TABLE 7-1 ANTIDEPRESSANT AGENTS | ||||||||||||||||||||||||||||||||||||||||||||||||||||||||||||||||||||||||||||||||||||||||||||||||||||||||||||||||||||||||||||||||||||||||||||||||
---|---|---|---|---|---|---|---|---|---|---|---|---|---|---|---|---|---|---|---|---|---|---|---|---|---|---|---|---|---|---|---|---|---|---|---|---|---|---|---|---|---|---|---|---|---|---|---|---|---|---|---|---|---|---|---|---|---|---|---|---|---|---|---|---|---|---|---|---|---|---|---|---|---|---|---|---|---|---|---|---|---|---|---|---|---|---|---|---|---|---|---|---|---|---|---|---|---|---|---|---|---|---|---|---|---|---|---|---|---|---|---|---|---|---|---|---|---|---|---|---|---|---|---|---|---|---|---|---|---|---|---|---|---|---|---|---|---|---|---|---|---|---|---|---|
|
Genetic
Pharmacogenetics
Neuroanatomical
Neurotransmitter and related hypotheses
Monoamine
Interactional
Second messenger
Membrane and cation
Biological rhythms
Neuroendocrine
Immunological
requires more than statistical association (40). Thus, study populations must be homogeneous; positive and negative predictive values must be sufficiently high; and the results justify the costs of testing.
Prefrontal cortex (PFC)
Striatum
Hippocampus
Other related areas
Hippocampus
Cerebral cortex
Amygdala
Lower brainstem center (which controls sympathetic output)
Brain tyrosine hydroxylase and NE
Postsynaptic β-adrenergic receptor sensitivity and density
The basal firing rate of NE neurons in the locus coeruleus
catecholamine receptors secondary to decreased NE availability. This reasoning is consistent with the original hypothesis of diminished NE functioning, with antidepressants returning receptors to a more normal state of sensitivity. Siever and Davis (62) further elaborated on this concept by suggesting the possibility of dysregulation in the homeostatic mechanisms of one or more neurotransmitter systems, culminating in an unstable or erratic output.
The lower CSF HVA levels in some depressed patients
An increased incidence of depression in Parkinson disease as well as in patients receiving DA-depleting or antagonistic agents
The antidepressant effect of agents that enhance DA transmission
The ability of various classes of antidepressants and ECS to enhance DA effects in animal models
Decreased 5-HT uptake in the platelets (Vmax) of depressed patients is linked to a decrease in the number of platelet imipramine binding sites (80).
Blunting of the maximal prolactin response to intravenous tryptophan (the precursor of 5-HT) is found in depressed patients. Similar results have also been observed with fenfluramine and m-chlorophenylpiperazine (mCPP) (81).
p-Chlorophenylalanine, which decreases 5-HT synthesis, reverses the clinical efficacy of antidepressants (82).
Depletion of plasma tryptophan precursors may reverse antidepressant-induced remissions (83).
Tryptophan and 5-hydroxy’tryptophan, the precursors of 5-HT, may have antidepressant effects, alone or in combination with other drugs (84).
Antidepressants reduce 5-HT receptor number but not their affinity (85), in a manner analogous to β-adrenergic receptor downregulation.
Electroconvulsive therapy (ECT) potentiates prolactin response to thyrotropin-releasing hormone (TRH), which is mediated by serotonin (86).
ECS enhances 5-HT2 receptor functional activity and binding characteristics in postmortem studies on animals (87).
Frontolimbic 5-HT2A receptor binding may identify vulnerability to mood disorders (88).
5-HTT binding potential in the amygdala and midbrain is lower during a major depressive episode (MDE) (89).
Blocking the reuptake transport mechanism (90)
Antagonizing specific receptor subtypes (91)
Modulating presynaptic receptors
GABA into the hippocampus reversed or prevented its development (102). Martin et al. (103) found that GABA-B receptors are downregulated in the frontal cortex of animals with learned helplessness and are normalized by successful treatment with chronic TCA administration. A recent study in depressed patients using proton magnetic resonance spectroscopy (MRS) indicated that reduced GABA in the occipital cortex may predict treatment resistance (104). Other human data include
GABA concentrations are reduced in the CSF, blood, and postmortem brains of depressed patients when compared to controls (105)
Antidepressant treatment reduces the ability of 3H-flunitrazepam to bind to the benzodiazepine (BZD) binding site
Selective serotonin reuptake inhibitors (SSRIs) and ECT increase cortical GABA in depressed subjects (106,107)
Valproate (an indirect GABA agonist), via the inhibition of GABA transaminase, may be effective in treating bipolar depression (110)
Fengabine (α-GABA agonist) may benefit unipolar depression (111)
5,6-Dihydroxytryptamine lesions of the serotonin nuclei are known to attenuate the reduction in β-noradrenergic receptor binding induced by chronic tricyclic treatment (118)
6-Hydroxydopamine lesions of the NE nuclei in the dorsal and ventral bundles, as well as in the locus coeruleus, block the enhanced locomotor responses to quipazine after repeated ECS
REM density) found in depression. Consistent with this theory is evidence that ECT
Decreases brain ACh levels
Increases activity of choline acetyltransferase, the enzyme most prominently involved in ACh breakdown
Causes release of CSF ACh
Produces cholinergically mediated electroencephalographic (EEG) slowing following a series of treatments
Causative
Phenomenological (expressive)
Epiphenomenological (possible useful state markers)
pathway, which in turn leads to regulation of cAMP-dependent protein kinase and subsequently to activation of the cAMP response element binding protein (CREB).
A decrease in total sleep time
An increase in sleep onset latency
A decrease in the arousal threshold
An increase in wakefulness
Terminal insomnia (early morning awakening)
REM-related phenomena
A decrease in REM onset latency An increase in REM density
A redistribution of REM sleep to earlier in the sleep phase
Depression may alter immunological function
An unidentified infectious process (e.g., viral) may induce affective disturbances
The neuronal reuptake pump for a neurotransmitter
A specific neurotransmitter receptor subtype
A subunit of an ion channel
Maintain or improve efficacy
Widen the therapeutic index
Improve tolerability profiles
Reduce pharmacodynamically or pharmacokinetically mediated drug-drug interactions
CYP isoenzyme(s) responsible for their metabolism
Their ability to inhibit CYP isoenzyme activity
Elimination half-lives
Linear versus nonlinear pharmacokinetics
Changes in plasma drug levels as a function of age and gender
SSRIs. Its levels can be up to 100% greater in physically healthy individuals older than 65 years than in younger individuals. For this reason, the recommendation is to start paroxetine at half the usual dose and adjust upward more slowly in elderly patients. Age-related changes in SSRI plasma levels are important to monitor because elderly patients are likely to be on concomitant medications and effects on specific CYP isoenzymes are concentration dependent (see Chapter 15).
The capsules should not be chewed or divided
The contents should not be removed and sprinkled on food or dissolved in juice
Conditions that significantly delay gastric outflow could cause erratic and incomplete absorption
Conditions that appreciably increase the pH of the stomach could lead to earlier breakdown of the enteric coating and quicker absorption than usual
When taken in the morning with food, the extent of absorption is decreased by approximately 10%. When taken in the evening with food, there is a 3-hour delay in absorption and a one-third decrease in the AUC, or conversely a one-third increase in an apparent clearance compared to the morning dose. To put these numbers in perspective, the evening effect of food is comparable to the difference permitted between generic formulations of the same drug.
Hydroxybupropion
Threohydrobupropion
Erythrohydrobupropion
N-demethylation (CYP 1A2, 2C19, and 3A3/4)
N-oxidation (CYP 2D6)
Aromatic hydroxylation (CYP 2D6)
The majority (i.e., greater than 90%) are normal or “extensive” metabolizers in whom levels of 0.5 to 1.5 ng/mL/mg/day develop.
Members of a smaller group (i.e., 5% to 10%) are genetically deficient in CYP 2D6 and, hence, are poor metabolizers in whom plasma drug levels in the range of 4.0 to 6.0 ng/mL/mg/day develop.
An even smaller group of patients (i.e., 0.5%) are ultrarapid metabolizers of TCAs in whom levels of less than 0.5 ng/mL/mg/day develop (194).
The incidence of seizures is dose related and, hence, concentration related.
Seizures typically occur within days of a dose change and a few hours after the last dose, suggesting that peak plasma concentrations play a role.
Individuals with lean body mass (i.e., smaller volume of distribution), such as anorexic-bulimic patients, are at increased risk.
Amitriptyline (80 to 150 ng/mL)
Desipramine (110 to 160 ng/mL)
Imipramine (≤265 ng/mL)
Nortriptyline (50 to 150 or 170 ng/mL, depending on the study)
A blood sample is then drawn 10 to 12 hours after the last dose to ensure that absorption and distribution of the drug are complete and because virtually all the data on optimal concentration ranges are based on this postdose time interval.
None or a poor relationship between plasma level and therapeutic response
A threshold for therapeutic response, such that below this level, there is less likelihood of response and above it, there is a better chance for response
A threshold for toxicity such that above this level, the potential for increased adverse effects outweighs the likelihood of further therapeutic gain
Plasma levels are in the usual optimal range, but there is insufficient clinical response: try another agent, an augmentation strategy, or ECT (see also “Alternative Treatment Strategies” later in this chapter)
Plasma levels are low and there is insufficient response: increase the dose
Plasma levels are high and there is insufficient clinical response or there are severe adverse effects: reduce the dose or try another agent
in antidepressant clinical trials. Increasingly, however, there is concern regarding the adequacy of these scales to assess medication-related changes in depression (222,223). In this context, self-report scales (e.g., BDI; IDS-SR) and functional outcome measures are increasingly used in clinical practice to better assess medication impact (i.e., measurement-based care).
TABLE 7-2 FIRST-GENERATION ANTIDEPRESSANTS VERSUS PLACEBO: ACUTE TREATMENT | |||||||||||||||||||||||||||||||||||||||||||
---|---|---|---|---|---|---|---|---|---|---|---|---|---|---|---|---|---|---|---|---|---|---|---|---|---|---|---|---|---|---|---|---|---|---|---|---|---|---|---|---|---|---|---|
|
Hospitalization
Functional impairment as assessed by a scale such as the Global Assessment Scale
Absolute symptom severity as assessed by a standardized rating assessment
Depressive subtype, particularly psychotic or melancholic as defined in Diagnostic and Statistical Manual of Mental Disorders, 4th edition, Text Revision (DSM-IV-TR)
effectiveness study involved 4,041 outpatients with unipolar major depression (230). The primary outcome was remission [i.e., not response as in randomized controlled trials (RCTs)] defined by a final score of ≤7 on the 17-item HDRS. The initial phase was an open-label trial with citalopram (mean exit dose = 41.8 mg/day) for 12 weeks. Those who did not achieve remission or did not tolerate treatment could then participate in three additional sequential levels involving various switch or augmentation options until they met remission criterion.
Citalopram. (Celexa)
Escitalopram (Lexapro)
Fluoxetine (Prozac)
Fluvoxamine (Luvox)
Paroxetine (Paxil)
Sertraline (Zoloft)
Less expense
Less time
Less risk of developing a nonmarketable product at the end of the process
The pharmaceutical company is able to maintain and perhaps grow its franchise in the marketplace
comparative antidepressants, one important limitation is that the studies were generally based on depressed outpatients.
TABLE 7-3 SSRIS VERSUS PLACEBO: ACUTE TREATMENT | ||||||||||||||||||||||||||||||||||||||||||||||||||||||
---|---|---|---|---|---|---|---|---|---|---|---|---|---|---|---|---|---|---|---|---|---|---|---|---|---|---|---|---|---|---|---|---|---|---|---|---|---|---|---|---|---|---|---|---|---|---|---|---|---|---|---|---|---|---|
|
TABLE 7-4 SSRIS VERSUS FIRST-GENERATION ANTIDEPRESSANTS: ACUTE TREATMENT | ||||||||||||||||||||||||||||||||||||||||||||||||||||||
---|---|---|---|---|---|---|---|---|---|---|---|---|---|---|---|---|---|---|---|---|---|---|---|---|---|---|---|---|---|---|---|---|---|---|---|---|---|---|---|---|---|---|---|---|---|---|---|---|---|---|---|---|---|---|
|
and there was no placebo control. There also have been four studies and one meta-analysis of European clinical trials that found no difference in antidepressant efficacy between several different SSRIs and several different tertiary amine TCAs in patients hospitalized for major depression (251,252,253,254 and 255). Finally, there are two relatively small studies showing that fluoxetine and fluvoxamine both had antidepressant efficacy superior to placebo in patients with melancholia (256,258). Another larger study failed to find a difference between paroxetine and amitriptyline in treating such patients (258).
Ease of administration
Lack of cardiotoxicity
Single mechanism of action
Sexual dysfunction
Potential for adverse drug interactions mediated through CYP450 and P-glycoprotein systems
Single mechanism of action
predetermined doses, regardless of need, and dosage is adjusted rapidly to that predetermined dose. This approach is in contrast to clinical practice, wherein patients are typically started on the lowest usually effective dose and only adjusted upward as needed and tolerated. Such adjustment is often done much more gradually than in a fixed dose study, and thus the patient may adjust to dose-dependent adverse effects. Most patients, for example, will adapt to the nausea produced by drugs that potentiate 5-HT, such as the SSRIs and venlafaxine.
Such studies are technically difficult
Such studies involve high risk (i.e., answers may not be favorable to the sponsor)
Trials during clinical development are designed to meet registration requirements rather than to answer such questions
An ascending dose-antidepressant response curve, with possible greater overall efficacy at the higher doses
Lack of affinity for muscarinic, histaminergic, α-adrenergic, dopaminergic, and opioid receptors
Minimal effects on any of the major CYP isoenzymes in contrast to antidepressants such as fluoxetine, fluvoxamine, or paroxetine
Possible increase in blood pressure
Sexual dysfunction
Wide variability in effective dose
Starting and target dose of 50 mg/day
Lack of affinity for muscarinic, glutaminergic, α-adrenergic, dopaminergic, and opioid receptors
Minimal pharmacokinetic effects mediated by the CYP450 system
Adverse effects at 50 mg/day demonstrated placebo-level discontinuation rates
Efficacy trials were inconsistent in terms of ideal dosing
Sexual dysfunction
Stress urinary incontinence
Fibromyalgia
Generalized anxiety disorder
twice daily (n = 95 and 93, respectively) or placebo (n = 93) for 8 weeks. In the fifth study, subjects who entered the 8-week trial were assigned to placebo (n = 70) or duloxetine (n = 70) given in a forced titration regimen (i.e., 20 µg twice a day to 60 µg twice a day over the first 3 weeks). In all five studies, duloxetine was superior to placebo (and comparable to SSRIs used in three of these trials) in reducing depressive symptoms as measured by the 17-item HDRS. Analysis of these studies did not reveal any relationship between antidepressant efficacy and age, gender, or race, although the vast majority of patients in these trials were Caucasians.
Equal impact on 5-HT and NE reuptake across dosing range
Improves pain symptoms and fibromyalgia syndrome
Effective for generalized anxiety disorder
Safe in overdose
Does not cause clinically significant elevation in blood pressure or heart rate
Lack of affinity for muscarinic, histaminergic, α-adrenergic, dopaminergic, and opioid receptors
May worsen narrow-angle glaucoma
May elevate liver enzymes
Gastrointestinal symptoms: nausea, constipation
May cause sexual side effects or urinary hesitancy
May produce discontinuation syndrome
May produce clinically relevant drug interactions (e.g., via CYP 2D6)
when using the paradigm for amphetamine-like drugs. The combined plasma concentration of bupropion and its three active metabolites is consistent with the conclusion that the inhibition of both of these uptake pumps likely occurs with clinically relevant doses.
TABLE 7-5 SPECIFIC ANTIDEPRESSANTS VERSUS PLACEBO: ACUTE TREATMENT | ||||||||||||||||||||||||||||||||||||||||||||||||||||||||||||||||||||||||||||
---|---|---|---|---|---|---|---|---|---|---|---|---|---|---|---|---|---|---|---|---|---|---|---|---|---|---|---|---|---|---|---|---|---|---|---|---|---|---|---|---|---|---|---|---|---|---|---|---|---|---|---|---|---|---|---|---|---|---|---|---|---|---|---|---|---|---|---|---|---|---|---|---|---|---|---|---|
|
TABLE 7-6 SECOND-GENERATION VERSUS FIRST-GENERATION ANTIDEPRESSANTS: ACUTE TREATMENT | ||||||||||||||||||||||||||||||||||||||||||||||||
---|---|---|---|---|---|---|---|---|---|---|---|---|---|---|---|---|---|---|---|---|---|---|---|---|---|---|---|---|---|---|---|---|---|---|---|---|---|---|---|---|---|---|---|---|---|---|---|---|
|
Pharmacologically unique relative to other conventional antidepressants; most closely resembles the action of psychostimulants
May benefit patients who historically have not responded to TCAs (313)
Relatively free of sexual adverse effects, in contrast to the SSRIs or venlafaxine (314)
Useful in conditions not typically responsive to other antidepressants (e.g., adult attention deficit hyperactivity disorder [ADHD] (315) and smoking cessation (316))
Not sedating, low incidence of anticholinergic adverse effects, does not cause weight gain, and minimal effects on intracardiac conduction
Possibly a less robust antidepressant effect
Possible increase in suicidality in younger patients
Increased risk for seizures (e.g., with overdose)
Contraindicated in those with eating disorders
adjust the dosage within the dose range based on efficacy and tolerability. Hence, dosages for patients who failed to respond should have been adjusted to the highest tolerated dose. Such a design produces a curvilinear dose-response curve with a diminution in response at the highest level because dosages for nonresponders are adjusted upward to the highest tolerated dose. In addition, there is the problem of dropouts, which occurs because of the acute nuisance adverse effects at high doses, as discussed in the sections on SSRIs and venlafaxine. Nonetheless, this result indicates that there is generally no advantage to exceeding 500 mg/day of nefazodone.
An ascending dose-antidepressant response curve in contrast to SSRIs
Perhaps a more benign adverse effect profile, particularly with regard to activation and sexual dysfunction
A potentially more rapid onset of anxiolytic effect
A lack of blood pressure elevation, in contrast to venlafaxine
Need for a twice-a-day dosing schedule
Need for dose adjustment
Rare but serious hepatotoxicity
Produces substantial inhibition of CYP 3A3/4, leading to the potential for adverse pharmacokinetic interactions
Sedation; useful for anxiety, agitation, hostility, and dysomnia
Relative safety with overdose
No anticholinergic effects
No quinidine-like effect
Not antiarrhythmic and may induce or exacerbate ventricular arrhythmia (not proven)
Priapism
Postural hypotension
α2-adrenergic receptors (327). The latter effect increases NE release by a direct effect on the presynaptic α2-adrenergic autoreceptor and indirectly increases serotonin release via the tonic effect on the adrenergic input to raphe neurons. In addition to these mechanisms of action, mirtazapine and mianserin also block histamine and specific 5-HT receptors (i.e., 5-HT2A, 5-HT2C, 5-HT3) but have minimal affinity for muscarinic or α1-adrenergic receptors and do not inhibit either NE or 5-HT uptake pumps.
Sedation; may be useful for agitation and hostility in geriatric patients
Safety in overdose
Minimal anticholinergic effects
No quinidine-like effect
No blood pressure effects, including orthostatic hypotension
Daytime sedation
Increased appetite
Weight gain
Transient neutropenia (typically presents and resolves within first 6 weeks of treatment)
Transient mild elevations in liver function tests, particularly serum glutamic-pyruvic transaminase (SGPT; typically presents and resolves within first 6 weeks of treatment)
Rare adverse event of severe neutropenia (3 out of 2,796 patients); uncertain causal relationship to mirtazapine treatment
Chronic pain
Migraines
Insomnia
TABLE 7-7 FIRST-GENERATION ANTIDEPRESSANTS: CONTROLLED, DOUBLE-BLIND STUDIES | ||||||||||||||||||||||||||||||||||||||||||||||||||||||||||||||||||
---|---|---|---|---|---|---|---|---|---|---|---|---|---|---|---|---|---|---|---|---|---|---|---|---|---|---|---|---|---|---|---|---|---|---|---|---|---|---|---|---|---|---|---|---|---|---|---|---|---|---|---|---|---|---|---|---|---|---|---|---|---|---|---|---|---|---|
|
Dual mechanism of action may provide more robust efficacy (tertiary amines).
Generic formulations are less expensive.
Amoxapine may be more effective for psychotic depression.
Clomipramine may benefit refractory obsessive-compulsive disorder.
Amitriptyline may be helpful for pain disorders.
Lethal in overdose
Anticholinergic effects
May lower seizure threshold
May cause hypotension
Atypical depression (331)
Mixed anxiety and depressive disorders
Panic disorder, with or without agoraphobia
Eating disorders, particularly bulimia (because of required dietary restrictions, however, this may not be a feasible therapy in many)
(e.g., tyramine and tryptamine) are comparably deaminated by both types.
TABLE 7-8 MAOIS VS. PLACEBO: ACUTE TREATMENT | ||||||||||||||||||||||||||||||||||||
---|---|---|---|---|---|---|---|---|---|---|---|---|---|---|---|---|---|---|---|---|---|---|---|---|---|---|---|---|---|---|---|---|---|---|---|---|
|
tyramine-induced hypertensive crisis. Selegiline is an irreversible but relatively selective inhibitor of MAO-B. An oral preparation, currently marketed as a treatment for Parkinson’s disease, produces meaningful inhibition of MAO-B but not MAOA. Studies suggest that the selegiline transdermal system (STS) may minimize drug-food interactions. Normal volunteers given STS (20 mg/20 cm2) patch daily for 13 days were able to consume more than 300 mg of tyramine without experiencing any clinically relevant increases in systolic or diastolic blood pressure (340). Two, double-blind trials found that the same STS regimen was statistically superior to placebo in the treatment of outpatients with major depression, but effect sizes were modest (334,341). The only adverse effect greater with the STS than placebo was application-site reactions. Thus, physicians using this MAOI formulation for depression may appreciably lower risk of hypertensive crisis by
TABLE 7-9 CLINICAL CHARACTERISTICS OF MAOIS | ||||||||||||||||||||||||||||||||||||||||||||||||
---|---|---|---|---|---|---|---|---|---|---|---|---|---|---|---|---|---|---|---|---|---|---|---|---|---|---|---|---|---|---|---|---|---|---|---|---|---|---|---|---|---|---|---|---|---|---|---|---|
|
Minimizing inhibition of gastrointestinal MAO-A activity
Circumventing first-pass hepatic metabolism, which increases the parent compound to metabolite ratio
Brofaromine
Cimoxatone
Toloxatone
Impact three neurotransmitters (i.e., 5-HT, NE, and DA)
With newer agents, antidepressant effect attributed to enzyme MAO-A or MAO-B only; therefore, propensity to cause tyramine potentiation is reduced
Possibly more effective in endogenous and atypical depression
No nonspecific biochemical or pharmacological actions
Drug-drug and drug-food interactions
Effective dose is variable
Adverse effects—loss of appetite, nausea, and other gastrointestinal disturbances; insomnia
Shorter duration of action
Idiosyncratic responses at times
TABLE 7-10 LITHIUM VS. PLACEBO: ACUTE TREATMENT | ||||||||||||||||||||||||||||||||||||||||
---|---|---|---|---|---|---|---|---|---|---|---|---|---|---|---|---|---|---|---|---|---|---|---|---|---|---|---|---|---|---|---|---|---|---|---|---|---|---|---|---|
|
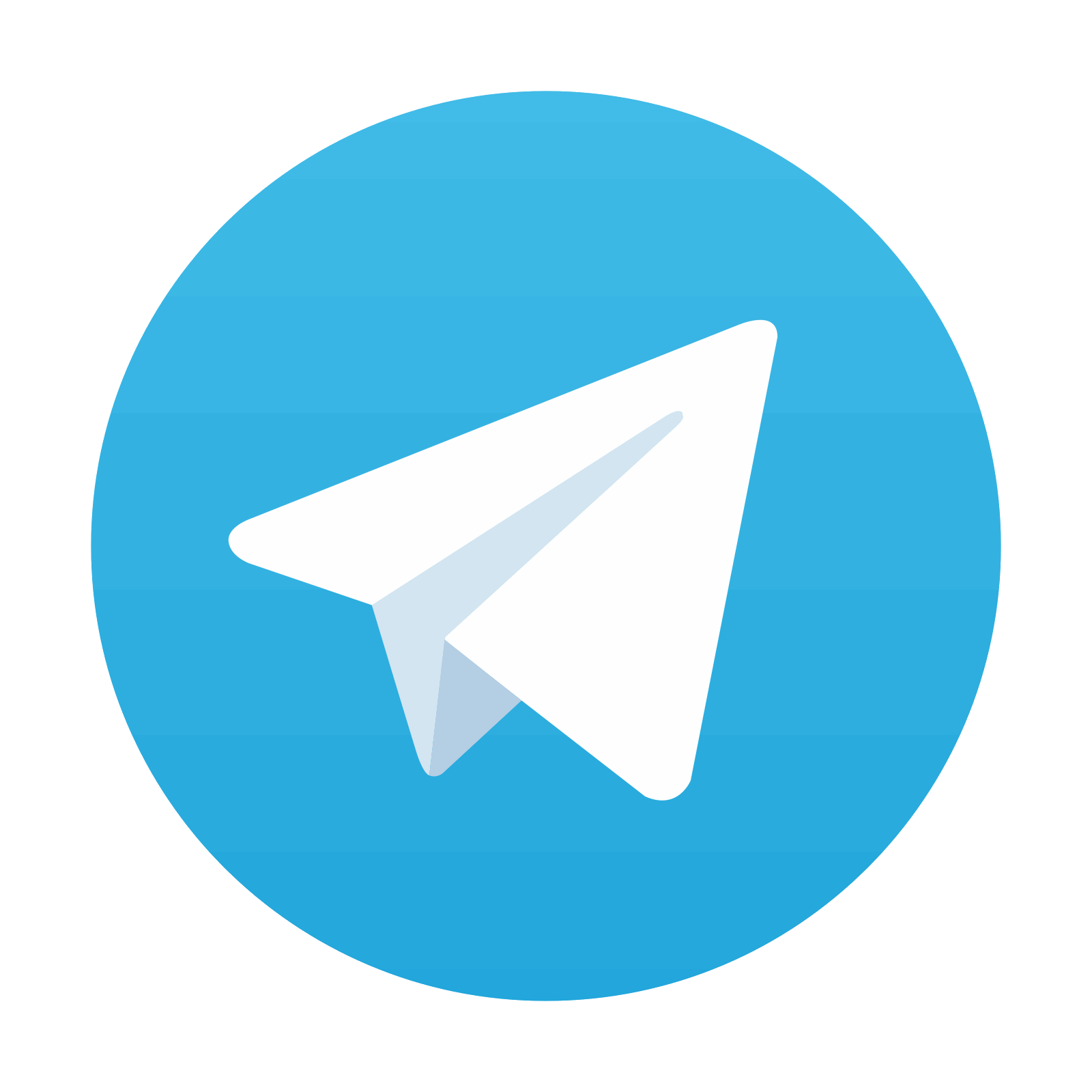
Stay updated, free articles. Join our Telegram channel

Full access? Get Clinical Tree
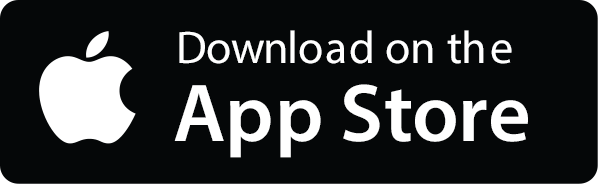
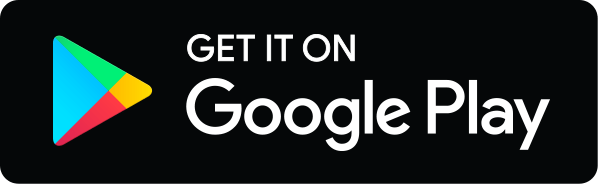
