The management of choroidal neovascular (CNV) membranes in age-related macular degeneration (AMD), ocular histoplasmosis syndrome, myopia, and uveitis has advanced greatly in the past few years. In general terms, there has been a progression from nihilistic observation to laser ablation to submacular surgery, photodynamic therapy (PDT) with and without intravitreal steroids to modern anti–vascular endothelial growth factor (VEGF) therapy.
Many potential treatments have been promoted by positive pilot studies ultimately followed by randomized clinical trials showing no significant efficacy. Examples of this sequence include alpha one interferon, thalidomide, brachytherapy, anecortave acetate, transpupillary thermotherapy for occult CNV, and external beam radiation.
Pegaptanib sodium (Macugen) and PDT (Visudyne) therapy resulted in modest slowing in the rate of visual loss but rarely resulted in visual improvement. Ranibizumab (Lucentis), a humanized Fab fragment of an anti-VEGF antibody, was the first medication to achieve reliable visual gains in a significant percentage of patients. Visual results in several pivotal multicenter, randomized clinical trials were simply outstanding compared to the results previously achieved with earlier therapies: 95% of patients avoided moderate visual loss (2 ETDRS lines or 25 letters), 30% to 40% of the patients experienced improved vision, and 75% achieved true stability. Phillip Rosenfeld introduced the concept off-label use of bevacizumab (1,2) (Avastin), a related, full-length anti-VEGF antibody, as a significantly less expensive alternative to Lucentis. Retrospective, prospective, and, more recently, small randomized trials and a large amount of anecdotal experience using Avastin have demonstrated similar outcomes to Lucentis. The National Eye Institute–sponsored Comparisons of Age-Related Macular Degeneration Treatments Trials (CATT) is a head-to-head comparison of Lucentis to Avastin and has both protocol and PRN treatment arms for both drugs.
Most patients are currently treated with monotherapy with either bevacizumab or ranibizumab. There are patients were combination with other therapies (like laser) can be entertained.
FOCAL THERMAL LASER ABLATION
The goal of laser therapy is to achieve complete ablation of the CNV complex. Laser therapy produces concurrent overlying retinal ablation with an immediate scotoma.
Photocoagulation of juxtafoveal lesions has been shown to produce greater than 50% recurrence rates, frequently on the foveal side (3). Many patients present with subfoveal lesions; therefore, laser treatment of these lesions was investigated in the Macular Photocoagulation Study (MPS) as well. It was shown that treated patients suffer an immediate loss of greater than three lines of vision but have slightly better vision after 18 months compared to patients who did not receive laser therapy. Few physicians ever became comfortable with causing immediate loss of central vision, and this treatment is no longer performed since Lucentis and Avastin became available.
Laser treatment can still be considered for extrafoveal CNVs if they are at a substantial distance from the fovea and the total size of the lesion (and the secondary scotoma) is small. In other words, the smaller and more peripheral the lesion is, the more amenable it is to laser therapy. It is important for the lesion to have clearly defined borders on angiography to guarantee that all the CNV is ablated whenever laser is preformed.
The advantage of laser above anti-VEGF therapy is single-session treatment with long-term control. The authors often recommend injection of Avastin or Lucentis concurrently with focal laser therapy for extrafoveal lesions with the hopes of decreasing recurrence rates.
PREVENTION STRATEGIES
Dietary Supplementation
Oral antioxidants such as zinc, beta-carotene, zeaxanthin, lutein, vitamin A, vitamin E, selenium, and others have been advocated for the prevention of AMD and treatment of early stages of AMD or atrophic (dry) AMD. The Age Related Eye Study showed that zinc plus antioxidants reduced AMD progression risk by 26% in patients with intermediate AMD. The Beaver Dam Eye Study looked at the dietary and smoking history of individuals with AMD compared to matched normals and concluded that spinach and, to a lesser extent, other dark leafy green vegetables slightly reduce the progression to AMD (4). Smoking was found to have a doubling effect on the incidence of AMD (5). The authors predict that some specific antioxidants may be proven to have minor value if given to individuals at genetic risk for AMD (drusen). It is likely that this effect will be greatest in patients who do not eat dark leafy green vegetables, other vegetables, whole grains, and fruits. Beta carotene has been shown to increase the risk of lung cancer in smokers in a Swedish study (6). At this time, the authors counsel the children of patients with AMD, drusen patients, and patients with early AMD to stop smoking and eat spinach and other dark leafy green vegetables at least five times per week.
The Age Related Eye Disease Study (AREDS) 2 Study is underway, which is structured to assess the effects of oral supplementation of macular xanthophylls (lutein and zeaxanthin) and/or long-chain omega-3 fatty acids (docosahexaenoic acid and eicosapentaenoic acid) on the progression to advanced AMD. An additional goal of the study is to assess whether forms of the AREDS nutritional supplement with reduced zinc and/or no beta-carotene work as well as the original supplement in reducing the risk of progression to advanced AMD.
Plasmapheresis
A randomized trial of plasmapheresis did not reach the primary endpoint, and there is no scientific rationale for this treatment. Unfortunately, many patients have been treated using this unproven, expensive treatment.
Laser Treatment of Drusen
Laser treatment of drusen has been shown to cause faster disappearance of drusen than the spontaneous disappearance rate (7,8–9). The reader is reminded that drusen are “associated with” AMD; they do not “cause” AMD. Threshold treatment has been shown to result in higher CNV rates in two US studies now investigating subthreshold treatment (10,11). Two large randomized clinical trails failed to show a benefit of subthreshold laser treatment (Friberg, Fine CAPT).
Photodynamic Therapy
PDT was arguably the first therapy other than focal laser therapy available for the treatment of choroidal neovascularizations (12–20). Since the introduction of anti-VEGF therapy, the use of PDT has decreased dramatically. There are still physicians who recommend the use of PDT for certain patients, often in combination with anti-VEGF therapy. The use of PDT as monotherapy has largely been abandoned for choroidal neovascularizations.
The authors currently do not recommend PDT therapy in the management of CNVs, since they aim to avoid any further injury to the choriocapillaris, which is often damaged with PDT therapy.
ANTI-VEGF THERAPY STRATEGIES
The injection frequency selected for the pivotal Lucentis trials was based on preclinical pharmacokinetic data and assumptions about the length of time untreated CNV membranes remain active. This strategy resulted in patients having an average of 21 injections over 24 months. Rosenfeld introduced the concept of treat and observe based on time domain optical coherence tomography (OCT) evidence of subretinal fluid (SRF), Pigment epithelial detachment (PED), or retinal edema (Prospective OCT Imaging of Patients with Neovascular AMD Treated with Intraocular Ranibizumab (PrONTO) Study). More recently, Spaide et al. introduced the concept of treat and extend; generally, the notion is to give a “booster shot” after the CNV is not leaking based primarily on OCT evidence and a 6-week rather than a 1-month appointment. If the lesion is not leaking at the 6-week appointment, another injection is given and the patient is scheduled to return in 8 weeks. If the lesion remains inactive at this point, no injection is given and follow-up is done at 1- to 2-month intervals. More recently, many physicians, including the authors, use clinical decision making and include vision, visual complaints, exudates, and hemorrhage in addition to spectral domain OCT (Spectralis) to determine the need for retreatment. Fluorescein angiography (confocal using Spectralis), formerly the primary tool in determining the need for treatment, is still used anytime there is a visual complaint or decreased vision and SD OCT fails to reveal the etiology. Indocyanine green angiography is used less often but remains a valuable tool.
COMBINATION THERAPY
Combination therapy is an appealing concept but is appropriate in some instances and not in others. Combination chemotherapy in oncology is utilized because the agents have narrow windows between effective and toxic drug levels as well as to provide multiple barriers to the evolution of cancer cells. Combination therapy for infectious disease is utilized in severe infections when the infectious agent has not been identified and delayed treatment would produce bad outcomes. Combination therapy for infectious disease creates multiple barriers for the evolution of the infectious agent but unfortunately leads to higher incidence of resistance.
Focal and/or panretinal photocoagulation laser plus anti-VEGF therapy is very effective in diabetic retinopathy and can be broadly defined as combination therapy. Topical nonsteroidal (Nevanac) therapy in combination with laser and anti-VEGF compounds is effective for diabetic macular edema because of multiple mechanisms: VEGF and inflammation. Combination therapy has been advocated for CNV, but in the authors’ view, it has very little place with current therapeutic options in view of the extraordinary success of Lucentis and Avastin monotherapy. PDT requires intravitreal steroids, which results in an approximately 30% incidence of steroid glaucoma and approximately 90% incidence of cataract while only modestly reducing the need for anti-VEGF therapy.
Induction with Lucentis or Avastin followed by maintenance with pegaptanib sodium (Macugen) is based on the notion that pan-isoform suppression (Lucentis, Avastin) may result in neuronal loss but suppressing only the so-called pathological 165 isoform would not. Long-term pan-isoform suppression has not been demonstrated to have risk in patients.
Vitrectomy to enable epiretinal radiation with a Strontium 90 probe (NeoVista) is currently being studied but requires anti-VEGF therapy to be effective, produces cataract in 90% of the AMD patients because of the vitrectomy, reduces the effectiveness of the anti-VEGF agent by eliminating the inherent sustained-release action of vitreous, creates retinal detachment risk, and potentially will produce radiation retinopathy.
Vitrectomy Surgery for Submacular Hemorrhage Displacement
Submacular hemorrhages have been surgically removed since the early 1990s. These cases have widely varying outcomes based on the underlying pathology and surgical trauma involved (21–23). Some investigators have injected tissue plasminogen activator (tPA) under the retina at the time of surgery and wait approximately 45 minutes for apparent liquefaction of the clot before aspiration (24–27). Others inject tPA into the vitreous approximately 24 hours before surgical removal in an attempt to liquefy the blood clot (28). It has been reported that tPA can be injected into the vitreous cavity in the office followed by a gas injection and face-down positioning (29). Hilel Lewis has shown that tPA does not cross the primate retina (30). This less invasive method was thought to displace the blood away from the macula; however, there is no randomized clinical trial evidence of efficacy. The authors no longer recommend intravitreal tPA.
The authors have positive experience with a technique developed by Hilel Lewis to displace submacular hemorrhage from underneath the fovea, with the aim of decreasing foveal photoreceptor damage caused directly from the submacular hemorrhage. Thin submacular hemorrhages (those that do not have mass effect and do not cause foveal elevation) do not require surgery and can be managed with anti-VEGF monotherapy, as the hemorrhage will invariably clear spontaneously and the final visual outcomes are not worsened by the presence of thin submacular hemorrhage.
Massive submacular hemorrhages (those that cause macular elevation from submacular mass effect), on the other hand, do cause foveal damage and are amenable to hemorrhage displacement. The authors recommend performing spectral domain OCT on all of these patients, since it is often difficult to determine the presence of an underlying retinal pigment epithelium (RPE) detachment or sub-RPE hemorrhage that could give the clinical impression of massive macular elevation. If the OCT demonstrates a subfoveal RPE detachment or sub-RPE hemorrhage with a thin overlying subretinal hemorrhage, the authors do not recommend surgical displacement and treat with anti-VEGF monotherapy as described previously. Only those patients in whom the OCT demonstrates thick subfoveal hemorrhage should be treated with surgical displacement.
Another important clinical consideration for surgical displacement is the duration between first symptoms and surgery. The natural history of massive submacular hemorrhages is first to appear with dark red submacular blood that changes in color over the course of weeks to a slate gray appearance as the blood is dehemoglobinized. By the time the hemorrhage appears gray, the clot is frequently too dense and hard to be amenable for displacement, and often there is already photoreceptor damage. Given these considerations, it is important to proceed with surgical displacement within 2 weeks of initial symptoms, while the hemorrhage is still dark red in clinical appearance.
The surgical sequence is as follows: A core vitrectomy is performed with 25-gauge sutureless technique, a posterior vitreous separation is carefully created if not present, and tPA is injected into the clot using a 38-gauge polyamide cannula (developed for macular translocation surgery). The tPA can be injected using the viscous fluid injection system of the Accurus and Constellation vitrectomy machines to allow the surgeon to use foot control of injection pressure. The VFC system should be set to a maximum of 12 mm Hg to prevent overpressuring the subretinal space. A slow, controlled injection is then achieved and a pocket of subretinal tPA resembling an exudative retinal detachment is created underneath the macula and further expanded below the inferior vascular arcade to create a path for the hemorrhage to be easily displaced.
Total fluid-air exchange is performed, and the patient is kept in the supine position for 45 minutes after surgery. The patient is then placed in the seated position so that the air bubble will displace the liquefied blood clot inferiorly. Vertical position should be maintained for 48 to 72 hours to keep the displaced hemorrhage away from the fovea while the injected SRF is reabsorbed. Timing is important; blood clots over 2 weeks old should not be operated. Surgical aspiration of subretinal blood results in damage to the photoreceptor outer segments and Retinal Pigment Epithelium (RPE) apical processes and should be avoided. Intravitreal Avastin should be used to treat underlying CNV membranes after successful displacement of the blood.
Surgical success should be defined as only thin subfoveal hemorrhage on postoperative day one.
SUBMACULAR SURGERY AND MACULAR TRANSLOCATION
In 1991, Thomas and Kaplan reported submacular surgery for subretinal neovascular membranes secondary to presumed ocular histoplasmosis syndrome (31). Subsequently, these authors and many other surgeons applied their technique to idiopathic submacular membranes as well as membranes secondary to AMD and many other disease processes. In 1993, the principal author developed a simplified approach to submacular surgery, which will be described herein along with a discussion of indications in the context of the negative results of the Submacular Surgery Trial and the advent of effective anti-VEGF therapy.
Although the initial article referred to removal of “neovascular” membranes, it is actually a fibrovascular scar that is removed. It is common for the scar (membrane) to be significantly larger than the “net” seen on the angiogram presumably because of vascular regression in some areas (Fig. 21.1).
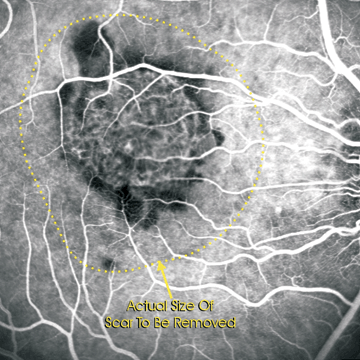
Figure 21.1 It is common for the fibrovascular scar (neovascular membrane) to be significantly larger than the “net” seen on angiography.
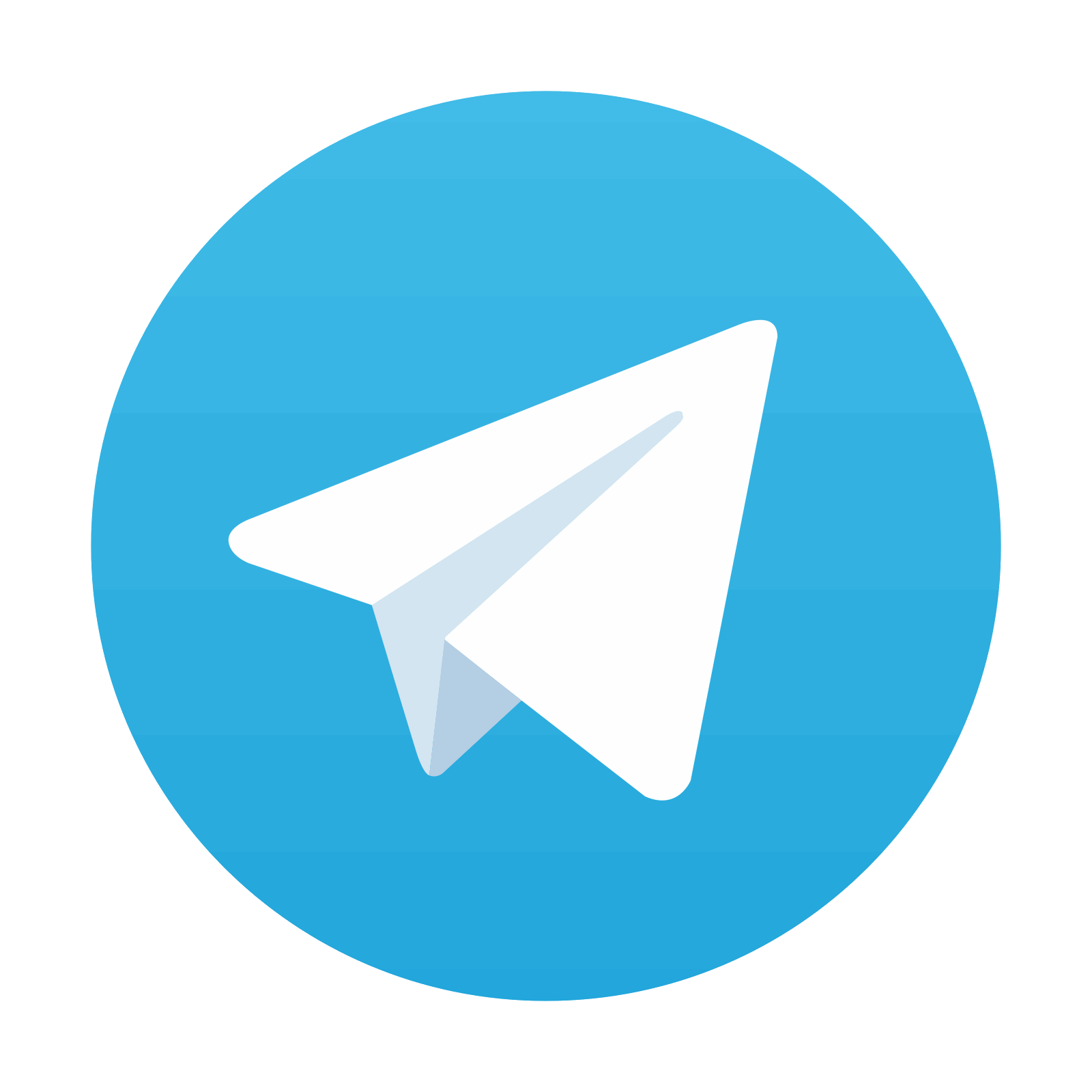
Stay updated, free articles. Join our Telegram channel

Full access? Get Clinical Tree
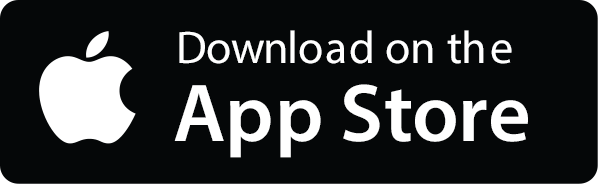
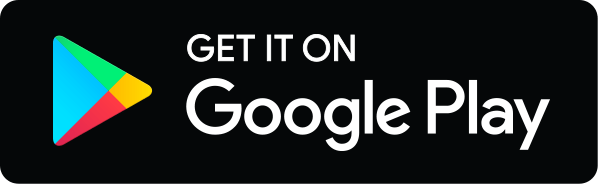