Purpose
To evaluate histologic and molecular changes in human keratoconic corneas after the procedure of transepithelial collagen cross-linking (CXL), without the removal of corneal epithelium.
Design
Experimental laboratory investigation.
Methods
Thirty corneal buttons were examined, 18 of which were from patients affected by severe keratoconus and submitted to penetrating keratoplasty (PK). Among these, 8 were analyzed without any treatment, 4 were treated with transepithelial CXL 2 hours before PK, and 6 were treated with transepithelial CXL 3 months before PK. Twelve normal corneal buttons from healthy donors were used as controls. The corneal buttons were then evaluated by hematoxylin-eosin staining and by immunostaining with markers of epithelial junction proteins (ß-catenin and connexin 43), of stromal keratocytes (CD34), of apoptosis (terminal deoxynucleotidyl transferase dUTP nick end labeling [TUNEL] assay), and of collagen type I fibers.
Results
The analysis of epithelial markers showed a clear defective expression in keratoconic corneas before and soon after the transepithelial CXL treatment, returning to normal in corneas analyzed 3 months after transepithelial CXL. The analysis of stroma components indicated a loss of keratocytes in the upper stroma of keratoconic corneas and a trend toward a normal situation 3 months after transepithelial CXL; similarly, collagen fibers appeared disorganized in keratoconus, while their pattern appears to be close to normal 3 months after treatment.
Conclusions
Histologic and immunohistochemical findings on human keratoconic corneas showed the presence of biochemical and morphologic alterations in the epithelium and the upper stroma that are significantly improved 3 months after transepithelial CXL. However, further studies are necessary to assess to what extent these results correlate with measurable biomechanical effects.
Keratoconus is a noninflammatory dystrophy of the cornea, usually bilateral, characterized by axial thinning, fragmentation of the epithelial basement membrane, breaks and scarring at the level of the Bowman membrane, keratocyte alteration, and, ultimately, stromal scarring. Typically, the condition starts at puberty, progressing in approximately 20% of the cases to such an extent that surgical procedures become necessary.
A new method has been developed for the treatment of progressive keratoconus: corneal collagen cross-linking (CXL), which increases the stiffness of the cornea using ultraviolet A (UVA) and riboflavin. Studies on rabbit and porcine eyes have shown after CXL a 70% increase in corneal rigidity in treated vs untreated corneas, and similar studies on human corneas showed a 328.9% increase in stiffness. Keratocyte apoptosis has been suggested to be important for replacement of defective cells and the formation of better-structured collagen fibers, as confirmed by in vivo confocal microscopy studies. Recently, a modified method called transepithelial cross-linking has been introduced as a promising therapy for keratoconus patients to stiffen the cornea without epithelial debridement, using a new riboflavin solution able to penetrate and cross the epithelial barrier. However, the real efficacy of this treatment is still under debate, although some clinical studies have shown encouraging results. Mazzotta and associates reported in vivo confocal microscopy studies in which a limited apoptotic effect was visible as compared to the classic epi-off CXL procedure, whereas Filippello and associates demonstrated in a clinical study a significant arrest of keratoconus progression evidenced after transepithelial CXL, with a statistically significant improvement in visual acuity and topographic parameters. However, histologic evaluations of transepithelial CXL results in human corneal architecture are necessary to ascertain the effects of treatment.
The purpose of the current study was to investigate the morphologic findings in human corneas treated with transepithelial CXL before penetrating keratoplasty (PK), and to compare these findings with normal corneas and untreated keratoconic corneas. More specifically, we analyzed the effects of transepithelial CXL on epithelial cells, keratocytes, and collagen fibers.
Methods
Materials
This was an experimental laboratory investigation on human corneal samples. Human corneal samples were collected and divided into 4 study groups: (1) 12 cadaver normal corneas (donors aged 35-55 years) from Lucca Eye-Bank as untreated normal controls; (2) 8 corneal buttons with keratoconus (donors 23-38 years old) removed after PK and used as untreated pathologic samples; (3) 4 corneal buttons with keratoconus (donors 23-32 years old) treated with transepithelial CXL 2 hours before PK (2h transepithelial CXL); (4) 6 corneal buttons with keratoconus (donors 22-38 years old) treated with transepithelial CXL 3 months before PK (3m transepithelial CXL).
All patients were affected by severe keratoconus without stromal scarring. Patients were informed that the transepithelial CXL procedure could not restore their vision and avoid PK that had already been previously scheduled. Patients who had previous corneal surgery, intracorneal ring implants, or a history of delayed wound healing were excluded. The Careggi Hospital (University of Florence) Ethics Committee approved the study, and all patients provided written informed consent for the treatment and for the participation in the research, after receiving a detailed description of the nature of the treatment. Practice and research were in accordance with the tenets of the Declaration of Helsinki.
Transepithelial CXL was performed under sterile conditions applying an enhanced aqueous riboflavin solution, composed by riboflavin phosphate 0.1%, dextran T500, and the enhancers trometamol (tris[hydroxymethyl]aminomethane) and EDTA (ethylenediaminetetraacetic acid) sodium salt (Ricrolin TE; Sooft, Montegiorgio, Italy) on the intact corneal epithelium for 30 minutes before irradiation with UVA (370 nm at 3 mW/cm 2 ) for another 30 minutes. Trometamol is a biologically inert low-toxicity amino alcohol present as buffering solution in a wide range of cosmetic products and as an alkalinizing agent in pharmaceutical drugs. Sodium EDTA is a well-known chelator of calcium and magnesium ions, which are known to be important in keeping the integrity of tissues, including the epithelial tissue. These enhancers, by weakening epithelial intercellular junctions, help riboflavin to penetrate the corneal stroma through an intact epithelium, thereby avoiding the need for epithelial debridement.
Three days before transepithelial CXL treatment, patients were administered single-dose eye drops of netilmicin 0.3%, 1 drop 4 times a day, for prophylactic purposes. The enhanced riboflavin solution was instilled 30 minutes before UVA exposure, with 1 drop being instilled every 10 minutes thereafter. To reduce the risk for UVA exposure of retroirideal eye structures, miosis was induced with pilocarpine 1.0% 30 minutes before the procedure. Twenty minutes before UVA irradiation, the cornea was anesthetized with single-dose anesthetic eye drops (oxybuprocaine hydrochloride 0.2%), 1 drop every 5 minutes. The UVA source (Vega; CSO, Florence, Italy) was switched on 2 hours before the procedure. All treatments were performed with the power set at 2.9-3.0 mW/cm 2 and a circular spot diameter of 8.0 mm in an outpatient setting. The patient was asked to lie down in a reclining armchair, and the periocular skin was disinfected for 5 minutes with povidone-iodine diluted to 10.0%. To improve riboflavin penetration, a ring-shaped silicone container was designed and used. The ring container was 12.0 mm in diameter and 3.0 mm high with a flange 2.0 mm wide and 0.3 mm thick at its base. Once covered by the eyelid, the flange stabilizes the ring on the cornea. The elasticity of the cylinder, which is rigid enough to resist the pressure of the eyelid, allows minimum lid adjustments. The outer edge of the cylinder and the flange also protect the corneal limbus and its stem cells from accidental UVA irradiation. The silicone ring was filled with 2 drops of the enhanced riboflavin solution in direct contact with the corneal epithelium to cover the corneal apex entirely ( Figure 1 ). If an attempted blink caused part of the product to spill from the ring, more enhanced riboflavin solution was instilled until riboflavin was homogeneously dispersed over the corneal apex and its epithelial layer. The silicone ring filled with enhanced riboflavin solution was left in direct contact with the cornea for 30 minutes before the cornea was irradiated with UVA for 30 minutes. During the irradiation, a homogeneous level of the enhanced riboflavin solution was constantly maintained by adding 1 drop every 3-5 minutes. In contrast to the initial permeation phase, during the irradiation phase, riboflavin formed only a thin layer over the epithelium without building up. Thus, UVA rays were not prevented from penetrating the stroma, shielded by the corneal epithelium. A simple adhesive sterile plaster was positioned on the outer edge to collect any drops of the enhanced riboflavin solution leaking from the corneal ring during the procedure. At the end of the procedure, the silicone ring was removed and all residue of the enhanced riboflavin solution was rinsed away with a sterile physiologic salt solution. Immediately postoperatively, the treated eye was medicated with 1 drop of single-dose norfloxacin. The patient was given a prescription for single-dose netilmicin, 1 drop 3 times a day for a week; and sodium hyaluronate 0.15%, 1 drop 3 times a day for 20 days.
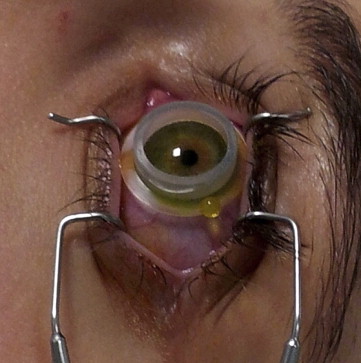
PK of CXL-treated corneas was performed 2 hours or 3 months after the transepithelial CXL procedure. For all 3 keratoconus groups and for normal corneas, we analyzed central corneal buttons of 7 mm diameter. Immediately after removal, the corneal buttons were cut in small pieces that were fixed in formalin solution, routinely processed, embedded in paraffin, and used for immunohistochemistry and morphologic detail examination (hematoxylin-eosin staining).
Immunohistochemical Studies
Immunohistochemical studies were performed on tissue sections, as previously described. Briefly, the slides were stained by the indirect immunoperoxidase technique using as primary antibodies the mouse monoclonal antibodies anti-CD34 (1:100 dilution; Dakopatts, Carpinteria, California, USA), anti-collagen type I (1:500 dilution; Sigma-Aldrich, St. Louis, Missouri, USA), anti-ß-catenin (1:100 dilution; Santa Cruz Biotechnology, Santa Cruz, California, USA), and anti-connexin-43 (1:100 dilution; Sigma-Aldrich). The diameter of the collagen fibers in the anterior stroma was measured by a computerized image analyzer program (ImageJ 1.38x, W.S. Rasband, U. S. National Institutes of Health, Bethesda, Maryland, USA) on 15 fields for slide. Computer-assisted quantification of CD34, ß-catenin, and connexin-43 (Cx43) was performed using Adobe Photoshop 6.0 software (Adobe System Incorporated, San Jose, California, USA).
Terminal Deoxynucleotidyl Transferase dUTP Nick End Labeling Assay
Apoptotic cells were identified using Fragel DNA Fragmentation Detection Kit, Fluorescent – TdT Enzyme (Calbiochem, San Diego, California, USA). Paraffin-embedded tissues were deparaffinized and subjected to the terminal deoxynucleotidyl transferase dUTP nick end labeling (TUNEL) assay according to the manufacturer’s protocol, as previously described. The numbers of apoptotic cells were counted in 10 separate fields of 4 slides.
Statistical Analysis
The results were expressed as mean ± SD. Statistical analysis was performed by the Mann-Whitney test or by analysis of variance (ANOVA) with post hoc test (Bonferroni correction), as appropriate. P < .05 was considered significant. All statistical analyses were carried out using SPSS software version 15.0 (SPSS Inc, Chicago, Illinois, USA).
Results
Morphologic Details
Morphologic studies with hematoxylin-eosin staining showed a thinning of the corneal thickness and a reduction of 33.2% in keratocytes number in all keratoconus specimens ( Figure 2 , Top middle-left) with respect to control ( Figure 2 , Top left and Bottom; Table ). In 2h transepithelial CXL corneas ( Figure 2 , Top middle-right), the outer layers of the epithelium were lost, likely because of the effect of the enhancers in the transepithelial riboflavin formulation, but the deeper layers were well preserved; the stroma still showed a reduction of keratocytes ( Figure 2 , Bottom; Table ) (reduction of 32.2%). In 3m transepithelial CXL corneas ( Figure 2 , Top right) a normal multilayered epithelial structure was again detected, and keratocyte density increased (increase of 30.7% vs keratoconus and 29.7% vs 2h transepithelial CXL), even though still lower than in control healthy corneas ( Figure 2 , Bottom; Table ) (reduction of 2.5%).
Specimens | Epithelium | Stroma | ||||
---|---|---|---|---|---|---|
ß-Catenin | CX43 | Keratocytes | CD34 | TUNEL | Collagen I | |
Control | 100 ± 15.54 | 100 ± 10.94 | 100 ± 5.9 | 100 ± 28.02 | 100 ± 65 | 100 ± 12.56 |
Keratoconus | 70.17 ± 9.47 a | 63.57 ± 6.07 a | 66.78 ± 8.5 a | 46.5 ± 12.88 a | 400 ± 90 a | 64.67 ± 5.1 a |
2h TE-CXL | 58.46 ± 9.45 a | 43.61 ± 4.88 a | 67.8 ± 8.9 a | 42.26 ± 9.19 a | 650 ± 120 a | 66.72 ± 4.18 a |
3m TE-CXL | 100.23 ± 19.74 | 87.72 ± 17.55 b | 97.5 ± 6.4 | 98.13 ± 13.65 | 300 ± 70 a , c | 89.8 ± 18 |
Immunohistochemistry
Epithelium
ß-Catenins are multifocal proteins involved in cellular junctions and pivotal members of signal transduction pathways. Immunohistochemical analysis of ß-catenin expression in the epithelium showed a uniform labeling in untreated control corneas ( Figure 3 , Top left), whereas in keratoconic corneas only few epithelial cells showed immunoreactivity for ß-catenin ( Figure 3 , Top middle-left). In 2h transepithelial CXL corneas, ß-catenin immunostaining in the preserved epithelial layers was weak and scattered ( Figure 3 , Top middle-right). Finally, in 3m transepithelial CXL corneas high ß-catenin expression was detected in the whole epithelium ( Figure 3 , Top right), similar to untreated control corneas. Quantitative analysis ( Figure 3 , Bottom; Table ) indicated a significant relative decrease of the immunostaining in keratoconus compared with control corneas ( P < .001) and 3m transepithelial CXL ( P < .001). Such staining for ß-catenin decreased even further in 2h transepithelial CXL corneas ( P < .001).
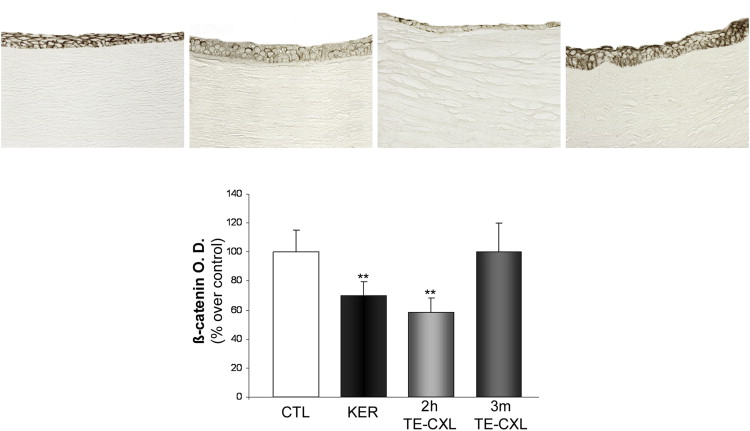
Connexins are a polygenic family of transmembrane proteins that form gap junction channels. These gap junctions permit the direct cell-to-cell exchange of ions, secondary messengers, water, electrical impulses, metabolites, and nutrients, and thus mediate intercellular functional communication among corneal epithelial cells.
Epithelial cells of control corneas ( Figure 4 , Top left) strongly expressed Cx43 throughout the epithelium. Keratoconic corneas ( Figure 4 , Top middle-left) exhibited a decreased and scattered immunolabeling pattern, and in 2h transepithelial CXL corneas ( Figure 3 , Top middle-right) Cx43 immunolabeling was observed only sporadically in the epithelium. In 3m transepithelial CXL corneas ( Figure 4 , Top right), Cx43 expression was characterized by an immunostaining pattern similar to control corneas. Quantitative analysis ( Figure 4 , Bottom; Table ) indicated that the lowest amount of Cx43 expression was present in 2h transepithelial CXL ( P < .001); 3m transepithelial CXL showed an immunopositivity stronger than keratoconus ( P < .001), though still lower with respect to control corneas ( P < .01).
Stroma
Keratocytes, the main stromal cells, play an important role in the preservation of corneal transparency and mechanical stability through the synthesis and maintenance of the collagen component. Keratocyte distribution in normal and keratoconic corneas has been evaluated by CD34 staining. CD34 is a glycosylated type I transmembrane protein recently used as a specific marker of keratocytes in human corneas. In control corneas ( Figure 5 , Upper row left and Lower row left), keratocytes were found throughout the corneal stroma, whereas in keratoconus ( Figure 5 , Upper row middle-left and Lower row middle-left) a patchy loss of CD34 immunoreactivity was observed mainly in the anterior part of the cornea (decrease of 53.5%). In 2h transepithelial CXL corneas ( Figure 5 , Upper row middle-right and Lower row middle-right), a decrease of 57.74% in CD34 cell positivity was present in the anterior stroma, while in 3m transepithelial CXL corneas ( Figure 5 , Upper row right and Lower row right) the CD34 positivity was regularly detected in keratocytes of the all corneal stroma showing a distribution similar to control. Quantitative analysis of CD34 staining ( Figure 5 , Bottom; Table ) showed no significant difference between 3m transepithelial CXL and control, while a significant increase of the immunoreactivity was evident when compared with keratoconus ( P < .001) and 2h transepithelial CXL corneas ( P < .001).
