Surgery of oropharyngeal cancer has evolved from large, open transcervical and transmandibular approaches to minimally invasive transoral endoscopic techniques. Transoral laser microsurgery and transoral robotic surgery allow complete oncologic resection through the mouth with minimal cosmetic deformity and optimal speech and swallow function. With a significant increase in the incidence of oropharyngeal cancers, there is a growing role for up-front surgery, especially in young, healthy patients with human papillomavirus–associated squamous cell carcinoma. This article explores the development of transoral endoscopic surgery, its role in the multidisciplinary treatment of patients with oropharyngeal cancer, and oncologic and functional outcomes.
- •
Surgery of oropharyngeal cancers has evolved from extensive open approaches with significant associated morbidity to minimally invasive approaches through the mouth.
- •
Transoral laser microsurgery and transoral robotic surgery are 2 different techniques of transoral endoscopic surgery that can achieve complete oncologic tumor resection without cosmetic deformity while optimizing functional rehabilitation.
- •
An up-front surgical approach allows tailored adjuvant treatment based on a patient’s disease burden.
- •
Long-term side effects associated with chemotherapy and radiation may be reduced or avoided in carefully selected patients undergoing transoral surgical resection.
- •
Transoral endoscopic surgery will play an increasing role in the treatment of human papillomavirus–associated oropharyngeal cancer as incidence continues to increase.
AESOP | Automated Endoscopic System for Optimal Positioning |
NCCN | National Comprehensive Cancer Network |
OP | Oropharyngeal |
OPSCCa | Oropharyngeal squamous cell carcinoma |
PSS-HN | Head and Neck Performance Status Scale |
QOL | Quality of life |
TES | Transoral endoscopic surgery |
TLMS | Transoral laser microsurgery |
TORS | Transoral robotic surgery |
Historical perspectives
Surgery
Until the first quarter of the nineteenth century, there were few reports of operations of the head and neck for cancer, other than for the lip and oral tongue. Before the advent of anesthesia, the pain and inability to restrain even the most willing of patients precluded more than simple and rapid excisions of small tumors.
Although the discovery of anesthesia (ether, 1842; nitrous oxide, 1844; chloroform, 1847) rendered invasive procedures more tolerable, these inhalational gases and the cumbersome apparatuses necessary to administer them made manipulation in the mouth and throat and the control of bleeding even more difficult. Even if surgery were successful, there was no method of alimentation other than an oral diet and no antibiotic treatment to control infection. Postoperative care consisted of extended periods of bed rest (4–6 days after a general anesthetic, 48 hours after local anesthesia) because the benefits of early ambulation were not appreciated. High mortality resulted from exhaustion, general sepsis, and hemorrhage.
The limitations imposed by inhalational anesthetics made radiation the favored treatment method for tumors of the pharynx and larynx in the early 1900s. In the mouth, this was sometimes combined with cautery and endothermy excision either soon after the application of interstitial radon or later, when radionecrosis developed. Large wounds developed, were left open, and frequently resulted in bleeding requiring the ligation of one or both carotid arteries, which became one of the most common procedures performed. Postradiation edema with resultant airway obstruction was common in patients treated for pharynx and larynx cancer and led to many emergent tracheotomies.
In the late 1930s and 1940s, several important medical developments led to the advancement of head and neck surgery. The introduction of sulfa drugs and, later, penicillin, greatly reduced infection and the rates of postoperative wound dehiscence and fistula formation. The introduction of intravenous Pentothal anesthesia freed the surgeon from having to contend with large, cumbersome inhalational devices and led to the development of endotracheal tubes. Blood banks made transfusions possible in the event of life-threatening blood loss. The drum dermatome allowed for the harvest of large, split-thickness skin grafts of uniform thickness to cover extensive surface defects in the head and neck. These advances made radical head and neck surgery possible. Where previously the extent of extirpation was limited by such complications as shock, asphyxia, hemorrhage, infection, sepsis, and failure of wound healing, the primary limitation became the preservation of those structures necessary to maintain a patient’s life.
New surgical prowess led to the evolution of open surgical approaches to the oropharynx, including the lateral pharyngotomy, anterior pharyngotomy, mandibulotomy, and lingual release. These approaches start from the outside of the neck and move inward toward the mucosa of the upper aerodigestive tract, which can facilitate the identification and preservation of major neurovascular structures. They are frequently performed with an ipsilateral neck dissection and mandibulectomy (ie, Commando procedure). These procedures led to a great improvement in oncologic outcomes for patients with oropharyngeal cancer, but at a significant price. They result in large incisions with significant tissue dissection and distortion, disruption of the native musculature, and violation of the pharyngeal mucosa with resulting compromise in speech, swallow, and airway function. Patients often require temporary or permanent tracheotomies and feeding tubes and may require extensive rehabilitation. It was not until the end of the twentieth century, when technologic developments led to minimally invasive approaches to the upper aerodigestive tract combined with a human papillomavirus (HPV) epidemic, that a new era of oropharyngeal cancer surgery began.
Radiation and Chemotherapy
Although radiation therapy had an integral role in treatment of upper aerodigestive tract cancers in the early 1900s, it was eventually eclipsed as the first-line treatment modality by the advances in anesthesia and surgery mentioned earlier. However, the second half of the twentieth century saw great developments in radiation technology. In the 1970s, computers were introduced into treatment planning and, in combination with new imaging techniques of computed axial tomography, magnetic resonance imaging, and positron emission tomography scans, improvements in accurately targeting and dosing tumors of the head and neck became possible. More recent developments in intensity-modulated and image-guided radiotherapy led to further refinements while limiting toxicity to healthy tissue.
Concurrent advances in chemotherapy with the introduction of taxanes and biotargeted therapies such as cetuximab intensified the effects of radiation. Oncologic outcomes for patients treated nonoperatively eventually rivaled those treated by up-front surgery. However, the side effect profile and complications for traditional, open surgery remained high, whereas that of radiation and chemotherapy became more tolerable. This difference is shown in a meta-analysis by Parsons and colleagues. In a compiled report of 51 retrospective studies including 6400 patients with tonsillar cancer who underwent surgery (with or without radiation) and radiation (with or without neck dissection) at North American academic medical centers, the rates of local control, locoregional control, 5-year survival, and 5-year cause-specific survival were similar between the 2 groups. However, the rates of severe or fatal complications were significantly greater for the up-front surgery group (23%) compared with the radiation groups (6%). Furthermore, the available data on the functional consequences of treatment suggested the superiority of a nonsurgical approach. These data, combined with emerging studies showing the equivalence of combined-modality organ-preservation chemoradiation compared with total laryngectomy for advanced laryngeal cancer, led to a gradual shift in treatment paradigms for oropharyngeal cancer from operative to nonoperative treatment.
Because of the inherent morbidity of surgery and equivalent oncologic outcomes, nonsurgical treatment gained momentum at the end of the twentieth century. Nonetheless, to date, there is insufficient evidence showing a superior treatment response obtained from surgery or radiation with or without chemotherapy. The only randomized, prospective study comparing surgery versus radiation in the management of advanced head and neck cancer is the Radiation Therapy Oncology Group (RTOG) 73-03 trial. A subgroup of 70 patients with oropharyngeal cancer was randomized to receive either preoperative radiation, postoperative radiation, or radiation alone. There was no statistically significant difference in overall survival between these groups, although the study has been criticized for being underpowered. In contrast, a retrospective analysis of the National Cancer database has indicated that up-front surgical treatment of oropharyngeal cancer followed by radiation treatment does afford a survival benefit compared with radiation and chemoradiation alone.
In Hayes Martin’s landmark book Surgery of Head and Neck Tumors he recalls lamenting in the early 1920s the common belief that radiation therapy would entirely replace surgery in the treatment of cancer. As a resident, he regretted being born too late to ever be able to perform a total laryngectomy. This sentiment resonates with many young otolaryngologists who have completed training in the last 20 years. With the advent of modern chemoradiation, surgery of the oropharynx has been relegated to a salvage undertaking in many institutions. Just as Dr Martin went on to learn the integral role of surgery in laryngeal cancer, we too are realizing the increasing role of primary surgery in the treatment of oropharyngeal cancer with the advancements of minimally invasive surgical approaches.
Current minimally invasive surgical approaches
Minimally invasive surgery is designed to reduce morbidity and mortality without compromising oncologic outcomes. The ideal minimally invasive approach to the oropharynx is via a transoral approach, thereby limiting tissue dissection, disruption of speech and swallow musculature, minimizing blood loss, avoiding major neurovascular structures, and limiting injury to normal tissue. Transoral approaches to the oropharynx have traditionally been limited to those tumors that can be directly visualized and manipulated with standard instrumentation and lighting. Because of line-of-sight limitations for tumors in the base of the tongue, transoral approaches had been reserved for smaller lesions of the tonsil and palate. However, in the last 20 years, innovation in surg tech has greatly expanded the ability to resect tumors of the oropharynx via a transoral approach ( Table 1 ). Transoral laser microsurgery (TLMS) and transoral robotic surgery (TORS) are the principal minimally invasive transoral endoscopic approaches used for the oropharynx to currently.
Inclusion | Exclusion |
---|---|
T1/T2 oropharynx lesions | Involvement of carotid artery, prevertebral fascia, mandible |
NO, N1, N2a, N2b neck disease | Distant metastasis |
Patients who will definitively need chemoradiation despite surgical intervention (obvious extracapsular nodal extension, low likelihood of obtaining negative margins at the primary site) | |
Extensive unilateral or bilateral soft palate involvement | |
Extension of disease into the nasopharynx | |
Tonsillar lesions with extension along the posterior pharyngeal wall past the midline | |
Deep base of tongue lesions with extension to the contralateral side | |
T4 lesions | |
Trismus or other anatomic factors precluding transoral access to the oropharynx | |
Possible Operative Candidates | — |
T3 disease | — |
Cystic N3 disease | — |
HPV-negative oropharynx tumors may best be treated by surgery followed by chemotherapy and radiation, given their poor prognosis | — |
TLMS
Technologic advancements in both binocular microscopy and surgical lasers made precise tissue cutting with minimal blood loss possible. In addition, the recognition that tumors in the upper aerodigestive tract need not be removed in continuity with cervical lymph nodes, and that they may be removed piecemeal without resultant tumor dissemination, led to the development of TLMS in the 1960s and 1970s. Since its inception in Europe, where TLMS showed excellent functional and oncologic outcomes for early stage laryngeal cancer, its role has expanded to the pharynx and oral cavity. It has since become a well-established technique in the treatment of oropharyngeal tumors, as initially described by Steiner and colleagues in 2003.
A representative operating room setup for TLMS is shown in Fig. 1 . The basic instruments include a laser delivery device, binocular operating microscope, a mouth gag with tongue retractor or laryngoscope, and microlaryngeal instruments. A 10,600-nm carbon dioxide (CO 2 ) laser is frequently used. The CO 2 laser beam is absorbed by water at the tissue-laser interface and transformed into thermal energy, which results in precise tissue cutting. The depth of laser penetration is superficial and thereby predictable. By adjusting the focal length of the laser, its ability to cut rather than photocoagulate or ablate tissue can be varied. The laser becomes a versatile tool by further altering the power, spot size, and duration of pulse. The laser has recently become coupled to computer systems that control the shape, size, and pattern of the beam, allowing for precise, reproducible movements.
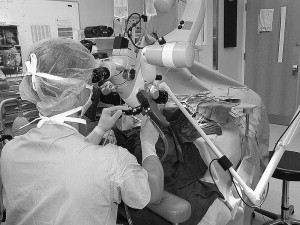
The laser has traditionally been coupled to the operating microscope. The linear path of the laser from the microscope creates line-of-sight limitations with the inability to see or deploy the laser around curved surfaces. This limitation is particularly challenging for tumors of the base of the tongue, where only the most anterior edge or protrusion of the lesion is visualized and accessible by the laser. Within the last 5 years, fiberoptic delivery systems for CO 2 and other lasers have been developed. Line-of-sight restraints are partially avoided with the fiberoptic lasers and flexible delivery instruments, which allow greater angles of approach to tumor resection. Rather than aiming the laser from the microscope to a distant point within the patient’s mouth, the tip of the laser device is placed within the oropharynx and allows greater freedom of movement.
Excellent technical accounts of TLMS surgical approaches to the oropharynx have been discussed elsewhere and are beyond the scope of this article. One characteristic particular to TLMS is that line-of-sight limitations, narrow field of vision, and tumor size require that most tumors are removed piecemeal after bisecting the lesion. Although initially anathema to the principles of head and neck surgical oncologists, the oncologic safety of this practice has been substantiated with long-term follow-up showing equivalence to en bloc resections. Both piecemeal and en bloc resections have been described to achieve negative margins with minimal injury to normal tissue. Potential disadvantages of TLMS include a steep learning curve and the use of only 1 hand to hold an instrument while the other manipulates the laser.
There are also unique safety concerns to the patient and operating room staff when delivering the intense, focused energy of laser devices. Staff are susceptible to errant laser beams and must wear eye protection. The patient also is at risk of inadvertent burns. Damp towels should cover the eyes and exposed skin of the patient’s head and neck, and also reflective surfaces (eg, metallic laryngoscope, suspension bar). A laser endotracheal tube and close communication with anesthesia is mandatory to help prevent the most feared complication of airway fire.
Transoral Robotic Surgery
The first surgical application of a robot was in 1985 when the PUMA 560 placed a needle for brain biopsy by computed tomography guidance. The first robotic system, known as Automated Endoscopic System for Optimal Positioning (AESOP), was approved by the US Food and Drug Administration (FDA) in 1993. It provided a robotically controlled arm to manipulate an endoscope for laparoscopic surgery. This device led to the evolution of the da Vinci Surgical System (Intuitive Surgical, Inc, Sunnyvale, CA), which was FDA approved for abdominal laparoscopic surgery in 2000 and for transoral otolaryngology surgical procedures restricted to T1 and T2 benign and malignant lesions in 2009.
The robot consists of several key components ( Fig. 2 ):
- •
Surgeon console
- •
Patient-side cart
- •
Vision system
- •
Endowrist instruments.
The surgeon console provides a three-dimensional (3D), high-definition image of the operative field and the master controls for the robotic arms and video endoscope. It is positioned at a distance from the patient. The patient-side cart is positioned next to the patient and includes 3 or 4 robotic arms delivering the surgical instruments and video endoscope. This setup is a master-slave system, and surgeon input is required for all robotic movement. The vision system includes a 3D high-definition 0° or 30° endoscope coupled to an image processing tower and monitor for the operating room staff and assisting surgeon. The endowrist instruments are articulating wristed instruments with 7° of freedom in both 8-mm and 5-mm sizes. A typical setup for TORS is shown in Fig. 3 . The table is rotated 180° from anesthesia. The patient is appropriately relaxed to allow the placement of a mouth gag and suspension. Three of the robotic arms (scope in the center flanked by right and left 5-mm effector arms) are advanced through the mouth. A 0° or 30° scope can be used for tonsil resections and a 30° scope affords optimal visualization of the tongue base. The assisting surgeon sits at the head of the bed and uses an instrument (typically a suction, cautery, retractor, or clip device) in each hand, which allows for 2 surgeons and 4 instruments working at the same time in the oropharynx.
TORS provides the surgeon with:
- •
A 3D, high-definition view of the operative field from the perspective of being inside the mouth of the patient
- •
A 30° maneuverable scope that affords a wide field of vision, including around the tongue base
- •
Wristed instruments with tremor-filtration, motion scaling, and 540 degrees of motion to provide precise bimanual tissue manipulation in areas heretofore inaccessible
- •
Capability of en bloc excision of large oropharyngeal tumors, which some surgeons favor more than TLMS
- •
No constraints of line-of-sight issues and 1-handed surgery as with TLMS.
Disadvantages include the high cost of the robotic system, logistics of sharing the robot among multiple surgical specialties, need to stage the neck dissection, and training and credentialing requirements. Notable advantages and disadvantages of TORS and TLMS are identified in Table 2 .
Advantages | Disadvantages |
---|---|
TORS | |
3D, high-resolution image | Lack of tactile feedback |
Overcomes line-of-sight constraints | Cost of robotic system |
Wide field of view | Sharing robot with other services |
Bimanual manipulation | Training and credentialing requirements |
Tremor-filtration, motion scaling | — |
Allows for en bloc resection | — |
TLMS | |
Ease of startup | Narrow field of view |
Precise binocular vision | Single-hand surgery |
Ability to differentiate normal from abnormal tissue with laser | Steep learning curve Line-of-sight limitations |
Ability to reach larynx/hypopharynx | Laser safety issues |
Current minimally invasive surgical approaches
Minimally invasive surgery is designed to reduce morbidity and mortality without compromising oncologic outcomes. The ideal minimally invasive approach to the oropharynx is via a transoral approach, thereby limiting tissue dissection, disruption of speech and swallow musculature, minimizing blood loss, avoiding major neurovascular structures, and limiting injury to normal tissue. Transoral approaches to the oropharynx have traditionally been limited to those tumors that can be directly visualized and manipulated with standard instrumentation and lighting. Because of line-of-sight limitations for tumors in the base of the tongue, transoral approaches had been reserved for smaller lesions of the tonsil and palate. However, in the last 20 years, innovation in surg tech has greatly expanded the ability to resect tumors of the oropharynx via a transoral approach ( Table 1 ). Transoral laser microsurgery (TLMS) and transoral robotic surgery (TORS) are the principal minimally invasive transoral endoscopic approaches used for the oropharynx to currently.
Inclusion | Exclusion |
---|---|
T1/T2 oropharynx lesions | Involvement of carotid artery, prevertebral fascia, mandible |
NO, N1, N2a, N2b neck disease | Distant metastasis |
Patients who will definitively need chemoradiation despite surgical intervention (obvious extracapsular nodal extension, low likelihood of obtaining negative margins at the primary site) | |
Extensive unilateral or bilateral soft palate involvement | |
Extension of disease into the nasopharynx | |
Tonsillar lesions with extension along the posterior pharyngeal wall past the midline | |
Deep base of tongue lesions with extension to the contralateral side | |
T4 lesions | |
Trismus or other anatomic factors precluding transoral access to the oropharynx | |
Possible Operative Candidates | — |
T3 disease | — |
Cystic N3 disease | — |
HPV-negative oropharynx tumors may best be treated by surgery followed by chemotherapy and radiation, given their poor prognosis | — |
TLMS
Technologic advancements in both binocular microscopy and surgical lasers made precise tissue cutting with minimal blood loss possible. In addition, the recognition that tumors in the upper aerodigestive tract need not be removed in continuity with cervical lymph nodes, and that they may be removed piecemeal without resultant tumor dissemination, led to the development of TLMS in the 1960s and 1970s. Since its inception in Europe, where TLMS showed excellent functional and oncologic outcomes for early stage laryngeal cancer, its role has expanded to the pharynx and oral cavity. It has since become a well-established technique in the treatment of oropharyngeal tumors, as initially described by Steiner and colleagues in 2003.
A representative operating room setup for TLMS is shown in Fig. 1 . The basic instruments include a laser delivery device, binocular operating microscope, a mouth gag with tongue retractor or laryngoscope, and microlaryngeal instruments. A 10,600-nm carbon dioxide (CO 2 ) laser is frequently used. The CO 2 laser beam is absorbed by water at the tissue-laser interface and transformed into thermal energy, which results in precise tissue cutting. The depth of laser penetration is superficial and thereby predictable. By adjusting the focal length of the laser, its ability to cut rather than photocoagulate or ablate tissue can be varied. The laser becomes a versatile tool by further altering the power, spot size, and duration of pulse. The laser has recently become coupled to computer systems that control the shape, size, and pattern of the beam, allowing for precise, reproducible movements.
The laser has traditionally been coupled to the operating microscope. The linear path of the laser from the microscope creates line-of-sight limitations with the inability to see or deploy the laser around curved surfaces. This limitation is particularly challenging for tumors of the base of the tongue, where only the most anterior edge or protrusion of the lesion is visualized and accessible by the laser. Within the last 5 years, fiberoptic delivery systems for CO 2 and other lasers have been developed. Line-of-sight restraints are partially avoided with the fiberoptic lasers and flexible delivery instruments, which allow greater angles of approach to tumor resection. Rather than aiming the laser from the microscope to a distant point within the patient’s mouth, the tip of the laser device is placed within the oropharynx and allows greater freedom of movement.
Excellent technical accounts of TLMS surgical approaches to the oropharynx have been discussed elsewhere and are beyond the scope of this article. One characteristic particular to TLMS is that line-of-sight limitations, narrow field of vision, and tumor size require that most tumors are removed piecemeal after bisecting the lesion. Although initially anathema to the principles of head and neck surgical oncologists, the oncologic safety of this practice has been substantiated with long-term follow-up showing equivalence to en bloc resections. Both piecemeal and en bloc resections have been described to achieve negative margins with minimal injury to normal tissue. Potential disadvantages of TLMS include a steep learning curve and the use of only 1 hand to hold an instrument while the other manipulates the laser.
There are also unique safety concerns to the patient and operating room staff when delivering the intense, focused energy of laser devices. Staff are susceptible to errant laser beams and must wear eye protection. The patient also is at risk of inadvertent burns. Damp towels should cover the eyes and exposed skin of the patient’s head and neck, and also reflective surfaces (eg, metallic laryngoscope, suspension bar). A laser endotracheal tube and close communication with anesthesia is mandatory to help prevent the most feared complication of airway fire.
Transoral Robotic Surgery
The first surgical application of a robot was in 1985 when the PUMA 560 placed a needle for brain biopsy by computed tomography guidance. The first robotic system, known as Automated Endoscopic System for Optimal Positioning (AESOP), was approved by the US Food and Drug Administration (FDA) in 1993. It provided a robotically controlled arm to manipulate an endoscope for laparoscopic surgery. This device led to the evolution of the da Vinci Surgical System (Intuitive Surgical, Inc, Sunnyvale, CA), which was FDA approved for abdominal laparoscopic surgery in 2000 and for transoral otolaryngology surgical procedures restricted to T1 and T2 benign and malignant lesions in 2009.
The robot consists of several key components ( Fig. 2 ):
- •
Surgeon console
- •
Patient-side cart
- •
Vision system
- •
Endowrist instruments.
