This article discusses the epidemiology, diagnosis, and management of traumatic cerebrospinal fluid (CSF) leaks. An overview of traumatic CSF leaks is presented, and both conservative and operative therapies are reviewed. Management decisions are discussed based on the current literature. Controversial clinical topics are addressed, including the use of prophylactic antibiotics and the timing of surgical repair.
- •
Eighty percent of cerebrospinal fluid (CSF) leaks occur following nonsurgical trauma and complicate 2% of all head traumas, and 12% to 30% of all basilar skull fractures
- •
The most common presentation is unilateral watery rhinorrhea, and β 2 -transferrin is now the preferred method of confirming a fluid as CSF
- •
High-resolution computed tomography (CT) is the preferred method of localizing the site of skull base defect following craniomaxillofacial trauma, but can be coupled with magnetic resonance imaging (MRI) or cisternography
- •
Conservative management of CSF leaks includes strict bed rest, elevation of the head, and avoidance of straining, retching, or nose blowing, and results in resolution of the majority of traumatic CSF leaks over a 7-day period
- •
CSF diversion improves the rates of resolution when added to conservative therapy
- •
Endoscopic endonasal approaches should be the preferred method of repair with greater than 90% success rates; however, open transcranial and extracranial repairs still have their place in operative management
- •
Small postsurgical defects can be repaired endoscopically with mucosal grafts, while the pedicled nasal septal flap has emerged as the preferred method of repair for large defects
- •
Prophylactic antibiotics have not been shown to reduce the risk of meningitis and may select for more virulent organisms
- •
Data suggest that early surgical repair (<7 days) can reduce the risk of meningitis.
EBM Question | Level of Evidence | Grade of Recommendation |
---|---|---|
Do spontaneous CSF leaks represent a distinct clinical entity and a variant of idiopathic intracranial hypertension? | 2b | B |
Does a reduction in CSF pressure alone lead to resolution of CSF leaks? | 5 | D |
Does decreasing ICP, transiently or long-term, improve outcomes of endoscopic closure of spontaneous CSF leaks? | 4 | C |
CSF is an ultrafiltrate of plasma or serum, which contains electrolytes, glucose, and proteins. Located in the cerebral ventricles and cranial/spinal subarachnoid space, this fluid serves in physical support and provides buoyancy for the brain and spinal elements. CSF also serves to maintain homeostasis of neural tissues by removing by-products of metabolism and regulating the chemical environment of the brain. CSF is produced by the choroid plexus in the lateral, third, and fourth ventricles. It circulates from the lateral ventricles through the foramina of Monro into the third ventricle and then to the fourth ventricle through the aqueduct of Sylvius, then communicates with the subarachnoid space through the foramen of Magendie and foramina of Luschka. CSF circulates throughout the meninges between the arachnoid and is reabsorbed into the venous system via arachnoid villi, which project into the dural venous sinuses. Adults average 140 mL of CSF volume, and the body produces 0.33 mL of CSF per minute and thus regenerates its CSF volume 3 times per day. As CSF is an ultrafiltrate of serum, serum abnormalities will be reflected in CSF (ie, hyperglycemia). Fluctuations in the cerebral blood flow related to the cardiac output result in generalized brain volume fluctuations, which gives rise to the vascular pulsations seen in CSF. In addition, major branches of the circle of Willis are located in the subarachnoid space and are likely contribute to this phenomenon.
CSF leaks are rare but are associated with morbidity such as general malaise and headache. More importantly, they can lead to potentially life-threatening complications such as meningitis. As such, they require thorough and timely evaluation and treatment. CSF leaks occur when the bony cranial vault and its underlying dura are breached. Such leaks may be broadly categorized as traumatic or nontraumatic in origin. Traumatic causes can be further subclassified into surgical and nonsurgical, with surgical causes divided into planned (as in failure of reconstruction of a planned dural resection) or unplanned (as a complication following an ethmoidectomy). Nontraumatic CSF leaks may be subclassified into high or normal pressure leaks, with the recognition that tumors can occupy either subclass by mass effect in the high-pressure group or by direct erosive effect on the skull base in the normal-pressure group. If no cause can be found the leak may be classified as idiopathic; however, with careful history taking, physical examination (including nasal endoscopy), and radiologic evaluation, true idiopathic leaks are rare.
Epidemiology of cerebrospinal fluid leaks
Approximately 80% of CSF leaks result from nonsurgical trauma, 16% from surgical procedures (although this number is rising), and the remaining 4% are nontraumatic. Of the traumatic leaks, more than 50% are evident within the first 2 days, 70% within the first week, and almost all present within the first 3 months. Delayed presentation may result from wound contraction or scar formation, necrosis of bony edges or soft tissue, slow resolution of edema, devascularization of tissues, posttreatment tumor retraction, or progressive increases in intracranial pressure (secondary to brain edema or other process). As with most maxillofacial trauma, traumatic CSF leaks occur most commonly in young males and complicate 2% of all head traumas, and 12% to 30% of all basilar skull fractures.
Anterior skull base leaks are more common than middle or posterior leaks, due to the firm adherence of the dura to the anterior basilar skull. The most common sites of CSF rhinorrhea following accidental trauma are the sphenoid sinus (30%), frontal sinus (30%), and ethmoid/cribriform (23%) ( Fig. 1 ). Temporal bone fractures with resultant CSF leak can present with CSF otorrhea or rhinorrhea via egress through the Eustachian tube with an intact tympanic membrane. Although a rare complication of functional endoscopic sinus surgery (FESS), the frequency in which this procedure is performed makes it a significant cause of CSF leaks ( Fig. 2 ). When looking at surgical trauma, the most common sites of CSF leak following FESS are ethmoid/cribiform (80%), followed the frontal sinus (8%) and sphenoid sinus (4%). After neurosurgical procedures the most common site of CSF leak is the sphenoid sinus (67%) because of the high number of pituitary tumors that are addressed via transsphenoidal approach.
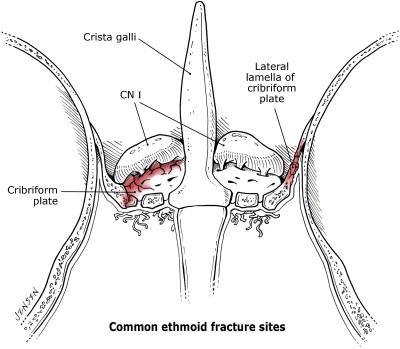
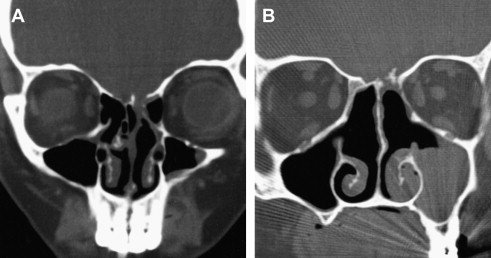
Regarding nontraumatic leaks, high-pressure leaks comprise approximately half of these, with more than 80% resulting from tumor obstruction. The remainder is caused by either benign intracranial hypertension (BIH) or hydrocephalus; however, recent publications suggest an increased role of BIH in idiopathic or “spontaneous” CSF leaks. One hypothesis is that in patients with occult elevated CSF pressure, an intermittent CSF leak may serve as a release valve that decompresses the elevated pressure. Once the leak resolves, either by the normalization of CSF pressure with an intermittent leak or by surgical repair, the CSF pressure will slowly increase and result in recurrence of the leak. It is not surprising that the association between idiopathic CSF leaks and BIH has been made, as the demographics of the populations are quite similar. Furthermore, the clinical manifestations and demographic profile of patients with empty sella syndrome are highly similar to those for patients with BIH and patients with nontraumatic CSF leaks. These associations have important clinical implications in the management of idiopathic CSF leaks as well as surgical failures after traumatic CSF leak repair.
Detection of cerebrospinal fluid leaks
Presentation
Presentation of traumatic CSF leaks can be subtle, and diligence is required when one is suspected. Fain and colleagues presented an analysis of 80 cases of trauma to the cranial base. From these cases they determined that there are 5 types of frontobasal trauma. Type I involves only the anterior wall of the frontal sinus. Type II involves the face (craniofacial disjunction of the Lefort II type or crush face), extending upward to the cranial base and to the anterior wall of the frontal sinus, because of the facial retrusion. Type III involves the frontal part of the skull and extends down to the cranial base. Type IV is a combination of types II and III, whereas type V involves only ethmoid or sphenoid bones. In this study CSF leaks were infrequent in types I and II, but occurred more frequently in types III, IV, and V, which included a dural tear in each case. Therefore, when a patient presents with one of these fractures a CSF leak should be suspected.
The most common presenting sign is unilateral watery rhinorrhea following skull base trauma. Patients may complain of a salty or even sweet taste in their mouth; however, with severe trauma to the skull, history may be unobtainable secondary to the patient’s neurologic status. Rhinorrhea is classically positional in nature and is most commonly associated with standing or leaning forward. Although this presentation seems straightforward, establishment of the diagnosis and precise localization of the leak can present major challenges. Other rhinologic pathology, including seasonal allergic rhinitis, perennial nonallergic rhinitis, and vasomotor rhinitis, are relatively common, and may mimic some of the signs and symptoms of CSF rhinorrhea or may occur simultaneously with a CSF leak. Furthermore, CSF rhinorrhea is often intermittent, even after trauma, which may lead to false-negative results on diagnostic testing if testing is performed during the quiescent phase. Lastly, the subarachnoid cistern is a relatively low-pressure system. Thus, leaks may be of low volume, which can lead to false-negative testing or failure to recognize that a leak even exists. In cases of high clinical suspicion and initially negative diagnostic testing, further follow-up with repeat testing is warranted.
Identification
Traditionally the presence of a halo sign (clear ring surrounding a central bloody spot) on gauze, tissue, or linen has been used to predict CSF leak following trauma. This halo forms as blood and CSF separate; however, this test should only be used to arouse suspicion as tears, saliva, and other non-CSF rhinorrhea can give false-positive results. Historically the components of the rhinorrhea (including glucose, protein, and electrolytes) have been measured to confirm the diagnosis of CSF. These tests, however, should not be relied on, as their sensitivity and specificity are unacceptably low.
β 2 -Transferrin has emerged as a highly sensitive and specific way of identifying CSF, and is now the preferred method of confirming a fluid as CSF. The method was initially discovered in 1979 by Meurman and colleagues who, when performing protein electrophoresis of CSF, tears, nasal secretions, and serum, noted a β 2 -transferrin fraction only in the CSF samples. Techniques for isolating this marker have been subsequently simplified and refined, leading to enhanced sensitivity and specificity. As with any test, a reliable result requires an adequate sample. Of note, although quite specific, there are reports of β 2 -transferrin being detected in aqueous humor and in the serum of patients with alcohol-related chronic liver disease.
Beta-trace protein (βTP) is another marker that has been used for the detection of CSF. This protein is produced by the meninges and choroid plexus and is released into CSF. It is present in other body fluids, including serum, but at much lower concentrations than in CSF. Detection of βTP has 100% sensitivity and specificity in cases of confirmed CSF rhinorrhea, but cannot be reliably used in patients with renal insufficiency or bacterial meningitis, because serum and CSF levels of βTP substantially increase with reduced glomerular filtration rate and decrease with bacterial meningitis.
Detection of cerebrospinal fluid leaks
Presentation
Presentation of traumatic CSF leaks can be subtle, and diligence is required when one is suspected. Fain and colleagues presented an analysis of 80 cases of trauma to the cranial base. From these cases they determined that there are 5 types of frontobasal trauma. Type I involves only the anterior wall of the frontal sinus. Type II involves the face (craniofacial disjunction of the Lefort II type or crush face), extending upward to the cranial base and to the anterior wall of the frontal sinus, because of the facial retrusion. Type III involves the frontal part of the skull and extends down to the cranial base. Type IV is a combination of types II and III, whereas type V involves only ethmoid or sphenoid bones. In this study CSF leaks were infrequent in types I and II, but occurred more frequently in types III, IV, and V, which included a dural tear in each case. Therefore, when a patient presents with one of these fractures a CSF leak should be suspected.
The most common presenting sign is unilateral watery rhinorrhea following skull base trauma. Patients may complain of a salty or even sweet taste in their mouth; however, with severe trauma to the skull, history may be unobtainable secondary to the patient’s neurologic status. Rhinorrhea is classically positional in nature and is most commonly associated with standing or leaning forward. Although this presentation seems straightforward, establishment of the diagnosis and precise localization of the leak can present major challenges. Other rhinologic pathology, including seasonal allergic rhinitis, perennial nonallergic rhinitis, and vasomotor rhinitis, are relatively common, and may mimic some of the signs and symptoms of CSF rhinorrhea or may occur simultaneously with a CSF leak. Furthermore, CSF rhinorrhea is often intermittent, even after trauma, which may lead to false-negative results on diagnostic testing if testing is performed during the quiescent phase. Lastly, the subarachnoid cistern is a relatively low-pressure system. Thus, leaks may be of low volume, which can lead to false-negative testing or failure to recognize that a leak even exists. In cases of high clinical suspicion and initially negative diagnostic testing, further follow-up with repeat testing is warranted.
Identification
Traditionally the presence of a halo sign (clear ring surrounding a central bloody spot) on gauze, tissue, or linen has been used to predict CSF leak following trauma. This halo forms as blood and CSF separate; however, this test should only be used to arouse suspicion as tears, saliva, and other non-CSF rhinorrhea can give false-positive results. Historically the components of the rhinorrhea (including glucose, protein, and electrolytes) have been measured to confirm the diagnosis of CSF. These tests, however, should not be relied on, as their sensitivity and specificity are unacceptably low.
β 2 -Transferrin has emerged as a highly sensitive and specific way of identifying CSF, and is now the preferred method of confirming a fluid as CSF. The method was initially discovered in 1979 by Meurman and colleagues who, when performing protein electrophoresis of CSF, tears, nasal secretions, and serum, noted a β 2 -transferrin fraction only in the CSF samples. Techniques for isolating this marker have been subsequently simplified and refined, leading to enhanced sensitivity and specificity. As with any test, a reliable result requires an adequate sample. Of note, although quite specific, there are reports of β 2 -transferrin being detected in aqueous humor and in the serum of patients with alcohol-related chronic liver disease.
Beta-trace protein (βTP) is another marker that has been used for the detection of CSF. This protein is produced by the meninges and choroid plexus and is released into CSF. It is present in other body fluids, including serum, but at much lower concentrations than in CSF. Detection of βTP has 100% sensitivity and specificity in cases of confirmed CSF rhinorrhea, but cannot be reliably used in patients with renal insufficiency or bacterial meningitis, because serum and CSF levels of βTP substantially increase with reduced glomerular filtration rate and decrease with bacterial meningitis.
Localization
Once confirmed, localization of the dural defect is critical to management of CSF leaks, particularly if operative management is considered. Following identification of a traumatic CSF leak, nasal endoscopy should be performed. This procedure may narrow the side/site of the leak. Findings commonly are nonspecific, including glistening of nasal mucosa, but occasionally active leaks can be identified. Although direct visualization plays an important role, imaging of the skull base is critical to localization of CSF leaks, particularly traumatic leaks.
High-Resolution CT Scan
Multiple imaging studies have been used to localize defects, but the most common is high-resolution CT (HRCT) scanning. This technology uses 1- to 2-mm sections in both the coronal and axial planes with bone algorithm (see Fig. 2 ), resulting in localization of the majority of skull base defects that result in CSF leak. However, it is important to recognize that congenital or acquired thinning or absence of portions of the bony skull base may be identified and may not necessarily correspond to the site of CSF leak. This technology can be used with most surgical image guidance systems. Due to the relative ease of obtaining this study and high degree of accuracy, this method should be used as the primary imaging modality for traumatic CSF leaks. Plain CT scans may lead to false-positive results secondary to volume averaging, and their use should be limited. The use of intrathecal fluorescein in combination with HRCT allows for the identification of most CSF leaks.
Intrathecal Fluorescein
Intrathecal agents have been used both to confirm the presence of and to attempt to localize CSF leaks. These agents are administered via lumbar puncture into the subarachnoid space and, as such, complications can be severe. Visible dyes, radiopaque dyes, and radioactive markers have been used with a positive result being visualization, either directly or radiographically, of the agent within the nose and paranasal sinuses.
Intrathecal fluorescein is the most popular visible agent. Popularized by Messerklinger, intrathecal fluorescein has been associated with multiple complications, including grand mal seizures and even death. However, in a study of 420 administrations low-dose (50 mg or less) intrathecal fluorescein was found to be useful in localizing CSF fistulas and was deemed unlikely to be associated with adverse events, as most complications were dose-related. The current recommended dilution is 0.1 mL of 10% intravenous fluorescein (not ophthalmic preparation) in 10 mL of the patient’s own CSF, which is infused slowly over 30 minutes. Patients should be extensively counseled about the risks, as this use is not approved by the US Food and Drug Administration (ie, off-label use).
CT Cisternograms
CT cisternography involves the intrathecal administration of radiopaque contrast (metrizamide, iohexol, or iopamidol) followed by CT scanning. Studies have shown that approximately 80% of CSF leaks can be confirmed through this technology. Weaknesses of this technology include its invasive nature, which can limit its use particularly in the pediatric population, as well as its low sensitivity in intermittent leaks. Positive findings usually reveal pooling of contrast in the frontal or sphenoid sinuses, but may not necessarily locate the actual defect. Furthermore, the density of the dye may obscure bony anatomy, leading to more difficulty in locating the bony defect.
Radionuclide Cisternograms
A variety of radioactive markers have been used to detect CSF leaks, including radioactive iodine ( 131 I)-labeled serum albumin, technetium ( 99m Tc)-labeled serum albumin or diethylenetriamine penta-acetic acid (DTPA), and radioactive indium ( 111 In)-labeled DTPA. This technique is similar to intrathecal fluorescein and involves administration of the tracer via a lumbar puncture. Intranasal pledgets are placed in defined locations under endoscopic guidance and analyzed for tracer uptake approximately 12 to 24 hours later. A scintillation camera is also used, but has poor resolution and difficulty precisely localizing the leak. Overpressure radionuclide cisternography increases the intrathecal pressure with a constant infusion to improve the sensitivity of radionuclide cisternography ; however, this modality in clinical practice results in a high degree of false-positive findings with sensitivities from 62% to 76%, limiting its utility.
MRI and MR Cisternograms
In contrast to the previously discussed cisternograms, MR cisternography is a noninvasive method for assessing the presence of intranasal CSF. This technique uses T2-weighted images with fat suppression and image reversal to highlight CSF. The characteristic signal tracking from the intracranial space to the paranasal sinuses represents a CSF leak. The sensitivity of this test is reported to be 85% to 92%, with 100% specificity. MRI and MR cisternography are able to distinguish inflammatory tissue from meningoencephaloceles; however, bony detail is poor ( Fig. 3 ).
