7 The State of the Art in Head and Neck Imaging This chapter will provide the clinician with an understanding of the basic principles and clinical applications of head and neck imaging. The discussion focuses on computed tomography (CT), magnetic resonance imaging (MRI), and positron emission tomography (PET), which are commonly used in the evaluation of otolaryngologic diseases. CT scanning has had a major impact on the diagnosis and management of otolaryngologic diseases. The use of computers to reconstruct radiograph attenuation data into an image allows the clinician to evaluate the anatomy and pathology from many different angles without overlying anatomical structures and to adjust the image contrast for better visualization of soft tissue and osseous pathology. There are continued advances in computer software allowing three-dimensional visualization of anatomical details and pathologic processes. CT provides high-resolution imaging using ionizing radiation. The x-ray tube delivers photons in a collimated beam to the patient, which represents the slice thickness of the image. The tube is linked to multiple detectors that collect these photons at a different rate of intensity, depending on the degree of absorption through the patient. It is rotated at many different angles to obtain different absorption patterns across various rays through a single slab of the patient. Current CT scanners have circumferential detectors moving in a 360-degree arc with continuous data acquisition and gantry rotation. Images are created through a mathematical reconstruction algorithm called filtered backprojection that adjusts the visibility of quantum mottle in images, known as “image noise.” The filtered backprojection algorithms can be customized to adjust the image noise and resolution for optimal evaluation of soft tissue or bone. Image interpretation and analysis are based on the qualitative observation of the different absorption values for various organs and their pathology. Quantitative absorption values can be obtained for each region of interest within a slice, referred to as Hounsfield units (HU). The scale for CT absorption generally ranges from +1000 to –1000 HU. The calculation of HU measurements are relative to water (designated as a zero value). Dense cortical bone measures approximately +1000 HU, and air measures –1000 HU. Generally, acute blood ranges from 56 to 76 HU, calcification is 140 to 200 HU, and fat is –30 to –100 HU. Metallic objects are at the highest HU range. Structures containing high protein concentrations will also have higher HU measurements in the range of clotted blood, such as tenacious sinus secretions, proteinaceous cysts, and the lens of the eye. CT absorption values that measure < 0 are mostly limited to air in the sinuses and fat in the neck and orbits. Intravenous administration of contrast is used in otolaryngologic imaging for improved detection and further evaluation of pathologic processes. Contrast enhancement improves detection of normal-enhancing structures, such as blood vessels in the neck, to determine their location and pathologic involvement. Pathologic processes, such as infection, inflammation, and neoplasms, will demonstrate variable uptake of contrast depending on the vascularity and permeability of these vessels. The delivery of contrast to the intravascular compartment will also affect the degree of contrast enhancement for both normal and pathologic processes. CT remains the preferred modality for clinical use in otolaryngologic imaging because of its many advantages. CT has widespread availability throughout academic, community, and private practices, with prompt accessibility 24 hours a day without prolonged scheduling delays, allowing for immediate imaging in the emergency setting. Critically ill and claustrophobic patients are able to tolerate CT imaging because of its rapid image acquisition time and patient accessibility. Patients who are not able to undergo MRI due to incompatibility from indwelling devices, such as pacemakers, inferior vena cava filters, and mechanical pumps, are candidates for CT scanning. There are few patient contraindications for CT scanning. In comparison to MRI, CT has the ability to limit image distortion artifact from surgical clips or implantable devices by changing the angle or level of scanning. There are software programs available for quick and easy postprocessing of the acquired images to aid in the visualization and interpretation of otolaryngologic diseases. Helical scanning allows for the volume of tissue scanned to be further reconstructed into thinner sections or imaging planes, known as multiple planar reformats. Reconstructions can be performed in any imaging plane with varying degrees of obliquity to obtain specific information. An example is the Poschl view of the petrous temporal bone used to visualize the bony covering of the superior semicircular canal. There are different reconstruction algorithms that also can be applied to highlight a particular tissue or organ. Bone disease is best visualized with a bone, edge, or detail algorithm to accentuate the interface between the bone and soft tissue. This can be particularly important in providing detailed bony anatomy needed for temporal bone, skull base, and sinus imaging. Any algorithm or reconstruction can be applied to the acquired CT data, which may be saved temporarily or permanently, depending on available storage space, for future use. There are recognized disadvantages to CT scanning, mainly due to its use of ionizing radiation and iodinated contrast administration. In the past decade, tremendous advances in CT technology and applications have increased its clinical utilization, creating concerns about individual and population doses of ionizing radiation. The most radiosensitive organs in the head are the lens of the eye and skin, with the greatest concern for early cataract formation and permanent skin damage, respectively. With assistance from the American College of Radiology, Radiologists and Diagnostic Imaging Physicists, scanning protocols are continually adjusted according to the ALARA (as low as reasonably achievable) principle to minimize patient radiation exposure while maintaining optimal image quality. Mechanisms used for dose reduction include adjusting x-ray beam filtration and collimation, x-ray tube current modulation and adaptation for a patient’s body habitus, peak kilovoltage optimization, improved detection system efficiency, and noise reduction algorithms. To further limit radiation exposure to the lens, CT scanning protocols should avoid including the orbits in the scanning field of view when appropriate. Other measures to limit total radiation exposure to patients have been employed in otolaryngologic imaging by using high-resolution helical scanning only in the axial plane, then performing reformations to avoid the additional radiation dose from direct coronal or sagittal imaging. This has been applicable particularly for imaging the petrous temporal bone, sinuses, and orbits. The additional patient risks from exposure to iodinated contrast are considered low. Overall, intravenous administration of iodinated contrast is considered safe, with severe or life-threatening reactions rare. Immediate adverse reactions are classified as idiosyncratic or nonidiosyncratic. Idiosyncratic reactions resemble allergic or hypersensitivity reactions that are unpredictable and independent of dose, categorized as mild, moderate, or severe. Severe reactions are potentially life-threatening, with development of hypotension, cardiac arrhythmias, bronchospasm, laryngeal edema, pulmonary edema, and respiratory arrest. Nonidiosyncratic reactions reflect the physiologic effects of contrast media and direct organ toxicity. These are predictable, dose-dependent reactions, including sensations of warmth, a metallic taste in the mouth, bradycardia, vasovagal reactions, and neuropathy. Known risk factors include previous reaction to iodinated contrast, multiple allergies, and asthma. Precautionary measures are recommended in patients identified at risk of contrast reactions with premedication using corticosteroids and antihistamines to reduce the risk of anaphylaxis. Delayed adverse reactions from iodinated contrast include contrast-induced nephropathy, estimated to occur in 2 to 7% of all patients receiving a CT scan with contrast. Generally, contrast-induced nephropathy manifests as an elevation in serum creatinine concentration within the first 24 hours that reaches a peak by 3 to 7 days, then returns to baseline within 1 to 2 weeks. In rare cases, patients require temporary or permanent dialysis. Patients with normal renal function are at very low risk for contrast-induced nephropathy. Known risk factors include preexisting renal insufficiency, diabetes mellitus, dehydration, concurrent use of nephrotoxic drugs, a high contrast dose, age > 70 years, and cardiovascular disease. Recommended precautions include adequate hydration, which is the most important method for preventing contrast-induced nephropathy. Administration of acetylcysteine (Mucomyst) and use of iso-osmolar contrast agents may also be done as precautionary measures. In summary, an overall risk–benefit assessment should be considered for patients prior to CT scanning. This is particularly recommended for the pediatric population, patients with identified risk factors, and patients requiring multiple or repeated CT examinations. Overall, CT scanning is the primary modality used in otolaryngologic imaging. It is preferred for imaging of the petrous temporal bone, sinuses, and orbit, given its superior spatial resolution for anatomical details and improved diagnostic imaging of bone structures compared with other modalities, such as MRI and plain films. Presently, CT scanning has replaced plain films for the detection of fractures of the orbit, petrous temporal bone, maxilla, face, and skull. CT imaging has a leading role in the evaluation of temporal bone disease for the external, middle, and inner ear. The high resolution of anatomical details, coupled with the ability to reformat the imaging plane into any obliquity, allows for optimal imaging of the temporal bone. For example, oblique planes can be reconstructed to obtain Poschl and Stenvers views to assess details of small structures, such as the superior semicircular canal and facial nerve canal, respectively. Another strength is the visualization and interpretation of temporal bone imaging in the challenging postoperative setting. Otologic procedures may extensively alter the appearance of the temporal bone with surgical removal of small middle ear structures or implantation of various prosthetic devices. The role of imaging with CT has been expanding in this area for accurately assessing the type and extent of the surgical changes and recognizing potential complications. Evaluation of the sinonasal cavity and the sinus drainage patterns is better with CT scanning techniques. The components of the ostiomeatal complex—the maxillary antrum, infundibulum, hiatus semilunaris, uncinate process, middle turbinate, and meatus—are well seen in the coronal plane. The sphenoethmoidal recesses are best evaluated on axial imaging. Processes that affect the nasal cavity and its turbinates are commonly viewed in both the axial and coronal planes. However, sagittal reformats provide improved visualization of the lateral nasal wall. CT and MRI have a complementary role in the evaluation of cranial nerve pathology and together offer a full picture of bone and soft tissue changes. CT can provide a road map of the skull base and facial bone anatomy preoperatively by depicting the neurovascular skull base foramina and canals. The effect of a lesion on the adjacent bone is useful in differentiating aggressive, rapidly growing processes from benign and indolent lesions. Malignant or aggressive processes may have a permeative or erosive appearance to the adjacent bone, whereas benign lesions may expand or remodel bone. Evaluation of the soft tissue neck is commonly performed by both CT and MRI modalities. CT provides improved detection of retained foreign bodies that are radiopaque and contain metallic elements. However, wood and glass fragments with lower lead levels may be difficult to visualize. Additional clinical indications for CT scanning are superior detection of a salivary gland calculus and calcifications on noncontrast imaging. Generally, CT and MRI are fairly comparable in the infrahyoid neck regarding the information they can provide. The fat content in the neck allows for increased conspicuity and delineation of pathology on CT. However, the ability of MRI to differentiate tumor–muscle interfaces, skull base and intracranial invasion, and perineural spread makes it a highly desirable imaging modality for otolaryngologic diseases. MRI is able to provide excellent anatomical detail in the evaluation of the neck without the use of ionizing radiation. The use of MRI in the evaluation of the neck has grown because of technological advances that allow for more rapid acquisitions and the ability of MRI to provide information on tissue characterization and pathologic information not available with other imaging modalities. MR image formation depends on the interaction of an applied magnetic field, radiofrequency (RF) waves, and the electromagnetic activity of atomic nuclei containing an odd number of protons and neutrons, both of which have spins. MR-active nuclei have a net spin, which induces a magnetic field as the nucleus spins around its axis. Hydrogen nuclei, or protons, are most commonly used in clinical MRI because of their abundance. An externally applied magnetic field causes the nuclei to spin at a given frequency, referred to as precessing. Also, the nuclei align parallel or antiparallel to the external magnetic field, creating a net magnetization vector. When an RF pulse is applied, the net magnetization vector is flipped to a certain angle, usually 90 degrees or less, producing two magnetization vector components, longitudinal (T1) and transverse (T2) magnetization. The transverse magnetization precessing around a receiver coil induces a current, which is the MR signal. When the RF pulse is turned off, the protons realign with the external magnetic field through the process of T1 relaxation, in which longitudinal magnetization recovers. In MR pulse sequences, there are two key parameters, the repetition time (TR) between the application of the RF excitation pulses and the echo time (TE) between the application of the RF pulse and the peak of the signal detected (spin echo).
Computed Tomography
Basic Principles
Advantages
Disadvantages
Clinical Applications
Magnetic Resonance Imaging
Basic Principles
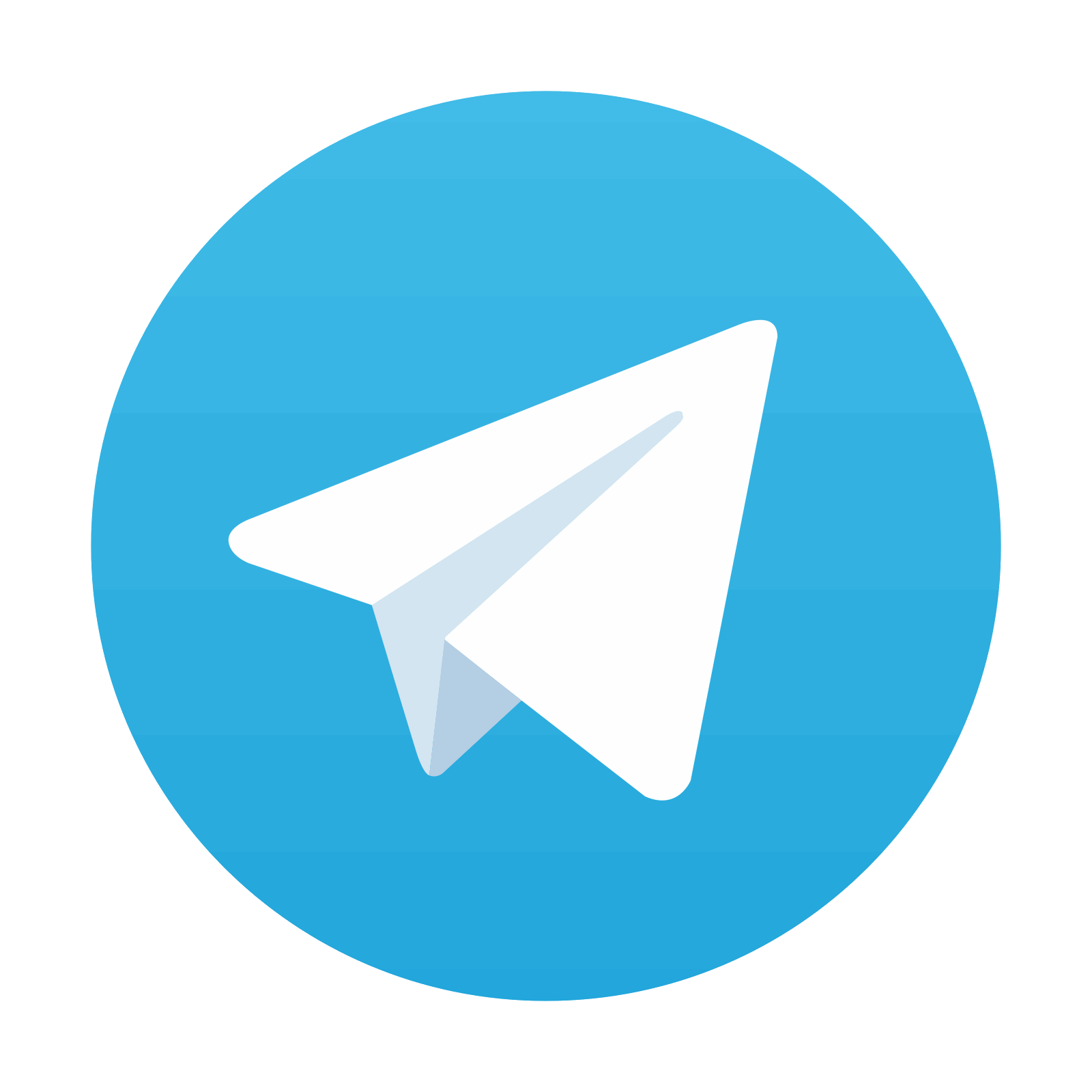
Stay updated, free articles. Join our Telegram channel

Full access? Get Clinical Tree
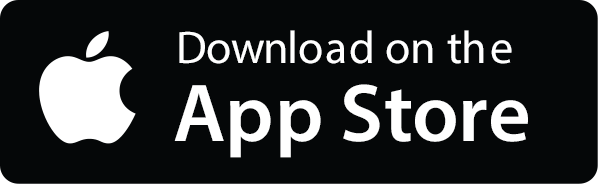
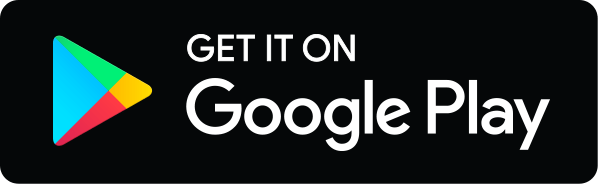