(1)
Charlotte Eye Ear Nose & Throat Associates, Charlotte, NC, USA
Abstract
The 4-aminoquinolines are predictably toxic at high enough concentrations. Toxicity in all tissues derives from derangement of lysosomal function and is dose dependent. Chloroquine is more toxic than hydroxychloroquine. The sensitivity of different cell types varies, but all cells exhibiting toxic effects accumulate myelin bodies.
Abbreviations
ABW
Actual body weight
4AQs
4-Aminoquinolines (chloroquine and hydroxychloroquine)
4AQR
4-Aminoquinoline retinopathy
A2E
N-retinylidene-N-retinylethanolamine
C
Chloroquine
C-tubes
Curvilinear tubules
d
Day
ED50
Effective dose 50
EOG
Electrooculogram
ERG
Electroretinogram
GCL
Ganglion cell layer
g
Gram
HC
Hydroxychloroquine
IBW
Ideal body weight
IM
Intramuscular
INL
Inner nuclear layer
IP
Intraperitoneal
IPL
Inner plexiform layer
IV
Intravenous
kg
Kilogram
L
Liter
LD50
Lethal dose 50
M
Mole
MCB
Membranous cytoplasmic body
mfERG
Multifocal electroretinography
mg
Milligram
μM
Micromoles/L
nm
Nanometer
NSAID
Nonsteroidal anti-inflammatory drug
PR
Photoreceptor
RA
Rheumatoid arthritis
RPE
Retinal pigment epithelium
TD50
Toxic dose 50
The 4-aminoquinolines (4AQs) are thought to be universally toxic under the proper circumstances, although there may be individual genetic factors that modulate risk [1, 2]. That is, the toxic effects on the retina are not idiosyncratic and can be reproducibly observed if a high enough dose of these drugs is given for a long enough period [1]. This is true in animal models and in clinical studies in humans. There is a gradation of toxicity, however, so that clinical retinopathy occurs in a small subset of patients if appropriate dosing by ideal body weight (IBW) is observed [3]. This chapter covers the toxicology of the 4AQs and their histopathologic effects. The remainder of the book is devoted to 4-aminoquinoline retinopathy (4AQR), beginning with its many definitions in Chap. 4, following with epidemiology in Chap. 5, and natural history in Chap. 6. Commonly used abbreviations are collected in “Abbreviations” for reference. Each term will be first used in its full form, along with its abbreviation.
Although the 4AQs are derived from quinine, their toxicities differ from the parent compound. Acute quinine toxicity is characterized by a macular cherry-red spot, electroretinogram (ERG) changes including a reduced b-wave, and a depressed electrooculogram (EOG) Arden ratio [4]. Acutely the retinal vessels have normal caliber [4, 5]. Later optic atrophy develops with narrowed arterioles and pigmentary changes in the retina [4, 5]. A bull’s-eye maculopathy does not develop, in contrast to 4AQR.
The toxicity of chloroquine and hydroxychloroquine is directly related to the 4AQ nucleus, modulated by various side-chain substitutions. Halogen substitutions at any position other than seven (see Chap. 2, Fig. 2.1) reduce pharmacologic activity and toxicity [6]. An aryl rather than an alkyl side chain decreases the therapeutic ratio [6]. Increasing alkyl side-chain length above five carbons decreases the therapeutic ratio and increases toxicity [6]. The 8-aminoquinolines primaquine and quinocide lack the 4AQ nucleus and cause no retinopathy [7]. Quinacrine is not a 4AQ, but rather a derivative of an acridine nucleus (see Chap. 2, Fig. 2.1). For years it was thought not to cause retinopathy [8]; in fact, it had been suggested as a substitute for patients who had discontinuation of 4AQs over concerns regarding retinopathy [8]. Unfortunately quinacrine retinopathy has been reported more recently characterized by a bull’s-eye macular lesion indistinguishable from that of 4AQR [9, 10]. However, the prevalence of retinopathy in quinacrine users is much lower [9]. It is probably unwise to place a patient with 4AQR on quinacrine, although this action has been suggested when the mechanism of action of antimalarials is desired with a lesser risk of retinopathy [11].
3.1 Toxic Concentrations
Toxicity has been studied in several different ways, including dose–response curves for lethality in animal models and dose–response curves for frequency of side effects in clinical studies. In a retinal pigment epithelium (RPE)-19 cell culture model of chloroquine toxicity, no toxicity was present at a concentration of 10 micromolar (μM), 100 % toxicity occurred at 250 μM, and the mean dose at which 50 % of cells were killed (lethal dose 50, LD50) was 120 μM [12]. In an intravenous-dosing model in mice, the LD50 for chloroquine was 25 mg/kg and was 45 mg/kg for hydroxychloroquine [13]. This order of toxicity is also seen in an intravenous-dosing model in dogs. It is not seen in rabbits, in which the two drugs reach LD50 at similar doses [13]. From cases of suicide, the lethal dose of chloroquine in the adult human is estimated to be 2.5–6 g and 0.75–1 g in a child [14–16]. The lethal dose of hydroxychloroquine is less well established than that of chloroquine, but based on data from animal models it is estimated to be 2–3 times that of chloroquine [15]. One patient survived ingestion of 5.8 g with a plasma level of 1.8 × 10−5 M/L [13]. Death from 4AQ poisoning occurs after hypotension, apnea, and convulsions. The toxicity may be reversible if life support is provided [14].
In clinical practice, the frequency of side effects depends on serum and blood concentrations. In adults, none were noted for serum concentrations of chloroquine below 4.5 × 10−7–1.25 × 10−6 M/L but occurred in 80–89 % of patients at serum concentrations above 2.5 × 10−6 M/L [15, 17, 18]. The incidence of gastrointestinal side effects in weeks 1–3 of therapy was related to hydroxychloroquine concentration in the blood in one study. The frequency ranged from less than 10% of patients with blood concentrations below 2.2 × 10−6 M/L to 30 % of patients with concentrations of 4.5 × 10−6 M/L and above [19]. In children, side effects were not noted for chloroquine concentrations below 8.75 × 10−7 M/L or for hydroxychloroquine concentrations below 1.40 × 10−6 M/L [20]. A maximum safe serum concentration has been estimated to be 8.75 × 10−7 M/L for chloroquine and 1.4 × 10−6 M/L for hydroxychloroquine [15].
Although it has been stated that the side effects of the 4AQs depend on the serum concentration and not on the cumulative dose [21], such a view does not account for the evidence supporting cumulative dose as a risk factor for retinopathy (see Chap. 7). Retinopathy and other forms of toxicity depend strongly on drug concentration, and hence daily dose; it is rarely seen in patients taking 4AQs for malaria prophylaxis in which dosing is much less than in patients taking the drugs for chronic autoimmune diseases [22, 23].
Plasma concentrations of 4AQs are rarely measured in clinical practice, but abundant information has been collected for daily dosage, a crude surrogate rendered cruder when used in the absence of information on IBW (see Chap. 7). Based on numerous retrospective case series, the current best estimates for dose thresholds at which risk of toxicity increases are 3 and 6.5 mg/kg/day based on IBW (Table 3.1). These thresholds are not optimal dosages, but ceilings above which dosing should not exceed [14]. The similar concept of threshold cumulative dose is analogous to the threshold concentration or adjusted daily dose at which risk of toxicity increases. Unlike the case of adjusted daily dose, there is no clear consensus about what the inflection point might be (see Chap. 7).
Table 3.1
Suggested thresholds at which the risk of retinopathy increases
Drug | Daily dose (mg/kg) | Study |
---|---|---|
Chloroquine | 3.0 (IBW)/day | |
3.5–4.0 | Ehrenfeld et al. [30] | |
<3.74 (ABW)/day | Mackenzie [31] | |
3.85 | Fleck et al. [32] | |
4.0 (IBW)/day | ||
4.0 is “too high” | Easterbrook [35] | |
4.0 (ABW)/day | ||
5.35 (IBW)/day | Mackenzie [31] | |
Hydroxychloroquine | 5.0–7.0 | Laaksonen et al. [20] |
6.0 | Scherbel [36] | |
6.15 | Fleck et al. [32] | |
6.0–6.5 | Ehrenfeld et al. [30] | |
6.5 (IBW)/day | ||
7.7 (ABW)/day | Mackenzie [31] |
3.2 Mechanism of Toxicity
The mechanism of toxicity of the 4AQs is unknown, but the 4AQ nucleus is considered to be the critical factor causing toxicity. Potential mechanisms of toxicity include all the known mechanisms of action of the drug (see Chap. 2) carried to excess [13, 45–47]. In a model using RPE-19 cultured cells, chloroquine inhibited protein degradation within autophagic vacuoles, leading to accumulation of ubiquitinated proteins that might be toxic [12]. Banks has noted that intravitreal chloroquine damages photoreceptors but systemically administered chloroquine spares photoreceptors and instead preferentially damages ganglion cells followed by the RPE [48, 49]. From this observation, he hypothesized that the RPE detoxifies photoreceptors with accumulation of some by-products until a critical threshold is reached, at which point the RPE is destroyed [7]. Mechanisms of toxicity related to melanin binding have been discounted as have mechanisms of therapeutic effects (see Chap. 2). For example, 4AQR occurs in albino animal models [50]. In fact, binding to melanin may be protective rather than harmful [24].
The retinal toxicity of 4AQs may be derived from the toxic effects of metabolic by-products from photopigment recycling. Lipofuscin is an accumulation of breakdown products of visual pigments within the RPE. Lipofuscin’s main component is N-retinylidene-N-retinylethanolamine (A2E) with lesser amounts of isoA2E, all-trans-retinal dimer phosphatidylethanolamine, and all-trans-retinal dimer-E. A2E is photooxidized to peroxy-A2E, furano-A2E, and epoxides [51, 52]. These endoperoxides are potentially cytotoxic and may cause RPE damage and atrophy [51, 53, 54]. Chloroquine and hydroxychloroquine may potentiate the toxic effects by forming complexes with polar lipids, rendering these lipids indigestible and leading to excessive intralysosomal storage of the complexes [47]. These ideas are sketchy, and none has been developed into a testable hypothesis.
The maculocentric nature of 4AQR has prompted speculation of a role for light energy or the distribution of cones or rods (see Chap. 1) [24]. Short-wavelength (blue) light is more damaging to retinal neurons than longer-wavelength light. The blue light of the sky originates in the superior visual field and is focused on the inferior macula, the location of the earliest funduscopically visible damage in 4AQR. Thus, one possible mechanism of 4AQ toxicity is to unmask the susceptibility of the macula, and especially the inferior hemimacula, to the damaging effects of blue light. The relative resistance to 4AQ toxicity of the fovea compared to the perifovea has not been explained [55].
3.3 Nonretinal Ocular Toxicity
The 4AQs have nonretinal ocular toxic effects. Corneal deposits occur frequently with chloroquine use, infrequently with hydroxychloroquine use, and are reversible when the drug is discontinued. They do not spontaneously resolve as long as drug is continued [8, 14, 34, 56–67]. Rates of keratopathy reported in various series are shown in Table 3.2. The weighted mean estimates for chloroquine and hydroxychloroquine are 78 % and 2 %, respectively. The provenance of the deposits is debated. Some claim that they are salts of 4AQs, whereas others say that they are lipid deposits [68, 69]. The deposits may appear as punctate dots in the corneal subepithelium and Bowman’s layer as a fine powder-like stippling. They may also appear as a vortex figure similar to amiodarone keratopathy, with the highest concentration below the pupil (Fig. 3.1) [60, 61, 65, 70, 71]. Sometimes these changes are best seen on retroillumination and are easier to see in a patient with dilated pupils [35, 57, 72]. They can occur within 3 weeks of starting the drug, and are related to the daily dosage ingested [60, 65]. Their frequency is dose related. Twenty-eight percent of patients taking hydroxychloroquine at doses of greater than 800 mg/day had corneal verticillata, whereas at doses of 400 mg/day fewer than 5 % of patients did [8, 68]. They usually disappear within 6–8 weeks after stopping the drug, but can occasionally persist up to 2 years [58, 68].

Table 3.2
Rates of 4-aminoquinoline keratopathy in various case series
Study | Drug | N | Percentage with keratopathy |
---|---|---|---|
Hobbs et al. [73] | C | 28 | 79 |
Percival and Behrman [74] | C | 198 | 37.9 |
Hobbs et al. [65] | C | 165 | 33.3 |
Henkind and Rothfield [60] | C | 45 | 73.3 |
Araiza-Casillas et al. [75] | C | 78 | 50 |
C | ~1,500 | 90 | |
Marks and Power [76] | C | 222 | 15 |
Weighted mean rate | C | 2,014 | 78 |
Henkind and Rothfield [60] | HC | 6 | 16.6 |
Grierson [63] | HC | 758 | 0.9 |
Mantyjarvi [77] | HC | 63 | 1.6 |
Elder and Rahman [78] | HC | 262 | 0.4 |
HC | ~500 | 5 | |
Weighted mean rate | HC | 1,589 | 2 |

Fig. 3.1
This 52-year-old woman with rheumatoid arthritis was placed on hydroxychloroquine 400 mg/day for 6 months before being examined. Her slit lamp examination showed bilateral verticellate keratopathy (white arrows, panel a). She was not taking amiodarone or other drugs that are known to cause corneal deposits besides hydroxychloroquine. She was 5 ft 1 in. tall and weighed 211 lb. Her IBW was 127 lb. Thus her adjusted daily dose was 6.93 mg/kg/day—a toxic dose. She was advised that she had hydroxychloroquine deposits in her corneas that might go away with revision of her dosage to a nontoxic level. (b) For comparison, a typical example of a patient with amiodarone keratopathy is shown (yellow arrows)
Reports are inconsistent as to whether keratopathy is a risk factor for retinopathy. Presence of keratopathy was a risk factor for retinopathy in one series [75]. In others, presence of corneal deposits did not imply higher risk of retinopathy, and cases of retinopathy have developed in patients who never had keratopathy [34, 65, 79]. Few cases with retinopathy develop keratopathy. For example, none of the 16 cases with retinopathy in Michaelides’ series had corneal changes, nor any of the author’s series of cases of 4AQR [38].
Keratopathy does not occur more commonly in one autoimmune disease than another, and is not gender or age dependent [65]. Some authors state that presence of keratopathy implies overdosage and a need to reduce dosing [20, 72, 75]. Easterbrook states that this is not the case for chloroquine, but if prominent in a patient taking hydroxychloroquine it should raise the issue for more thorough investigation [68]. Corneal deposits without retinopathy are not an indication to stop 4AQs [76, 80].
Chloroquine corneal deposits are associated with symptoms of halos around lights in approximately 1 % of patients, but some observers report higher percentages [56, 65, 68]. Easterbrook has never seen a symptomatic case of hydroxychloroquine corneal deposits, nor has the author [68]. Visual acuity is not reduced in the presence of keratopathy. Decreased corneal sensitivity in association with keratopathy has been noted [59, 74]. Decreased corneal sensitivity has been reported to occur in 51.7 % of patients taking 4AQs compared to less than 10 % in a control group of nonrheumatic subjects not taking 4AQs [60]. This corneal effect need not be related to the presence of corneal deposits and typically is bilateral [60].
Cataracts have occasionally been listed as associations with 4AQ toxicity, but the relative high frequency of these in older patients makes such a link difficult to support [81, 82]. Difficulty with accommodation is common with the use of 4AQs, but often subsides over time, and is less of a problem with hydroxychloroquine than chloroquine [81–83]. Diplopia has occasionally been reported with the use of 4AQs [82]. Abducens palsy and optic atrophy have been occasionally associated with chloroquine ingestion [71]. Whitening of hair and eyelashes has been reported in 1.8 % of patients [60, 84].
3.4 Relative Toxicity of Chloroquine and Hydroxychloroquine
In animal models, the preponderance of the evidence suggests that hydroxychloroquine is less toxic than chloroquine on a weight basis (Table 3.3) [6]. For example, in rats given equal doses of hydroxychloroquine and chloroquine, growth rates have slowed more (evidence of toxicity) in the rats receiving chloroquine [85].
Table 3.3
Relative toxicity of 4-aminoquinolines
Animal model (Reference) | Mode of administration | Toxicity index chloroquine | Toxicity index hydroxychloroquine | Toxicity ratio of chloroquine to hydroxychloroquine |
---|---|---|---|---|
Mouse [6] | Acute IV | LD50 = 40 mg/kg | LD50 = 74 mg/kg | 1.85 |
Acute IP | LD50 = 73–125 mg/kg | LD50 = 300 mg/kg | 2.4–4.1 | |
Acute oral | LD50 = 250–1,000 mg/kg | LD50 = 1,000 mg/kg | 1–4 | |
Dog [6] | Acute IM | Maximal tolerated dose = 6 mg/kg | Maximal tolerated dose = 25 mg/kg | 4.2 |
Chronic oral | Maximal tolerated dose = 12 mg/kg/day × 28 weeks | Maximal tolerated dose = 32 mg/kg/day × 13 weeks | 2.7 |
The literature on toxicity in humans is inconsistent on the issue of differential toxicity of the antimalarial drugs. Although no scientifically valid studies have been done, it is the common clinical impression that chloroquine at 250 mg/day is more toxic than hydroxychloroquine at 400 mg/day [33, 38, 43, 75, 86–93]. At these doses, the rate of adverse effects observed in clinical practice is approximately half as much with hydroxychloroquine [15, 93]. Other circumstantial evidence to support the view includes the fact that 30 cases of chloroquine retinopathy appeared in the UK over a 30 year span, but only five cases of hydroxychloroquine retinopathy did [33]. The numbers of patients exposed to the two drugs were not known, but hydroxychloroquine usage was estimated to be more than one-sixth the rate of usage of chloroquine [33]. The rate of side effects increases more rapidly as dose increases with chloroquine than with hydroxychloroquine (Fig. 3.2) [20]. In RPE cell culture, hydroxychloroquine was a less potent enhancer of lipofuscinogenesis than chloroquine. The inhibition of lysosomal enzymes was less with hydroxychloroquine [37]. In some patients unable to tolerate chloroquine because of side effects, hydroxychloroquine may be tolerated [92]. The reverse is rarely the case [92]. One hypothesis advanced for the differential toxicity is that the hydroxyl group present in hydroxychloroquine but not in chloroquine gives the former less ability to penetrate the blood–retinal barrier [94, 95]. In addition, chloroquine but not hydroxychloroquine has been documented to cause breakdown of the blood–retina barrier, although if hydroxychloroquine were tested, it might also show this effect [95].


Fig. 3.2
Frequency of complications in percent related to dose of chloroquine (a) and hydroxychloroquine (b) in mg/kg/day of actual body weight. Data from Laaksonen [20]
A way to quantitate the relative toxicity of drugs involves the concept of the therapeutic ratio (see Chap. 1). The therapeutic ratio is the ratio of the toxic dose in half of the population (TD50) to the minimum dose effective in half of the population (ED50). Some have estimated that hydroxychloroquine is 50–80 % as effective as chloroquine [88, 96]. Estimates of the relative toxicity of hydroxychloroquine compared to chloroquine range from 33 to 60 % in humans and animal models [13, 14, 50, 88, 97, 98]. This can be reframed quantitatively using the concept of therapeutic ratio. Assume the ED50 of chloroquine (C) is one. If hydroxychloroquine (HC) is two-thirds as effective as chloroquine, then ED50HC = 0.67ED50C. If hydroxychloroquine is one-half as toxic, then TD50HC = .50TD50C. Therefore, the therapeutic ratio of HC is TD50HC/ED50HC = .67TD50C/.5ED50C = 1.33(TD50C/ED50C). That is, the therapeutic ratio of hydroxychloroquine is 1.33 times that of chloroquine. A higher therapeutic ratio implies greater safety.
The therapeutic ratio may depend on the particular toxicity being discussed. For retinopathy, conclusions about the relative toxicity of 4AQs are inconsistent. Finbloom and colleagues reported that of 31 patients taking chloroquine with a mean dose of 329 mg/day, 6 developed retinopathy [91]. By comparison, none of the 66 patients taking hydroxychloroquine at a mean dose of 280 mg/day developed retinopathy. On the basis of this experience, he concluded that chloroquine was a more toxic drug. However, it should be considered that the mean dose of chloroquine in this series would be high for most patients based on IBW, whereas the mean dose of hydroxychloroquine would not be high for most people. That is, the conclusion of different relative toxicity cannot convincingly be made based on this evidence. Mackenzie assumed that an average therapeutic daily dosage of chloroquine was 4.0 mg/kg/day based on actual body weight (ABW) and of hydroxychloroquine was 6.5 mg/kg/day. His data in patients with retinopathy indicated an average daily dose of 5.11 and 7.77 mg/kg/day based on ABW for chloroquine and hydroxychloroquine, respectively. From these data, the therapeutic ratio was calculated to be 1.27 and 1.20 for chloroquine and hydroxychloroquine, respectively. Looked at in this way, the relative toxicity of the two compounds with respect to retinopathy was approximately equal. The worse clinical experience with chloroquine may reflect the fact that the smallest pill size for chloroquine is 78 % of a toxic dose for a person with an IBW of 60 kg, but the smallest pill size for hydroxychloroquine (200 mg) is 40 % of a toxic dose for hydroxychloroquine in a person of this IBW. Others have agreed that there is no convincing evidence of differential toxicity of the 4AQs [31, 39, 80, 99, 100]. A more nuanced view is that differential toxicity is present at doses above 4 mg/kg/day but that no differential toxicity exists at lower doses [20]. Synthesizing both animal and human data, the weight of the imperfect evidence supports the concept that chloroquine is more toxic than hydroxychloroquine. That is, the therapeutic ratio for hydroxychloroquine is higher.
3.5 Nonocular Toxicity of Chloroquine and Hydroxychloroquine
As might be expected for a biologic toxin, retinopathy is not the only manifestation of chloroquine and hydroxychloroquine toxicity. In a pigmented rabbit model of chloroquine toxicity, serum hypo- and dysproteinemia developed with decreases in serum albumin and alpha 1 and 2 globulin fractions and increases in beta and gamma globulin fractions [101]. Serum amino acid profiles changed with disappearance of tyrosine; decrease in taurine, aspartine, glutamine, tryptophan, and arginine; and increases in serine, alanine, GABA, and ornithine [101].
Most information in humans regarding the toxic side effects of the 4AQs relative to other drugs used for rheumatologic diseases comes from observational studies which are limited by confounding, variable thresholds for diagnosing side effects and different follow-up times [102, 103]. For example, if nonsteroidal anti-inflammatory drugs (NSAIDs), gold, penicillamine, and cyclophosphamide are given for rheumatological disease of different severity, then the prevalence of side effects may reflect the differences in disease severity and not the drugs used. In one study, increasing follow-up from 5 to 15 years doubled the rate of reported side effects leading to drug stoppage [102]. Prospective studies with standardized definitions of side effects yield more reliable data than retrospective observational studies. In one prospective study of patients with early rheumatoid arthritis (RA) randomized to NSAIDs, hydroxychloroquine, gold, or methotrexate, total side effects and those severe enough to cause discontinuation of drug were found in the following order: gold (65 and 30 %) > methotrexate (64 and 11 %) > hydroxychloroquine (49 and 8 %) > NSAIDS (34 % and not given) [104]. In prospective, double-blind studies, the key statistic to consider is the differential frequency of side effects between patients taking 4AQs. For example, in one such study side effects were noted in 40 % of patients in the control group, but 62.5 % of patients taking chloroquine [105].
As with ocular side effects, nonocular side effects are less frequent with hydroxychloroquine (9–17 %) than chloroquine (16–19 %) at usual clinical doses [45, 83, 102, 103]. Approximately 6–10 % of patients who begin to take chloroquine and 5–17 % of patients who begin to take hydroxychloroquine are unable to continue taking the drugs because of side effects [78, 102, 106–108]. Many of the side effects resolve spontaneously if the drug is continued, if the daily dose is divided, or if nocturnal dosing is used [103]. Because the side effects occur at similar rates in patients taking a placebo, it is difficult in some cases to tie them to the ingestion of the 4AQ [15]. The most common nonocular side effect of both 4AQs is gastrointestinal upset accompanied by cramps, nausea, and loss of weight, which has been noted in 6–12 % [75, 88, 92, 102, 106, 109, 110]. Depigmentation of hair can be noted, and can resolve spontaneously even if the drug is continued [70, 83, 84, 92, 110, 111]. Other tissues not exposed to light, such as the palate, can also become depigmented [14, 83]. Ototoxicity, neuropathy, and myopathy have been described [112, 113]. Cutaneous allergy develops in 1–3 % and may include rash or symptoms of itching, hives, and exfoliation [14, 70, 92, 106, 110]. Occasionally patients taking chloroquine develop a blue-gray discoloration of the tibia, face, and palate attributed to increased melanin and hemosiderin production [83, 99]. Psoriasis may be exacerbated [114]. Mild ileus can occur [14]. Rarely, bronchospasm has been reported [114]. Reduced amplitude of the T wave in the electrocardiogram without cardiovascular symptoms has been reported [115]. Although it is rare, leukopenia, lymphopenia, thrombocytopenia, aplastic anemia, and hemolysis have been observed [114].
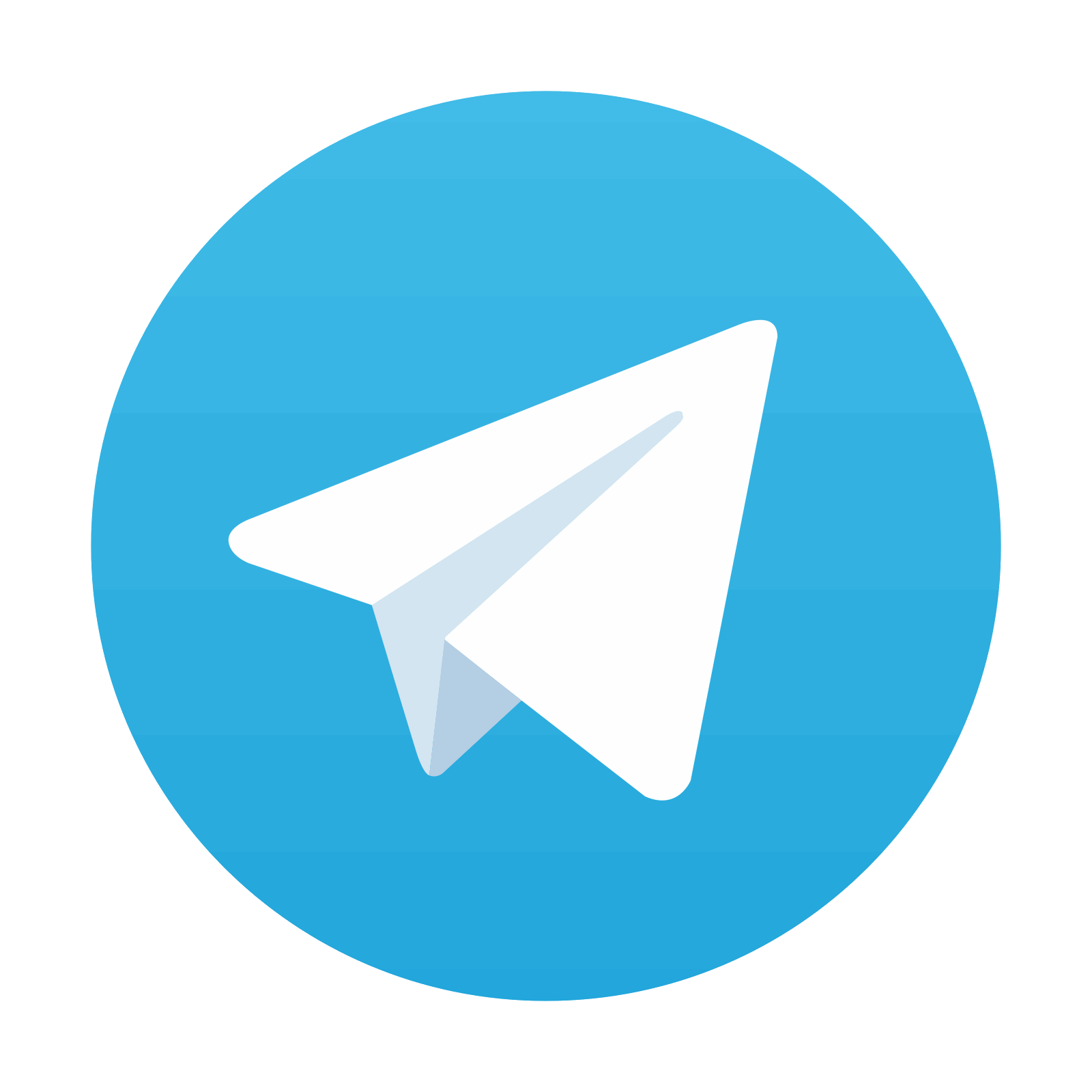
Stay updated, free articles. Join our Telegram channel

Full access? Get Clinical Tree
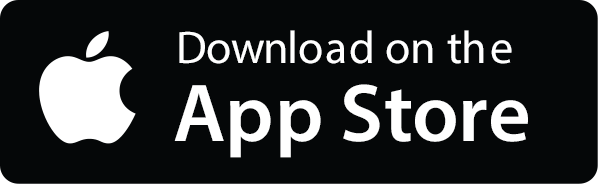
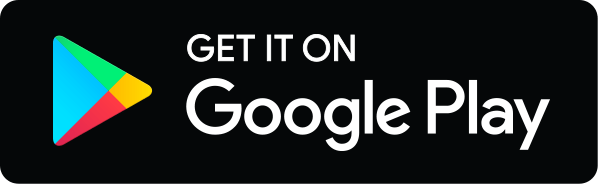