes with modern PRK and surface treatments, comparable to those with laser in situ keratomileusis (LASIK). Published studies comparing PRK and LASIK demonstrate that not only are the final refractive and visual outcomes comparable, even for corrections of high myopia, but there may be less induction of higher order aberrations with surface techniques. For these reasons, despite the advent of femtosecond laser flap creation and the advantages of LASIK in terms of reduced postoperative pain and speed of recovery, most refractive surgeons still offer excimer surface ablation procedures.
Although the short- and medium-term outcomes of PRK are satisfactory, for any refractive procedure to be viable, the corrections need to be stable for several decades, without long-term complications that may degrade the transparency or biomechanics of the cornea. This has not been the situation with procedures such as radial keratotomy, in which continued biomechanic instability led to progressive peripheral ectasia and hyperopic drift. The use of larger ablation zones in PRK increases both ablation depth and stromal tissue removal. Consequently, increased wound-healing reactions, late regression and delayed complications due to impaired biomechanics may result. In the literature there are long-term follow-up studies of PRK that demonstrate good refractive and biomechanical stability, but these treatments were performed with small optical zones of 5.00 mm or less and with follow-up of 15 years or less. In the present study, to further investigate the long-term efficacy and safety of PRK, we present the outcomes of a series of 42 eyes in 42 patients who underwent PRK 20 years ago as part of a randomized prospective study to investigate the effects of 5.0 mm and 6.0 mm optical zones as well as a multizone treatment. This study represents the longest follow-up of excimer laser procedures to date.
Methods
Subjects
The original study comprised 100 patients (100 eyes) who underwent myopic spherical PRK at our institution between 1992 and 1993, using 5.0 mm, 6.0 mm or a multizone treatment with either −3.0 diopter (D) or −6.0 D corrections as part of an ethics committee-approved (Guy’s Hospital Ethics Committee, London, uK EC92/333) prospective, randomized clinical trial. Prior to participation, patients were counseled to discuss the experimental nature of the procedure. All were older than 18 and had had stability of refraction over the preceding 2 years. Those with ocular pathology, previous anterior segment surgery, diabetes, or connective tissue disorders were excluded. Of the 100 original patients, 41 underwent examination at our institution; 4 agreed to attend for optometric evaluation locally and send the report to us; and 3 patients who attended were excluded from analysis because they had undergone further excimer treatment. Ethics committee approval was obtained for this study (Research Ethics Committee, Northern Ireland, UK 13/NI/01650) and patients gave written, informed consent for the results of their ophthalmic and optometric examinations to be used for this study.
Pre- and Postoperative Evaluation
Pre- and postoperatively, Snellen uncorrected distance acuity and corrected distance visual acuity (CDVA) were measured, and refraction, autorefraction, keratometry, biomicroscopy, tonometry, and mydriatic fundoscopy were performed. Postoperative follow-up occurred at 7 days, 1 month, 3 months, 6 months, 1 year, and 20 years. Patients were questioned regarding symptoms of foreign-body sensation, dryness and quality of night-time vision. Corneal haze was measured by a subjective grading method (Fantes) based on the following criteria: 0 = no haze; 0.5 = trace/just perceptible; 1 = easily seen with slit-lamp; 2 = moderate haze; 3 = pronounced haze, iris details visible; 4 = scarring, iris details obscured. Preoperatively and for the first 12 months following surgery, corneal topography was performed using a first-generation Placido disc videokeratography system (Tomey TMS-1, Cambridge, Massachusetts). At 20 years, topography and corneal wave-front analysis were performed using the Keratron Scout (Optikon, Rome, Italy) Placido disc device and corneal tomography by the Pentacam Scheimpflug camera system (Oculus Optikgeräte, Wetzlar, Germany). Preoperatively, axial length and anterior chamber depth were determined by B-scan ultrasonography. At 20 years, axial length and anterior chamber depth were determined by partial coherence interferometry (IOL Master; Carl Zeiss Meditec, Jena, Germany).
Laser and Treatment Parameters
The UV200 excimer laser (Summit Technology, Waltham, Massachusetts) was used to treat the eyes included in this study. The operative techniques have been described previously. These lasers employed broad-beam and iris-diaphragm technology. All treatments were spherical, using a 5.0 mm (17 patients) or 6.0 mm (18 patients) optical zone or a multizone (7 patients) treatment and were based on the algorithms developed by Munnerlyn and associates. Patients receiving 5.0 mm and 6.0 mm optical zone treatments underwent −3.0 or −6.0 D corrections, and all patients undergoing multizone treatments underwent −6.0 D corrections. The target postoperative refraction was not emmetropia in all cases. The central ablation depth for patients with 5.0 mm zone treatments and undergoing −3.0 D corrections was 27 um, and for −6.0 D corrections, it was 54 um. The central ablation depth for patients with 6.0 mm zone treatments and undergoing −3.0 D corrections was 39 um, and for −6.0 D corrections, it was 78 um. The multizone treatment, which comprised a −5.0 D correction with a 4.6 mm optical zone and a −1.0 D correction with a 6.0 mm zone, had a planned central ablation depth the same as the 5.0 mm zone treatments of 54 um.
Vector Analysis
To investigate vectoral astigmatic change in the manifest refraction, vector analysis was performed in all eyes according to the system described by Retzlaff and associates.
Statistical Analysis
Paired Student t tests were used to compare refractive changes pre- and postoperatively. F-tests were used to test the differences between variances at 1 and 18 years. Multivariate regression analysis and ANOVA tests were used to determine whether variables such as age, refractive correction and gender were acting independently of each other in terms of refractive outcomes. Then χ 2 analysis was used to compare qualitative data such as corneal haze scores. Results, with P < 0.05, were considered statistically significant.
Results
Preoperative Patient Demographics
The mean age of patients who attended the follow-up or sent optometric reports (n = 42) was 58.4 ± 10.04 years (42 to 84 years). The mean age at time of surgery was 38.4 ± 10.04 years (22–64 years). Of the patients, 28 were female and 14 were male. Mean preoperative spherical equivalent refraction (SEQ) was −5.13 ± 1.86 D (−2.75 to −8.00 D) ( Table 1 , Figure 1 , Figure 2 ). Mean preoperative refractive cylindrical correction was −0.54 diopter correction (DC) (0 to −1.75 DC). Mean preoperative decimal equivalent CDVA was 1.1 ± 0.15 (0.67 to 1.2). Mean preoperative topographic simulated keratometry (Sim K) was 43.92 ± 1.3 D (range, 41.54 to 46.61 D).
Pre-Op | 1 Year | 20 Years | P Value Change in SEQ † | P Value Change in Variance † | |
---|---|---|---|---|---|
Whole cohort n = 42 | −5.13 ± 1.86 | −1.18 ± 1.35 | −1.72 ± 1.69 | P < 0.02 | P = 0.1 |
<40 at surgery n = 23 | −5.1 ± 1.76 | −1.21 ± 1.04 | −2.12 ± 1.78 | P < 0.005 | P < 0.02 |
>40 at surgery n = 19 | −5.17 ± 2.02 | −1.14 ± 1.68 | −1.23 ± 1.46 | P = 0.8 | P = 0.6 |
Female patients n = 28 | −5.08 ± 1.92 | −1.16 ± 1.25 | −1.83 ± 1.63 | P < 0.01 | P = 0.2 |
Male patients n = 14 | −5.23 ± 1.78 | −1.22 ± −1.57 | −1.48 ± 1.85 | P = 0.6 | P = 0.6 |
−3.0 D Corrections n = 20 | −3.31 ± −0.54 | −0.39 ± 0.46 | −0.86 ± −1.1 | P < 0.05 | P < 0.0005 |
−6.0 D Corrections n = 22 | −6.8 ± 0.62 | −1.89 ± 1.5 | −2.5 ± 1.78 | P = 0.09 | P = 0.5 |
5.0 mm optical zones n = 17 | −4.78 ± 1.75 | −1.21 ± 1.69 | −1.85 ± 1.85 | P = 0.08 | P = 0.8 |
6.0 mm optical zones n = 18 | −4.68 ± 1.79 | −0.86 ± 1.03 | −1.21 ± 1.46 | P = 0.2 | P = 0.2 |
Multizone treatment n = 7 | −7.16 ± 0.71 | −1.99 ± 0.89 | −2.7 ± 1.59 | P = 0.2 | P = 0.2 |
∗ Female and male patients, −3.0 and −6.0 diopter corrections and 5.0 mm, 6.0 mm and multizone treatment groups.
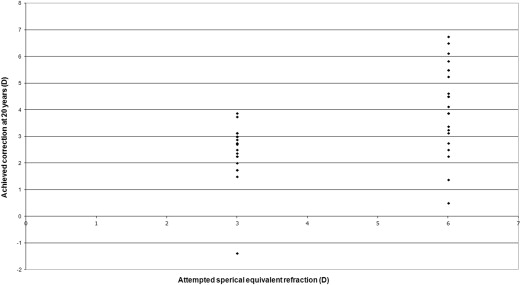
There were no differences between this cohort of 42 patients and the 58 who were part of the original study but who could not be traced or had undergone further excimer laser surgery in terms of age ( P = 0.2); preoperative ( P = 0.3) and 12 months’ refractive errors ( P = 0.2); preoperative and 12-months CDVA ( P = 0.8); preoperative Sim K ( P = 1.0); attempted correction ( P = 0.2); and haze scores at 6 months ( P = 0.1).
Refractive Outcome, Stability and Predictability
Preoperative data
Preoperative mean SEQ for all 42 eyes was −5.13 ± 1.86 D (−2.75 to −8.00 D) ( Table 1 , Figure 1 and Figure 2 ).
In 23 patients younger than 40 years of age at time of correction, mean preoperative SEQ was −5.1 ± 1.76 D (−3.00 to −7.875D), whereas in 19 patients older than 40, mean preoperative SEQ was −5.17 ± 2.02D (−2.75 to −8.0 D). There was no difference between these 2 groups in terms of gender or preoperative refractive error ( P = 1.0) ( Table 1 , Figure 3 ).
In 28 female patients, the mean preoperative SEQ was −5.08 ± 1.92 D (−2.875 to −8.0), whereas in 14 males, the mean preoperative SEQ was −5.23 ± 1.78 D (−2.750 to −7.875 D). There was no difference between these 2 groups in terms of preoperative refractive error ( P = 0.9) and age ( P = 0.3) ( Table 1 , Figure 4 ).
In 20 eyes that received corrections of −3.0 D, the mean preoperative SEQ was −3.31 ± 0.54D (−2.75 to −5.0 D), whereas in 22 eyes that received corrections of −6.0 D, the mean preoperative SEQ was −6.8 ± 0.62 D (−6.0 to −8.0 D). There was no significant difference between these 2 groups in terms of gender or age ( P = 0.9) ( Table 1 , Figure 5 ).
In 17 eyes that received 5.0 mm diameter corrections, the mean preoperative SEQ was −4.78 ± 1.75 D (−3.0 to −7.625), whereas in 18 eyes that received 6.0 mm treatments, the mean preoperative SEQ was −4.68 ± 1.79 D (−2.75 to −7.125 D). There was no difference between these 2 groups in terms of gender, age or preoperative SEQ ( P = 1.0) ( Table 2 , Figure 6 ).
Pre-Op | 1 Year | 20 Years | P Value Change in SEQ ∗ | P Value Change in Variance ∗ | |
---|---|---|---|---|---|
5.0 mm optical zone −3D n = 10 | −3.46 ± 0.69 | −0.31 ± 0.42 | −1.21 ± 1.26 | P < 0.04 | P < 0.006 |
5.0 mm optical zone −6D n = 7 | −6.66 ± 0.65 | −2.48 ± 2.04 | −2.77 ± 2.25 | P = 0.7 | P = 0.9 |
6.0 mm optical zone −3D n = 10 | −3.15 ± 0.29 | −0.475 ± 0.5 | −0.51 ± 0.83 | P = 0.9 | P = 0.2 |
6.0 mm optical zone −6D n = 8 | −6.59 ± 0.42 | −1.34 ± 1.34 | −2.08 ± 1.64 | P = 0.3 | P = 0.7 |
Multizone treatment n = 7 | −7.16 ± 0.71 | −1.99 ± 0.89 | −2.7 ± 1.59 | P = 0.2 | P = 0.2 |
In 7 eyes that received multizone treatments, all received −6.0 D corrections with a mean preoperative SEQ of −7.16 ± 0.71 D (−6.375 to −8.0 D). There were no differences between the multizone patients the 5.0 mm and 6.0 mm patients receiving −6.0 D corrections in terms of gender, preoperative SEQ ( P = 0.2) or age ( P = 0.5) ( Table 2 , Figure 7 ).
Postoperative data
Mean postoperative SEQ at 1 year for all 42 eyes was −1.18 ± 1.35D (+1.75 to −5.75 D). At 20 years it was −1.72 ± 1.69D (+1.5 to −4.625 D). This increase in myopic SEQ of −0.54 ± 1.33 D (+2.25 to −3.625) was significant ( P < 0.02) ( Table 1 , Figure 1 , Figure 2 ). At 12 months, 19 eyes (45%) were within ±0.5 D and 27 eyes (64%) were within ±1 D of the intended correction, whereas at 20 years, 15 eyes (36%) were within ±0.5 D, and 23 eyes (55%) were within ±1 D of the intended correction. Between 1 and 20 years, there was no statistical change in variance ( P = 0.1). Analysis of patients who had refractive shifts (myopic or hyperopic) in SEQ between 1 and 20 years greater than 0.75 D (n = 21), compared to those eyes in which the change was less than 0.75 D (n = 21), revealed no differences in terms gender or age. The eyes with refractive shifts greater than 0.75 D had greater preoperative refractive error ( P < 0.001), received higher PRK corrections ( P < 0.001) and more commonly received 5.0 mm or multizone treatments than 6.0 mm optical zone treatments ( P < 0.02).
Table 1 and Table 2 and Figures 1–8 show the results for the subgroups within our cohort in terms of sex, age, degree of attempted refractive correction, and optical zone treatment size and configuration. Between 1 and 20 years, a statistically significant increase in myopic SEQ was found in patients younger than 40 years of age at the time of correction ( P < 0.005) but not in those older than 40 ( P = 0.8); in females ( P < 0.01) but not in males ( P = 0.6); and for −3.0 D corrections. Multivariate regression analysis showed only a weak trend with age and refractive change between 1 and 20 years, which was insignificant ( P = 0.2), as was the trend for gender and refractive change ( P = 0.4). A statistically significant increase in variance between 1 and 20 years was found in patients younger than 40 years of age at time of correction and in those undergoing −3.0 D corrections ( Table 1 , Table 2 ).
Refractive astigmatism
The mean preoperative refractive cylindrical correction was −0.54 DC at 69 degrees (range, 0 to −1.75 DC). At 20 years it was −0.71 DC at 92 degrees (range, +0 to −2.75 DC). Vector analysis demonstrated a 0.76 ± 0.48DC change.
Visual acuity
Uncorrected distance visual acuity
At 20 years, 8 (19%) of eyes had Snellen decimal equivalents uDVA of 20/20 or better; 16 (38%), 20/30 or better; and 21 (50%) 20/40 or better. At 20 years, the efficacy index (postoperative uCVA/preoperative CDVA) was only 0.49.
Corrected distance visual acuity
At 20 years, CDVA was unchanged or improved compared to preoperative levels in 31 (74%) of patients; was unchanged in 24 (56%); was improved by 1 line in 5 (13%); and was improved by 2 lines in 2 (5%) ( Figure 9 ). It was reduced by 1 line in (17%) and by 2 lines in (5%). There were 2 eyes (5%) that lost more than 2 lines of CDVA. In 1, it was the result of advanced glaucoma (4 lines) and in the other, it was cataract (3 lines). Between 1 and 20 years, there was a statistically significant improvement in CDVA ( P < 0.02). The safety index (postoperative CDVA/preoperative CDVA) was 0.97.
Corneal haze
Typically, corneal haze appeared during the first month after surgery, was maximal at 3 to 6 months, and declined thereafter ( Figure 10 ). Over 20 years, haze continued to decline. Three eyes (7%) had trace haze (grade 0.5) at 20 years. Between 1 and 20 years there was a statistically significant reduction in haze scores ( P < 0.0001).
