MAJ Jason N. Harris
practices neuro-ophthalmology and general neurology at the San Antonio Military Medical Center, TX, USA. He holds adjunct faculty appointments as an Assistant Professor of Neurology at the Uniformed Services University of the Health Sciences (USUHS), Bethesda, MD as well as UT Health Science Center, San Antonio, TX. He received a B.A. in Zoology from Idaho State University and subsequently attended the Uniformed Services University of the Health Sciences, Bethesda, MD where he graduated AOA in 2006. In 2010, he completed a neurology residency at Walter Reed Army Medical Center. He subsequently practiced general neurology at Tripler Army Medical Center, HI for three years and served in Afghanistan caring for patients with traumatic brain injuries. Dr. Harris completed a clinical fellowship in neuro-ophthalmology from The Wilmer Eye Institute at Johns Hopkins, Baltimore, MD in 2014.

Neil R. Miller
Dr. Neil Miller is currently Professor of Ophthalmology, Neurology, and Neurosurgery at the Johns Hopkins Medical Institutions and also the Frank B. Walsh Professor of Neuro-Ophthalmology. Dr. Miller was President of the North American Neuro-Ophthalmology Society from 2000 to 2002 and Chairman of its Executive Board from 2002 to 2004. He has been past President of the International Neuro-Ophthalmology Society on three occasions: in 1982, 1992, and 2008. He is also an emeritus member of the International Orbital Society. In 2009, he was given the Lifetime Achievement Award by the American Academy of Ophthalmology (AAO). He has authored or co-authored 500 articles, 90 chapters, and 13 books in the fields of neuro-ophthalmology and orbital disease. Many of Dr. Miller’s previous fellows and residents hold faculty positions at major institutions throughout the United States and around the world.
Overview
Traumatic optic neuropathy (TON) is a paradox of superficial simplicity riddled with deep and hidden complexities. A host of different types of direct and indirect and primary and secondary injuries can affect different portions of the optic nerve(s). Thus, in the setting of penetrating as well as non-penetrating head or facial trauma, a high index of suspicion should be maintained for the possibility that TON is present.
TON is a clinical diagnosis, with imaging frequently adding clarification to the full nature/extent of the lesion(s) in question. Each pattern of injury carries its own unique prognosis and theoretical best treatment; however, the optimum management of patients with TON remains unclear. Indeed, further research is desperately needed to better understand TON. Observation, steroids, surgical measures, or a combination of these is the current cornerstones of management, but statistically significant evidence supporting any particular approach for TON is absent from the literature. To date, there has been one randomized placebo-controlled study investigating management of TON. It investigated high-dose corticosteroids vs. placebo in patients with traumatic optic neuropathy from non-penetrating trauma. No significant differences were seen in visual outcome among groups in this very small study. There have not been any randomized studies investigating surgical management. There exists the possibility that novel management strategies will emerge as more is understood about the converging pathways of various secondary and tertiary mechanisms of cell injury and death at play in TON.
Given our current deficiencies in knowledge regarding how to best manage TON, “primum non nocere” (first do no harm) is of utmost importance.
Anatomy and Physiology of the Optic Nerve
In discussing traumatic optic neuropathy, it will be beneficial to first briefly discuss the anatomy of the optic nerve.
The term “optic nerve” is a misnomer. In fact, the optic nerve is anatomically and physiologically a white-matter tract of the brain [1]. Embryologically, it forms from the optic stalk, an outpouching of the diencephalon. It is myelinated by oligodendrocytes rather than Schwann cells. It has a blood-brain barrier identical with other portions of the brain (a possible exception being the optic nerve head or optic disc). It is nourished by pial vessels invested in pia mater and, like the rest of the brain, is surrounded by arachnoid and dura mater. Astrocytes support its axons, and fibroblasts also are present. As might be expected, the optic nerve does not behave as do other nerves (including the other cranial nerves) when injured. Given that the optic nerve is, in fact, part of the central nervous system (CNS), treatment paradigms for TON are largely extrapolated from traumatic spinal cord and to some extent traumatic brain injury literature [2].
The optic nerve can be described as having four anatomical sections: the intraocular, orbital, canalicular, and intracranial portions. It also can be divided more broadly clinically into anterior and posterior segments (Fig. 17.1) [3].


Fig. 17.1
The optic nerve. Numbers refer to segments of the nerve. 1 intraocular segment, 2 orbital segment, 3 canalicular segment, 4 intracranial segment, 5 optic chiasm
The Intraocular Optic Nerve
The intraocular optic nerve is the shortest portion of the nerve. It can be divided into prelaminar and laminar segments. It is composed of about 1.2 million retinal ganglion cell (RGC) axons along with astrocytes, fibroblasts, and capillary-associated cells [4]. The prelaminar segment, also known as the optic nerve head or optic disc, is typically unmyelinated. At the optic disc, the RGC axons make an approximately 90° turn. They then pass through the 200–300 openings in the lamina cribrosa as the laminar segment of the nerve. The central retinal arteries and veins also travel through this portion of the nerve. The most superficial portions of the prelaminar portion of the nerve are supplied by small branches of the central retinal artery, whereas the remainder of the intraocular optic nerve is supplied mostly from the choroid, which in turn is supplied by short posterior ciliary arteries. The laminar portion usually is supplied more directly by several autoregulated posterior ciliary arteries (Fig. 17.2) [5–7].


Fig. 17.2
Blood supply of the intraocular optic nerve
The Orbital Optic Nerve
The orbital portion of the optic nerve is approximately 30 mm in length (Fig. 17.3).


Fig. 17.3
Anatomy of the optic nerve. The orbital portion of the nerve is approximately 30 mm in length. Note that the nerve is surrounded by orbital fat and covered by a dural sheath that extends through the optic canal
Collagenous tissue divides it into septae. It is surrounded by fat, the short and long posterior ciliary arteries, the short and long posterior ciliary nerves, and the rectus muscles. The distance from the posterior portion of the globe to the orbital apex is about 18 mm. This difference between the length of the nerve in the orbit and the distance from the posterior aspect of the globe to the orbital apex allows necessary eye movements and also provides a protective mechanism against optic nerve damage from proptosis unless it is extreme. The RGC axons usually become myelinated by oligodendrocytes after exiting the lamina cribrosa, resulting in enlargement of the nerve diameter from 3 to 4.5 mm. The nerve is encased by the optic sheath. The outer layer of this sheath, the dura mater, is 0.3–0.5 mm thick. The subarachnoid space of the nerve is contiguous with the subarachnoid space of the brain. Pial layers, mostly supplied by posterior ciliary arteries, surround the nerve, carrying in them capillaries subserving the nerve. As is the case elsewhere in the brain, there is a blood-brain barrier. Approximately 10 mm posterior to the globe, the central retinal artery, a major branch of the ophthalmic artery, pierces the dura inferomedially to the nerve and travels within the nerve to exit at the optic disc where it divides into superior temporal, inferior temporal, superior nasal, and inferior nasal branches. The portion of the nerve anterior to where the central retinal artery pierces the dura is sometimes referred to as the “anterior” portion of the nerve, whereas the remainder of the nerve is referred to as the “posterior” optic nerve [10].
As the optic nerve continues to travel posteriorly, it traverses the annulus of Zinn prior to exiting the orbit. The annulus is a tendinous structure to which the four rectus muscles are attached. It overlies both the optic canal and a portion of the superior orbital fissure laterally. In this region, the ophthalmic artery is inferolateral to the optic nerve. Lateral to the optic nerve in the annulus as they traverse the superior orbital fissure are the superior division of the oculomotor nerve, the nasociliary nerve (a branch of V1), the abducens nerve, the inferior division of the oculomotor nerve, and the superior ophthalmic vein (Fig. 17.4a, b). Also lateral to the optic nerve but anterior to the annulus is the ciliary ganglion [2].




Fig. 17.4
(a) Relationship of the orbital portion of the optic nerve to other orbital structures. (b) Relationship of the orbital portion of the optic nerve to other orbital structures
The blood supply of the orbital segment of the optic nerve is variable (Fig. 17.5). Centripetally penetrating pial vessels line the optic nerve throughout its extent; however, anteriorly, these vessels receive their blood supply primarily from posterior ciliary arteries, whereas posteriorly, their origin not only is from the posterior ciliary arteries but also directly from the ophthalmic artery. In addition, portions of the anterior nerve receive some blood supply from the central retinal artery and, in some cases, from the external carotid artery via branches from the middle meningeal, superficial temporal, and transverse facial arteries [2, 11]. Indeed, postmortem studies suggest a rich anastomotic network of blood vessels in this region, and this is perhaps one reason that this portion of the nerve seems more resistant to injury than other segments. However, there are in vivo animal studies that call into question the degree of anastomoses [12].


Fig. 17.5
Blood supply of the optic nerve
The Canalicular Optic Nerve
Immediately after the optic nerve traverses the annulus of Zinn, it enters the optic foramen to traverse the optic canal (Fig. 17.3). This canal runs posteromedially at an angle of about 35° with the mid-sagittal plane (Figs. 17.3 and 17.6).


Fig. 17.6
Orientation of the optic canals
The dura mater is contiguous with both structures, and the dura surrounding the nerve is tightly adherent to the periosteum of the canal, particularly superiorly (Figs. 17.3 and 17.7a, b).


Fig. 17.7
(a) The canalicular portion of the optic nerve, horizontal section. (b) The canalicular portion of the optic nerve, schematic drawing (note the tight attachment of the dura to the nerve within the canal)
The canal, which lies between the two roots of the lesser sphenoid wings, is 8–10 mm long and is wider at its intracranial end than at its orbital end [13]. The lateral wall of the canal is the optic strut, an important landmark that separates the canal from the superior orbital fissure. The thinnest portion of the canal is the medial wall, averaging 0.21 mm [14]. On the other side of this wall is the sphenoid sinus and, occasionally, a posterior ethmoid sinus. In 4 % of patients, there is only membrane separating the canal and the sinus [15]. The roof of the optic canal is significantly thicker, 2 mm on average.
The Intracranial Optic Nerve
As the optic nerve exits the optic canal and enters the intracranial cavity, the dura that surrounds it peels away to line the skull base. Superiorly, however, it forms a sharp fold – the falciform fold – that overhangs the canal (Fig. 17.8).


Fig. 17.8
Anatomy of the intracranial portion of the optic nerves. Note the falciform ligament (or fold). (a) The ligament is intact. (b) The dura overlying the right optic canal has been removed, revealing the underlying bone. The fold overlying the intracranial portion of the optic nerve at its exit from the canal is apparent. Car. A. internal carotid artery, Rec. A. recurrent artery of Heubner, Lent. Str. A. lenticular striate arteries, Ant. Per. Subst. anterior perforated substances, A1, A1 segment of the anterior cerebral artery, A. Ch. A. anterior choroidal artery, P. Comm. Art. posterior communicating artery, Ant. Clin. anterior clinoid process, Falc. Lig. falciform ligament (fold), M1, M1 segment of the middle cerebral artery, CN 1 olfactory nerve, CN II optic nerve
Thus, the intracranial portion of the nerve is surrounded only by pia mater and arachnoid as it travels posteriorly, superiorly, and medially to merge with the opposite optic nerve to form the optic chiasm. The length of the intracranial optic nerve is highly variable, ranging from 4 to 15 mm [11].
The internal carotid artery is lateral to the optic nerve as is the anterior clinoid process (Fig. 17.8). The blood supply to the intracranial optic nerve comes from the internal carotid artery, the superior hypophyseal arteries, the A1 segment of the anterior cerebral artery and the anterior communicating artery (Fig. 17.5) [17].
Pathogenesis of Traumatic Optic Neuropathy
The mechanism of injury is one important way of classifying TON, with most authors using the terms “primary” and “secondary” to describe the mechanisms by which the optic nerve can be damaged [10, 18].
Primary Mechanisms of Optic Nerve Damage
Primary mechanisms usually are described as either “direct” (penetrating) or “indirect” (blunt trauma). According to Steinsapir and Goldberg, “Direct injuries are open injuries where an external object penetrates the tissues to impact the optic nerve. Indirect optic nerve injuries occur when the force of collision is imparted into the skull and this energy is absorbed by the optic nerve” [10].
Direct injuries are less common than indirect injuries because of the protection offered by the orbit [2] and because a much larger surface area is available to receive the forces necessary for an indirect injury. Direct optic nerve injuries cause instantaneous and often irreversible damage to the portion of the nerve involved. Presumably, this is due to transection of the RGC axons. Walsh believed that immediate ischemia associated with contusion necrosis caused by direct damage to the microcirculation also plays a role [10]. Even with treatment, direct injury to the optic nerve carries a poor prognosis. This is particularly true when the injured eye has no light perception immediately after the injury [19, 20].
Indirect damage to the optic nerve occurs from a wide range of mechanisms [21–24]. In his descriptions of these mechanisms, Walsh included transient “concussion” of the nerve, more permanent “concussive” injuries, lacerations of some nerve fibers (but not the entire nerve), and immediate “contusion necrosis” [23]. Indeed, there are various manners in which forces can be transmitted to the optic nerve in indirect injury, ranging from transmission of concussive forces directly to the tightly adherent nerve in the optic canal to transmission of forces from attached structures farther away, such as may happen when rotational or translational forces are applied to the globe or brain (i.e., from a finger or a fall) [22, 23, 25 – 29]. In particular, when the forehead or temporal region is struck, forces travel posteriorly through the optic canal, potentially injuring the optic nerve within the canal [30]. This may explain why it is much more common for frontal blunt trauma than occipital trauma to cause indirect TON [22, 31, 32]. In addition to forces applied to the skull or facial bones, forces applied directly to the globe (both high- and low-velocity mechanisms) can cause optic nerve damage [29, 33–35], although such damage usually is masked by severe trauma to the globe itself. In some cases, the blunt trauma is caused not by a direct blow to the skull but by a distant effect such as a blast wave [36].
The distinction between direct and indirect mechanisms of injury can at times be blurred. For example, a fracture of the optic canal from an indirect injury that results in a transection of the optic nerve is considered an indirect injury by most authors, even though there may be little difference in outcome between this and a foreign body penetrating and lacerating the nerve [37], as either type of primary injury can cause tears in the dura or nerve sheath, hemorrhage into the nerve or sheath spaces, and compression necrosis (Fig. 17.9).


Fig. 17.9
Direct injury to the optic nerve. Left, longitudinal section through the nerve shows full thickness laceration. Right, in another case, direct injury resulted in an extensive subdural hemorrhage surrounding the nerve
Unfortunately, by the time a patient with a direct or indirect injury to the optic nerve is evaluated by a physician, there usually is nothing that can be done to reverse the damage. All currently available therapies thus are aimed at preventing further – i.e., secondary – injury to the nerve, something about which we know even less than we do about primary mechanisms of injury.
Secondary Mechanisms of Optic Nerve Damage
In addition to primary direct or indirect optic nerve damage, further injury/exacerbation of injury can be caused by secondary mechanisms that occur after the moment of impact. Usually, visual loss is immediate; however, delayed visual loss, presumably from secondary mechanisms, occurs in at least 10 % of cases [8]. Hippocrates described this phenomenon over 2000 years ago, writing “Dimness of vision occurs in injuries to the brow and in those placed slightly above. It is less noticeable the more recent the wound, but as the scar becomes old, so the dimness increases” [39]. Proposed mechanisms of secondary injury include vasospasm, edema, hemorrhage, and local compression of vessels or systemic circulatory insufficiency/failure leading to necrosis of the nerve. There also is evidence of numerous cell death mechanisms at play, including but not limited to the generation of oxygen-free radicals, arachidonic acid release, triggering of bradykinin pathways, disturbances of intracellular calcium cascades, production of excitatory amino acids, and interference with TRP, NMDA, and AQP channels [10, 40–43]. An extensive discussion of these secondary mechanisms is beyond the scope of this chapter.
Perhaps the most important common element to remember with respect to the various secondary mechanisms of indirect injury to the optic nerve is the role that ischemia is believed to play in each of them, either as a causative or resultant factor. Given this role, optimum secondary injury prevention strategies generally are designed to decrease ischemia or the inflammatory and apoptotic cascades that lead to or are associated with it [44].
Location of Injury
In addition to the mechanisms of injury, a description of TON should include the portion of the optic nerve that is injured [2, 3, 37].
Clinically, the injury can happen at either the anterior or posterior optic nerve (or both). As mentioned above, the anterior aspect of the nerve is that portion of the nerve anterior to where the central retinal artery enters and the central retinal vein exits the nerve, about 8–10 mm posterior to the globe [3, 21, 37, 44]. Both the anterior and posterior nerve can be injured by direct and indirect mechanisms as well as by one or more of the secondary mechanisms described above [10].
Anterior Traumatic Optic Neuropathy
Anterior TON, whether of the direct or indirect variety, is characterized by optic disc swelling in the setting of other evidence of an optic neuropathy (e.g., decreased acuity, poor or absent color vision, a visual field defect, and a relative afferent pupillary defect if the injury is unilateral or asymmetric). It usually is accompanied by injury to associated vasculature. This, in turn, can lead to retinal ischemia or infarction, central retinal vein occlusion, anterior ischemic optic neuropathy, or a combination of these phenomena (Fig. 17.10) [45, 46].


Fig. 17.10
Anterior indirect traumatic optic neuropathy in a young boy who was hit in the right eye while playing soccer
Choroidal rupture can also be an accompanying feature [47]. Because of the severity of optic nerve and, often, intraocular damage that usually is associated with direct injury to the anterior optic nerve, the prognosis for anterior TON usually is poor in this setting, regardless of treatment [3]. The prognosis for anterior TON caused by indirect injury, however, is not as clear. Indirect anterior TON usually results from blunt forces applied to the globe. Rotational and translational forces as well as sudden shifts in intraocular pressure have all been implicated in the pathogenesis of the damage. In such instances, the main force to the optic nerve appears to be at the junction of the nerve and sclera, on the side opposite the impact [29]. Tangential glancing blows can cause a rotary avulsion with minimal globe disruption. More often, off-center impact to the globe may cause intraocular damage and also be associated with slower “rotational-rebound” evulsion of the nerve [48]. There thus are a variety of mechanisms by which forces on the globe can lead to partial or complete optic nerve avulsion [27, 28, 33, 49–51]. In milder cases, there may not be avulsion, but there still may be injury to the nerve and its vasculature. In most of these cases, regardless of the nature of the forces, no treatment is likely to be of benefit in limiting optic nerve damage, let alone restoring vision (Fig. 17.11).


Fig. 17.11
Fatal indirect anterior traumatic optic neuropathy. Note subdural hematoma at the junction of the optic nerve and globe
The one exception to this rule is the presence of a hemorrhage in the subdural or subarachnoid space surrounding the anterior optic nerve. In such a setting, which can be suspected by the clinical findings and confirmed with computed tomographic (CT) scanning, magnetic resonance (MR) imaging, or ultrasonography, and urgent optic nerve sheath fenestration may be beneficial, particularly if it is clear that the patient had vision immediately after the injury and/or had a normal-appearing optic disc but then experienced progressive visual loss associated with the development of optic disc swelling [52].
Posterior Traumatic Optic Neuropathy
Posterior TON is much more common than anterior TON, with indirect trauma being much more common than direct trauma. As is the case with anterior TON, posterior TON caused by direct or penetrating trauma has a poor prognosis regardless of treatment; however, this is not necessarily the case with respect to posterior TON caused by indirect trauma.
In patients with posterior TON caused by indirect trauma, the canalicular portion of the optic nerve is most often the site of damage, with the next most common portion being the intracranial portion of the nerve as it passes beneath the falciform fold (Fig. 17.8) [21–23, 27, 28, 38, 45, 53, 54]. There are a number of reasons for this. First, as noted above, studies have shown that blunt trauma to the frontal region produces shock waves that travel posteriorly toward and often through the canal, at least in part because the conical shape of the orbit may funnel forces to this particular region (Fig. 17.12a, b) [30, 31].


Fig. 17.12
(a) Direction of concussive forces from blunt trauma to the forehead (note that the lines of force can traverse either the ipsilateral or contralateral optic canal). (b) Direction of concussive forces from blunt trauma to the forehead (note that the lines of force can traverse either the ipsilateral or contralateral optic canal)
This concussive force may or may not be associated with fractures of the optic canal that also can introduce a crushing or lacerating component to the initial trauma. In addition, the optic canal is the only location along the length of the optic nerve where the nerve is tightly tethered to a fixed structure – the bone of the canal (via the nerve sheath and periosteum) (Fig. 17.7a, b). Thus, not only are concussive forces more likely to be propagated via bone to this portion of the nerve than to any other portion, but this portion of the nerve has less ability to respond to even minor primary damage. Rotational or translational forces of the brain at the time of blunt head and/or facial trauma may play an important role with respect to injury to the portion of the optic nerve passing underneath the falciform fold [2, 10, 55, 56], whereas forces on the globe are less likely to play a significant factor to the tethered nerve given the generous laxity of the intraorbital nerve.
The above factors do not take into account the vulnerability of the canalicular portion of the optic nerve to subsequent secondary injury following the initial injury. The tight confines of the canal make this portion of the nerve particularly vulnerable to edema and hemorrhage, resulting in a compartment syndrome causing further ischemia and secondary damage (Fig. 17.13) [23].


Fig. 17.13
Subarachnoid, subdural, and intraneural hemorrhage of the optic nerve in a fatal case of posterior indirect traumatic optic neuropathy
Additionally, as previously noted, this region of the nerve is a watershed zone, unlike other portions of the nerve that receive blood via rich anastomoses. It is not clear how – or if – this watershed component plays a role in the prevalence and evolution of secondary injury in posterior indirect TON to this region of the nerve, but it certainly may be a factor.
Epidemiology of Traumatic Optic Neuropathy
A recent prospective surveillance in the UK found TON to affect 1 in 1,000,000 of the general population [57]. Series that have evaluated TON in the setting of both blunt and penetrating head and facial injuries have found that it is present in 0.7–2.5 % of cases [22, 58–60]. In most series, the vast majority of affected patients are young adult males [38, 57]. Motor vehicle accidents, bicycle accidents, falls, assaults, and sport injuries are common causes of injury [2, 38, 57]; however, other mechanisms of injury have been reported, including self-inflicted oedipism [51], diving accidents [33], and optic nerve massage [61]. Although seemingly trivial injuries can cause TON [44], the trauma usually is severe, with 34–75 % of cases in both prospective and retrospective series being associated with loss of consciousness and 36–82 % of affected eyes having no light perception [38, 57, 62]. The male prevalence and other presenting characteristics of TON are similar in pediatric and adult populations [63, 64].
Factors cited by various authors as portending a worse potential for recovery include age over 40, history of loss of consciousness or significant head injury, history of immediate visual loss, poor initial visual acuity, presence of blood in the posterior ethmoid air cells by imaging, presence of an orbital fracture or a fracture of the optic canal, and failure to recover after 48 h of steroid therapy (but see below) [20, 57, 65].
Clinical Evaluation
TON is initially a clinical diagnosis, with imaging frequently adding clarification to the full nature and extent of the injury. As noted above, as up to 2.5 % of patients with head injury have TON, a high index of suspicion should be maintained for this condition in that setting. Obviously, the first priority in patient care needs to be ensuring and maintaining the patient’s cardiorespiratory status and neurological stability. Given these requirements, initial assessments by the ophthalmologist may be limited or delayed. Indeed, it is not uncommon for head-injured patients, particularly those who have experienced loss of consciousness, to be diagnosed with TON weeks or even months after the injury when they are finally sufficiently alert and able to communicate with their physicians and families. Nevertheless, we believe that patients who have experienced head or facial trauma should be evaluated for the possibility of TON at the earliest opportunity.
History
Once a patient who has experienced facial or head trauma is deemed stable from a cardiorespiratory and neurological standpoint, an in-depth ocular history and examination should be performed. If the patient is unconscious or confused (as is often the case), history from witnesses should be sought. It is important to elicit from the history when the trauma happened, what the mechanism(s) were, what associated factors may have been present (any exposure to chemicals, etc.), and the nature and progression (or lack thereof) of visual deficits since the incident. This latter point is particularly important as it may indicate a secondary injury amenable to therapy.
Examination
The importance of the examination in the diagnosis of TON cannot be overemphasized, as patients often are unconscious or confused, with no witnesses available to provide an adequate history. General inspection of the eye and ocular adnexa is the first step. Any evidence of orbital or ocular penetrating injury should be noted, as should any periorbital swelling, ecchymosis, proptosis, and enophthalmos [10]. The orbital rim should be palpated gently for any fractures. When possible, a portable slit-lamp examination should be performed to determine if there is a hyphema or a lens dislocation, although the former often can be appreciated using a simple handlight.
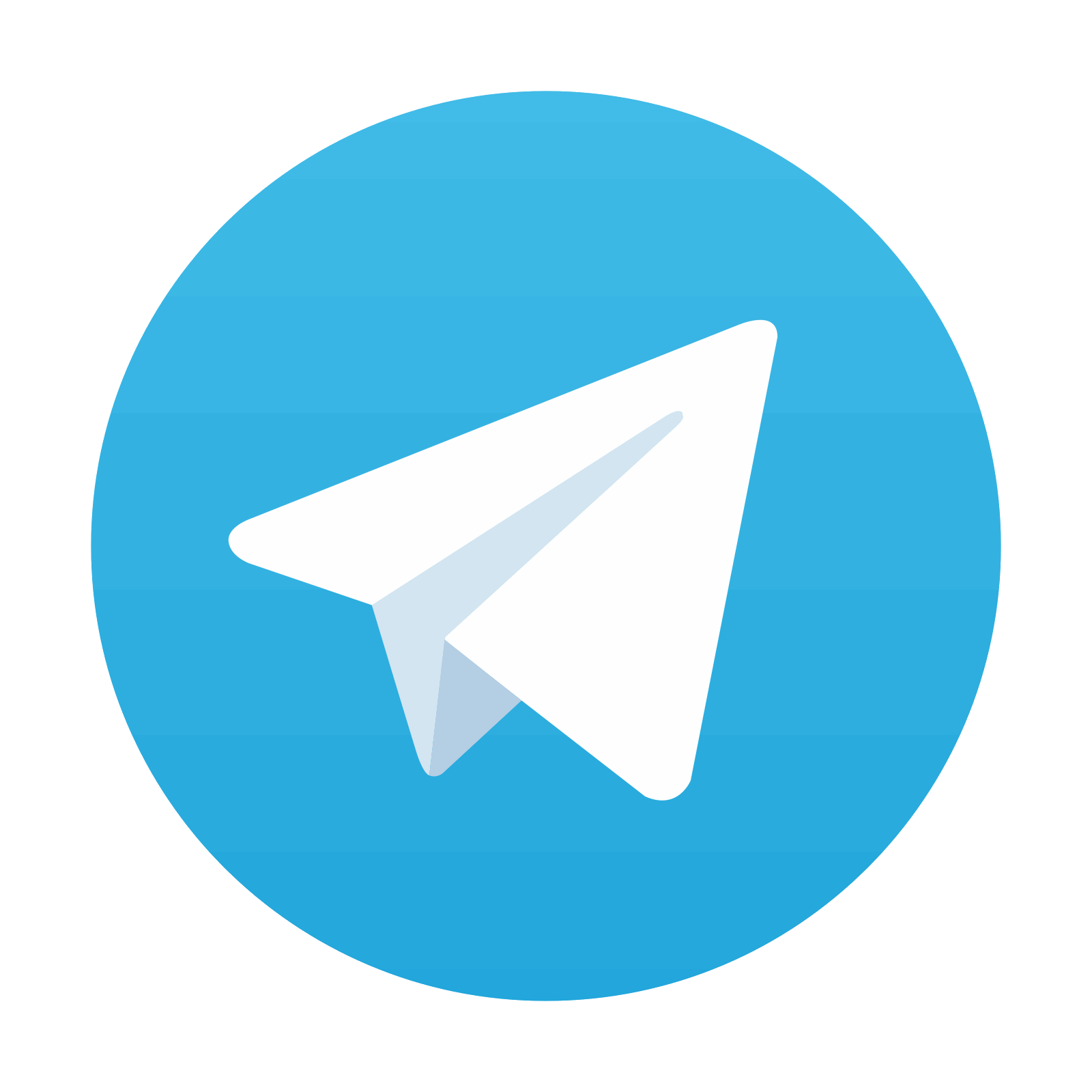
Stay updated, free articles. Join our Telegram channel

Full access? Get Clinical Tree
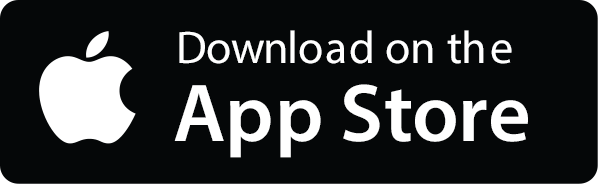
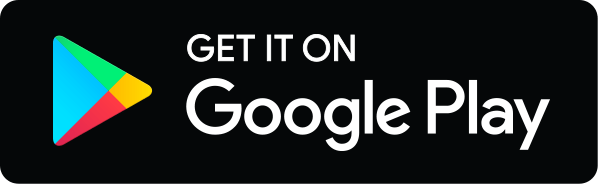