CHAPTER | 13 | The Retina |
Symptoms and Signs in Retinal Diseases
Evaluation of Retinal Diseases
Congenital and Developmental Anomalies
▃Anatomy of Retina
Retina is the innermost layer of eyeball. It extends from the margin of optic disc to ora serrata, which is 7 mm from the limbus.
■Microscopic Structure
Retina consists of 10 layers (Fig. 13.1). It comprises three layers of cells: photoreceptor cells, bipolar cells and ganglion cells. Layers of retina from out inwards are:
1.Retinal pigment epithelium (RPE): It is the single layer of hexagonal cells containing melanin granules which lies between the Bruch’s membrane of choroid and outer segment of photoreceptor cells (rods and cones).
2.Layer of rods and cones (photoreceptors): Rods and cones are the end organs of vision, which are nourished by choriocapillaris (Table 13.1). Rods and cones contain:
•Outer segment: It consists of membranous discs containing visual pigment.
•Inner segment: It is rich in mitochondria.
•Nuclear region: It forms outer nuclear layer.
•Synaptic terminal: It forms outer plexiform layer.
3.External limiting membrane (ELM): It is formed by glial tissue and perforated by rods and cones.
4.Outer nuclear layer (ONL): It is formed by nuclei of rods and cones.
5.Outer plexiform layer (OPL): It is formed by synapse between: rods and cones, bipolar cells and horizontal cells.
6.Inner nuclear layer (INL): It consists of cell bodies of bipolar cells, horizontal cells and amacrine cells. Bipolar cells constitute 1st order neurons. Horizontal cells connect one receptor cell to another. Amacrine cells synapse with bipolar cells and ganglion cells.
7.Inner Plexiform Layer (IPL): It is formed by synapses between axons of bipolar cells, dendrites of ganglion cells and processes of amacrine cells.
8.Ganglion cell layer (GCL): It contains cell bodies of ganglion cells. Ganglion cells are the 2nd order neurons of visual pathway.
9.Nerve fiber layer (NFL): It is formed by axons of ganglion cells which are nonmyelinated.
10.Internal limiting membrane (ILM): It separates retina from vitreous. It is the innermost layer of retina which is formed by glial tissue.
Table 13.1 Characteristic features of rods and cones | ||
Features | Rods | Cones |
Number | 120 million | 6 million |
Distribution | Mainly in peripheral retina | Occupy central retina |
Function | •Responsible for vision in dim light (scotopic vision). •Cannot detect color. •In periphery (extra foveal region) rods synapse with a bipolar cell. Hence, receptive field is more with less resolution. | •Responsible for vision in bright light (photopic vision). •Responsible for color vision. •At fovea there is1:1 correspondence between cones and bipolar cells. Hence, resolution is more and visual acuity is better. |
Loss of function | Leads to night blindness. | Leads to color blindness. |
Neurotransmitter | Glutamate | Glutamate |
The various layers of retina are bound together by neuroglia and vertical fibers of Müller, which have supportive as well as nutritive functions. To excite rods and cones, incident light has to traverse the tissues of retina. Information flows vertically from photoreceptors (rods and cones) to bipolar cells and then to ganglion cells, as well as laterally via horizontal cells in OPL and amacrine cells in IPL.
The retina comprises of RPE and neurosensory retina. The Bruch’s membrane separates RPE from choriocapillaris. RPE contain villous processes which reach out toward the outer segments of photoreceptors. The adhesion between RPE and neurosensory retina is weaker than that between RPE and the Bruch’s membrane. So, RPE can separate from neurosensory retina by fluid. This separation is called retinal detachment and the fluid in between the two layers is referred to as subretinal fluid (SRF).
■Division of Retina
Retina is divided into two parts by retinal equator which is considered to lie in the line with exit of four vortex veins. Retina posterior to retinal equator is called posterior pole which can be examined by direct ophthalmoscope and slit lamp with + 90D lens. Retina anterior to this equator is called peripheral retina which can be examined by indirect ophthalmoscope and slit lamp with three-mirror contact lens (Fig. 13.2).
Fig. 13.2 Division of retina.
Posterior pole of retina includes two distinct areas: optic disc and macula lutea.
Optic Disc
At optic disc, fibers of NFL pass through lamina cribrosa and run in the optic nerve. So, optic disc is also known as optic nerve head (ONH). All other layers of retina terminate at the optic disc, therefore the optic disc has no photoreceptors. The object is not seen if the image falls on optic disc, hence optic disc is a blind spot in the visual field. Its diameter is 1.5 mm = 1DD (1 disc diameter). A depression in the disc is called physiological cup. Retinal vessels emerge through this cup (Fig. 13.3a).
Fig. 13.3 (a) Posterior pole of retina. (b) Macula lutea.
Macula Lutea (Yellow Spot)
It is a circular area which appears darker than the surrounding retina and contains xanthophyll pigment. It is 5.5 mm in diameter. The center of macula is situated approximately 3 mm (2DD) away from the temporal margin of optic disc and approximately 1 mm below the horizontal meridian. Clinical landmarks within macula are as follows: fovea, foveola, and foveal avascular zone (FAZ) (Fig. 13.3b).
Fovea (fovea centralis) is a depression in inner retinal surface at the center of macula having a diameter of 1.5 mm (1 DD). Ophthalmoscopically, it is recognized by an oval light reflex. Parafoveal region (region around fovea) is the thickest part of retina containing several layers of ganglion cells.
Foveola is a small depression at the center of fovea and is the thinnest part of retina. It is 0.35 mm in diameter and is devoid of ganglion cells. It contains only cones. Other layers of retina are almost absent. This enables the most acute vision at foveola. Each cone relays to a single ganglion cell.
FAZ is an avascular area, that is, it does not contain any retinal blood vessel. It is 0.4 to 0.5 mm in diameter. Thus, it is located within fovea (1.5 mm) but extends beyond foveola (0.35 mm). Its exact limit can only be determined by fluorescein angiography (FA).
The eye is designed to focus the visual image on retina with minimal optical distortion. RPE cells contain melanin which absorbs any light not captured by the retina. It prevents light from being reflected off to the retina again.
In order to optimize light transmission to the foveolar cones, all retinal elements have to be displaced laterally out of the light path. Hence, light has a direct pathway to photoreceptors, and visual image received at foveola is the least distorted. Due to lateral displacement of retinal layers in the center of fovea, nerve fibers in outer plexiform (Henle’s) layer run almost parallel with the retinal surface before synapsing with the cells in INL (Fig. 13.4). Therefore, exudates within OPL (Henle’s layer) assume a star-shaped configuration (macular star), owing to this radial arrangement of fibers.
Fig. 13.4 Cross-section of fovea. Abbreviations: FAZ, foveal avascular zone; ILM, internal limiting membrane; RPE, retinal pigment epithelium.
■Blood Supply of Retina
Arterial Supply
Outer layers of retina up to ONL, that is, RPE, photoreceptors, ELM and ONL get their nutrition by diffusion from choriocapillaris.
Inner layers of retina are supplied by central retinal artery (CRA) and its branches. CRA is a branch of the ophthalmic artery.
Central retinal artery divides at or near the surface of the disc into two branches:
•Superior trunk: It divides into superior temporal (ST) and superior nasal (SN) branches.
•Inferior trunk: It divides into inferior temporal (IT) and inferior nasal (IN) branches (Fig. 13.5).
Fig. 13.5 Arterial supply of retina. Abbreviations: IN, inferonasal; IT, inferotemporal; SN, superonasal; ST, superotemporal.
Each of these branches divide dichotomously, spreading over retina and reaching the ora serrata. These are the end arteries, as they do not anastomose with each other. The only place where retinal system anastomoses with ciliary system is in the region of lamina cribrosa.
Choroidal vessels anastomose freely, whereas retinal vessels do not anastomose at all.
Macular region is supplied by branches from ST and IT arteries. Occasionally, cilioretinal artery from ciliary system enters the eye near the edge of disc and runs temporally toward macula.
Venous Drainage
The outer retinal layers are drained by vortex veins, while the inner layers of retina are drained through retinal veins.
The retinal veins do not accurately follow the course of arteries. At the disc, they join to form the central retinal vein (CRV) which follows the course of CRA. The CRA communicates with choroidal circulation in the prelaminar region.
Arteries are brighter red and narrower, while veins have purplish tint and are more tortuous.
In normal conditions, no pulsations can be seen in retinal arteries. In 80 to 90% of people, pulsations seen at the disc are venous due to the effect of intraocular pressure (IOP).
▃Symptoms and Signs in Retinal Diseases
Retinal affections may be congenital or developmental. Diseases of retina rarely occur in isolation. It is frequently affected with systemic diseases or involvement of adjacent structures (choroid, optic nerve, vitreous). The retina is richly supplied by blood vessels, therefore it is frequently involved in systemic vascular disorders. Retinal manifestation of a systemic vascular disorder is termed as retinopathy.
Retinal disorders may be classified into vascular, inflammatory, degenerative, neoplastic and detachment. Retinal affections, in general, give rise to the following symptoms and signs.
■Symptoms of Retinal Disorders
1.Diminution of vision.
2.Scotomas corresponding with the areas affected.
3.Metamorphopsia, that is, distortion of perceived images. It is a common symptom of macular disease and not present in optic neuropathy.
4.Micropsia, that is, decrease in image size. It is caused by the spreading apart of foveal cones.
5.Macropsia, that is, increase in image size. It is due to the crowding together of foveal cones.
6.Flashes of light in front of the eyes due to traction on retina.
7.Floaters (black spots in front of eyes).
8.Nyctalopia (night blindness). It is attributed to the interference with function of retinal rods. It may be congenital or found in retinitis pigmentosa and other tapetoretinal degenerations.
9.Hemeralopia (day blindness). It may be due to congenital deficiency of cones (rare).
10.Pain is invariably absent.
■Signs of Retinal Disorders
The retinal changes originate from retinal vascular changes affecting retinal arteries and arterioles, capillaries, veins and venules. In retinal capillaries, endothelium lacks fenestrations and forms inner blood–retinal barrier. Outside endothelium is a thick basement membrane. Within this membrane are the intramural pericytes (Fig. 13.6).
Fig. 13.6 (a) Capillary wall in retina; (b) Retinal capillaries and choriocapillaris. Abbreviations: GCL, ganglion cell layer; ILM, internal limiting membrane; INL, inner nuclear layer; IPI, inner plexiform layer; NFL, nerve fiber layer; ONL, outer nuclear layer; OPL, outer plexiform layer; RPE, retinal pigment epithelium.
Capillaries are distributed within inner layers of retina including INL. Outer layers have no blood vessels which are nourished by choriocapillaris.
Various signs in retinal diseases are (Flowchart 13.1):
Flowchart 13.1 Pathogenesis of retinal affections.
•Microaneurysms.
•Retinal hemorrhages.
•Exudates.
•Cotton-wool spots.
•Arteriovenous (A–V) shunts.
•Neovascularization.
•Changes in retinal vessels.
Microaneurysms
These appear as small, round, red dots and located in the INL of retina. Location of microaneurysms indicates areas of capillary nonperfusion. These may become thrombosed or may leak, resulting in formation of hard exudates, frequently arranged in rings. So, the center of ring of hard exudates usually contain microaneurysms.
Retinal Hemorrhages
These may be preretinal hemorrhages (subhyaloid hemorrhage) or intraretinal hemorrhages (hemorrhage within retinal tissue). Intraretinal hemorrhages could be further divided into superficial and deep hemorrhages.
Preretinal hemorrhage lies in the potential space between retina and vitreous. It never coagulates. Initially, preretinal hemorrhage is round and becomes hemispherical with straight upper border (boat-shaped hemorrhage), as red blood cells (RBCs) settle down inferiorly. The lower cellular portion of hemorrhage is darker than the serum-containing upper portion.
Superficial hemorrhages are located in the NFL, so they have a feathery or flame-shaped appearance, corresponding to NFL. These arise from large, superficial precapillary arterioles.
Deep hemorrhages are located in the compact deeper layer of retina. So, they have a “dot and blot” appearance. These arise from the venous end of capillaries in deeper layers (Fig. 13.7).
Fig. 13.7 Dot and blot hemorrhages. Source: Diabetic retinopathy. In: Tabandeh H, Goldberg M, eds. The Retina in Systemic Disease: A Color Manual of Ophthalmoscopy. 1st ed. Thieme; 2009.
Retinal Edema
Retinal capillaries are located in the INL and inner layers of retina, therefore edema is located between the OPL and INL of retina initially, and later it may also involve IPL and NFL. Retinal edema may be diffuse or localized. A localized edema in the macular region assumes a star-shaped configuration, owing to the formation of radiating folds. This configuration corresponds to the radial arrangement of fibers in OPL (Henle’s layer).
Hard Exudates
These are waxy, yellow lesions with relatively distinct margins. These are located mainly within the OPL (Henle’s layer). They develop at the junction of normal and edematous retina. These are composed of lipoprotein and lipid-filled macrophages. Lipoproteins are thought to leak from microaneurysms. Exudates within Henle’s fiber layer (OPL) in the macular region typically assume a star-shaped configuration, corresponding to the radial arrangement of fibers (macular star) (Fig. 13.8). When leakage ceases, phagocytosis of their lipid content results in spontaneous absorption of exudates but exudates enlarge if the leakage is chronic.
Fig. 13.8 Hard exudates.
Cotton-Wool Spots
These are composed of accumulations of neuronal debris within the NFL as a result of retinal ischemia. So, cotton-wool spots are fluffy, gray–white, and superficial (in NFL) with ill-defined margins (Fig. 13.9). Being superficial, they obscure underlying blood vessels. They disappear rapidly, as debris is removed by autolysis and phagocytosis. Retinal hypoxia leads to formation of A–V shunts and neovascularization in an attempt to re-establish blood supply.
Fig. 13.9 Cotton-wool spots.
Arteriolar–Venular Shunts/Intraretinal Microvascular Abnormalities (IRMA)
These are the shunt vessels running from the arterioles to venules (bye passing the capillary bed) formed in an attempt to re-establish blood supply. A–V shunts do not leak on FA.
Neovascularization (Formation of New Vessels)
Hypoxic retinal tissue elaborates vasoproliferative substance (such as vascular endothelial growth factor [VEGF]) in an attempt to re-establish blood supply, which results in the formation of new vessels. The new vessels leak protein and bleeds easily. VEGF can promote neovascularization on:
•Disc: Neovascularization at disc (NVD).
•Retina: Neovascularization elsewhere away from disc (NVE).
•Iris: Rubeosis iridis (Fig. 13.10).
Fig. 13.10 Formation of new vessels in response to hypoxic retina.
Changes in Retinal Vessels
Ratio of retinal vessels caliber (artery: vein) is 2:3. Veins are crossed by arteries. The changes in retinal vessels in various retinal diseases are venous tortuosity, venous dilatation, arteriolar narrowing and changes at A–V crossings. These changes at AV crossings are commonly associated with arteriosclerosis and include:
•Nipping and tapering of veins on both sides of crossings is known as Gunn’s sign.
•Banking of veins distal to A–V crossings is known as Bonnet’s sign.
•Right-angled deflection of veins at A–V crossings is known as Salus’ sign.
▃Evaluation of Retinal Diseases
•Retinal diseases can be evaluated by the following investigations:
•Electrophysiological tests: These include electroretinography (ERG), electro-oculography (EOG) and visually evoked potential (VEP).
•Fundus angiography: These include FA and indocyanine green angiography (ICGA).
•Optical coherence tomography (OCT).
•Ocular ultrasonography.
■Electrophysiological Tests
The objective recordings of visual functions can be achieved by certain tests which are known as electrophysiological tests. These tests include ERG, EOG and VEP.
Electroretinography (ERG)
Normal ERG is biphasic and consists of:
•α-Wave: It is a negative wave and arises from photoreceptors.
•β-Wave: It is a positive deflection and represents electrical activity in bipolar cells (although generated by Müller cells) (Fig. 13.11).
Fig. 13.11 Electroretinography. Abbreviation: RPE, retinal pigment epithelium.
Significance
ERG is useful in detecting functional abnormalities of outer retina up to the bipolar cell layer. ERG gives the indication of activity of rods and cones. ERG is normal in diseases involving ganglion cells and NFL. Focal ERG by using sharply focused light can provide information about macular function. It can assess retinal function in the presence of dense cataract.
Electro-Oculography (EOG)
EOG measures the resting potential between the electrically positive cornea and electrically negative back of eye (retina) with horizontal eye movements. It reflects activity of RPE and photoreceptors, so lesions proximal to photoreceptors will have a normal EOG.
Significance
As EOG is based on the activity of RPE and photoreceptors, it is subnormal or flat in case of retinitis pigmentosa, degenerative myopia, macular dystrophies and vitamin A deficiency.
Visually Evoked Potential (VEP)
It is also called VER (visually evoked response). VEP is the recording of electrical activity of visual cortex, which is generated by stimulation of retina. VEP is the only objective test to assess functional status of visual system beyond retinal ganglion cells. So, abnormal VEP with normal ERG and EOG suggests an organic lesion in the pathway between and including GCL and visual cortex.
■Fundus Angiography
Fundus angiography may be FA or ICGA. The former is excellent for demonstration of retinal circulation against the dark background of RPE. It is not helpful in delineating the choroidal circulation. To study the choroidal circulation, ICGA is of particular value as it provides better resolution of the choroidal vasculature. ICGA is a useful adjunct to FA.
Fluorescein Angiography
Features of Dye Used in Fluorescein Angiography
Dye used: Fluorescein dye.
Excitation peak of dye—490 nm (blue part of spectrum).
Emission peak of dye: 530 nm (green part of spectrum).
Dose: 5 mL of 10% OR 3 mL of 25% aqueous solution of sodium fluorescein.
Adverse effects: Allergy to Fluorescein results in laryngeal edema, bronchospasm and anaphylactic shock.
Contraindications:
•Allergy to Fluorescein.
•Diabetic nephropathy.
•Chronic renal failure.
•Opaque media in the eye, preventing proper evaluation.
Dye in Circulation
A significant percentage (70–80%) of Fluorescein bind to serum albumin (bound Fluorescein) and the rest remain unbound (free Fluorescein). Major choroidal vessels are impermeable to both bound and free Fluorescein. Choriocapillaris contain fenestrations, so free Fluorescein escape into extravascular space and pass across the Bruch’s membrane but tight junctions (zonula occludens) between RPE cells prevent passage of free Fluorescein molecules across RPE and maintain outer blood–retinal barrier. Passage of Fluorescein across RPE is, therefore, abnormal. Tight junctions between endothelial cells in retinal capillaries form inner blood–retinal barrier.
It prevents leakage of dye, and Fluorescein is confined within the lumen of retinal capillaries. Any leakage from retinal circulation is, therefore, pathological (Fig. 13.12).
Fig. 13.12 The outer blood–retinal barrier. Abbreviation: RPE, retinal pigment epithelium.
Thus, FA is an excellent method for studying retinal circulation. It is not helpful in delineating choroidal circulation, as Fluorescein leaks from choriocapillaris, producing choroidal fluorescence.
Technique of Fluorescein Angiography (FA)
Dilate the pupil and inject fluorescein dye into the antecubital vein of the patient. Arm-to-retina circulation time is about 10 second, so serial photographs are taken by fundus camera 8 to 10 seconds after commencement of dye injection. Late-phase photographs yield the most useful information in ICGA because the dye remains in neovascular tissue after leaving the retinal and choroidal circulations. Nornal angiogram consists of four overlapping phases.
A cilioretinal artery, if present, fills during the choroidal phase because it is derived from posterior ciliary circulation.
Normal Angiogram
Normal angiogram consists of four overlapping phases.
1.Prearterial/choroidal phase is characterized by filling of choroidal circulation.
2.Arterial phase shows appearance of dye in retinal arterioles.
3.A–V/capillary phase is characterized by complete filling of arterioles, filling of capillaries, and laminar flow in retinal veins.
4.Venous phase shows venous filling with arteriolar emptying (Flowchart 13.2).
Flowchart 13.2 Entry of fluorescein into the choroidal and retinal circulations.
Dye is absent from angiogram after 5 to 10 minutes. The late phase demonstrates late staining of disc.
Appearance of fovea on FA: Fovea appears dark on FA due to:
•Absence of blood vessels in FAZ.
•Increased density of xanthophyll pigment at fovea.
•Heavily pigmented RPE cells with melanin at fovea.
Abnormal Angiogram
It is based on blood–retinal barriers. Outer blood–retinal barrier at the level of choroidal circulation (RPE barrier) provides information regarding integrity of RPE. Inner blood–retinal barrier at the level of retinal circulation provides information regarding integrity of retinal vessels. Abnormal fluorescence may be:
•Hyperfluorescence (increased fluorescence).
•Hypofluorescence (decreased or absent fluorescence).
Causes of hyperfluorescence are as follows:
1.Increased transmission or RPE window defect (Fig. 13.13a): It is caused by atrophy of RPE as in atrophic age-related macular degeneration (ARMD) which results in unmasking of normal background choroidal fluorescence.
2.Breakdown of outer blood–retinal barrier (breakdown of RPE tight junctions): It results in pooling of dye in subretinal space as in central serous retinopathy (CSR) (Fig. 13.13b).
3.Breakdown of inner blood–retinal barrier as in cystoid macular edema (CME).
4.Leakage from abnormal vessels: Leakage of dye may occur from abnormal choroidal vessels such as choroidal neovascularization (CNV) or retinal vessels as neovascularization in proliferative diabetic retinopathy (PDR).
5.Staining of tissues: Staining due to prolonged retention of dye as in drusen or fibrous tissue.
Fig. 13.13 (a) RPE “window” defect as in atrophic ARMD. (b) Pooling of dye in subretinal space as in CSR. Abbreviations: ARMD, age-related macular degeneration; CSR, central serous retinopathy; RPE, retinal pigment epithelium.
Hypofluorescence could be due to blockage of normal fluorescence (blocked fluorescence, Fig. 13.14) or inadequate perfusion of tissues (filling defects) (Table 13.2).
Fig. 13.14 Lesions causing blocked fluorescence.
Table 13.2 Causes of hypofluorescence | |
Blocked fluorescence | Filling defects |
Blockage of background choroidal fluorescence only: It may be seen in subretinal or sub-RPE lesions such as blood and congenital hypertrophy of RPE. | Vascular occlusion involving choroidal or retinal circulation. |
Blockage of retinal as well as choroidal fluorescence: It may be seen in preretinal lesions such as subhyaloid hemorrhage and hard exudates in neurosensory retina. | Loss of vascular tissue as in choroideremia, myopic degeneration. |
Abbreviation: RPE, retinal pigment epithelium. |
Indocyanine Green Angiography
Features of Dye Used in Indocyanine Green Angiography (ICGA)
Dye used: ICG dye.
Excitation peak of dye: 805 nm (infrared [IR] spectrum).
Emission peak of dye: 835 nm (IR spectrum).
Dose: 25 to 40 mg ICG powder is dissolved in 2 mL of aqueous solvent (40 mg in 2 mL).
Adverse effects: These are less common than Fluorescein.
Contraindications: Because dye contains 5% iodine, it should not be given to the patients allergic to any iodine compound and seafood.
Dye in Circulation
A major percentage (98%) of ICG molecules bind with serum albumin. So, dye remains within choriocapillaris, as fenestrations of choriocapillaris are impermeable to albumin. Thus, ICGA provides better resolution of choroidal vasculature, and hence it is used for studying the choroidal lesions.
Causes of abnormal fluorescence in ICGA (hyper or hypofluorescence) are identical to FA, except in RPE detachment (pigment epithelial detachment). RPE detachment shows hyperfluorescence on FA, but hypofluorescence on ICGA.
■Optical Coherence Tomography (OCT)
OCT is a noninvasive, noncontact imaging system which provides cross-sectional images of retina, optic nerve head and anterior segment with a high-resolution. OCT is an optical analog of B-scan ultrasound, where instead of sound, IR light is used to image the layers of retina. The coherent light penetrates ocular tissues; reflected images are analyzed by a camera based on reflectivity of tissues, and a color code is assigned for interpretation.
It is based on the principle of Michelson low-coherence interferometry. In the retina, OCT is used to diagnose macular pathology such as:
•Macular hole.
•Macular edema.
•Pigment epithelial detachment (PED).
•Epiretinal membrane (ERM).
•ARMD.
OCT should be avoided in media opacities such as:
•Moderate to dense cataract.
•Vitreous hemorrhage.
•Posterior capsule opacification.
■Ultrasonography
Diagnostic ultrasound is used in cases of opacification of ocular media. Ultrasonography uses high-frequency sound waves (10 MHz). The sound waves produce echoes on striking the interfaces between acoustically different structures. Different pulse echo techniques are A-scan and B-scan. The sound is coupled to the eye by means of a saline bath or directly through a transducer.
A-Scan
The sound is transmitted from the transducer into eye and echoes are received by the same transducer, which are recorded as spikes. It produces a unidimensional display of vertical spikes along a base line. The height of spikes is proportional to the strength of the echo, and the position of spike indicates the time of receiving the echo. The linear distance between individual spikes is used to measure the anterior chamber depth, lens thickness and axial length. A-scans are used for biometry in intraocular lens (IOL) calculations.
B-Scan
In B-scan, the echoes are plotted as dots instead of spikes. The more sound reflected, the brighter the dot. It provides a two-dimensional (2D) ultrasonography and delineates intraocular structures in eyes with opaque media, intraocular and orbital mass lesions. The frequency of the transducer determines which part of the globe or orbit is examined. Low-frequency transducers are useful in detecting orbital pathology, while the moderate frequency transducers (7–10 MHz) are used to examine the globe for RD, posterior intraocular tumors and detection of calcification in retinoblastoma and optic disc drusen. The high-frequency transducer (30–50 MHz) images the anterior segment but only to a depth of 5 mm. Dynamic ultrasound scanning is performed by moving the eye but not the probe and allows differentiation of RD and vitreous detachment. The addition of a color Doppler’s facility allows the evaluation of blood flow.
▃Retinal Photocoagulation (OP8.2)
Photocoagulation is essentially a destructive form of therapy (Fig. 13.15). It depends upon absorption of laser light energy by ocular pigments. Ocular pigments are:
Fig. 13.15 Retinal photocoagulation laser spot.
1.Melanin (present in cells of RPE and choroid).
2.Hemoglobin (present in blood).
3.Xanthophyll (yellow pigment present in inner retinal layers of macula).
The absorbed light is converted into heat energy which is utilized for coagulation of new blood vessels, chorioretinal adhesion and destruction of RPE.
Laser (LASER = Light Amplification by Stimulated Emission of Radiation) can be delivered to the eye through:
•Slit lamp.
•Indirect ophthalmoscope.
•Fiber optic probe during pars plana vitrectomy (i.e., endophotocoagulation).
■Laser Used for Photocoagulation
Main photocoagulators are:
1.Argon green laser.
2.Krypton laser.
3.Diode laser—is also used for photodynamic therapy (PDT).
4.Frequency-doubled Nd: YAG laser (Nd: YAG = Neodymium: Yttrium-aluminum-garnet).
■Principles of Retinal Photocoagulation
The light energy emitted by argon laser is absorbed by all three ocular pigments.
The light energy emitted by krypton laser is absorbed well by melanin but poorly or not at all by hemoglobin and xanthophyll. So, the main effect of krypton laser is on choroid and RPE containing melanin.
•Photocoagulation of lesions inside FAZ: Xanthophyll in macular area absorbs argon laser light energy and becomes a heat source. So, when argon laser is applied close to fovea (inside FAZ), it becomes dangerous. The main advantage of krypton over argon is that it is not absorbed by xanthophyll pigment.
•Because krypton is not absorbed by hemoglobin, it may be possible to treat retina through vitreous hemorrhage with krypton but not with argon.
•Treatment in presence of nuclear sclerosis: As krypton is not absorbed by xanthophyll pigment in the lens, so krypton is more effectively transmitted through nuclear sclerosis than argon.
•Smaller the spot size, the greater is the energy. So, when changing to a smaller spot size during photocoagulation, the power level must be turned down and vice versa.
■Indications of Photocoagulation
•Diabetic retinopathy (DR).
•Other causes of retinal neovascularization.
•Sealing of retinal breaks.
•Macular disorders.
■Complications of Laser Photocoagulation
Complications of posterior segment include foveal burn, macular pucker, retinal and choroidal hemorrhages. Complications of anterior segment include:
•Cornea: Burn, erosion, and superficial punctate keratopathy.
•Iris: Absorption of heat by posterior pigment epithelium of iris increases the temperature of aqueous which results in damage to the corneal endothelium.
•Lens: Lens opacities (due to energy absorbed by posterior pigment epithelium of iris).
▃Congenital and Developmental Anomalies
■Coloboma of Retina
A typical coloboma is inferonasal, as embryonic fissure is inferior and slightly nasal (Fig. 13.16). The coloboma found in other direction is called atypical. Characteristic features of coloboma of retina are:
Fig. 13.16 Coloboma of the retina and choroid. Source: Coloboma. In: Lang G, ed. Ophthalmology. A Pocket Textbook Atlas. 3rd ed. Thieme; 2015.
•Fundus examination: Coloboma appears as an oval, white depressed defect with a rounded apex toward the disc. Few vessels are seen over the surface.
•Visual field: There is scotoma in the field corresponding to coloboma.
•Complications: There is high risk of RD.
•Prophylaxis: Prophylactic laser delimitation along the edges of coloboma may be done.
■Myelinated Nerve Fibers (Medullated Nerve Fibers)
Myelination of optic nerve progresses from the brain toward periphery. In normal eyes, myelination of optic nerve stops at lamina cribrosa. It may continue beyond the optic disc to involve NFL and appear as opaque nerve fibers (myelinated nerve fibers of retina, Fig. 13.17). Myelination is usually completed shortly after birth. So, strictly speaking medullated nerve fibers (MNFs) are not congenital. Characteristic features of MNF are:
Fig. 13.17 Myelinated nerve fibers. Source: Differential diagnosis of disc edema. In: Biousse V, Newman N, ed. Neuro-Ophthalmology Illustrated. 3rd ed. Thieme; 2019.
•Fundus examination: They appears as white patches with feathery margins covering the retinal vessels. Usually, they are contiguous with disc, and occasionally they are isolated. They are mistaken for cotton- wool spots.
•Visual field shows enlargement of blind spot when contiguous with disc or scotoma, corresponding to the position of patch when they are isolated.
Medullary sheath disappears if glaucoma or optic atrophy causes the fibers to degenerate, or in case demyelinating disorder such as multiple sclerosis is present.
▃Vascular Disorders of Retina (OP8.1, OP8.2, OP8.4)
Vascular disorders of retina arise due to:
•Obstruction of arterial circulation: It may be central retinal artery occlusion (CRAO) or branch retinal artery occlusion (BRAO).
•Obstruction of venous circulation: It may be central retinal vein occlusion (CRVO) or branch retinal vein occlusion (BRVO).
•Retinal telangiectasias.
■Retinal Artery Occlusion (OP8.1, OP8.2, OP8.4)
Obstruction may affect central retinal artery itself (CRAO) or a peripheral branch (BRAO). Central retinal artery is occluded almost always at lamina cribrosa where vessels normally become slightly narrow. In CRAO, entire retina is involved, but in BRAO, only the area supplied by the branch is affected.
Etiology
Obstruction of a retinal artery is due to atheroma, arteritis, embolus from heart, embolus from carotid arteries and raised IOP.
1.Obliteration of artery due to atheroma (atherosclerosis): Thrombosis at the level of lamina cribrosa is the most common cause of CRAO. The risk factors for atherosclerosis include age, hypertension, smoking, diabetes, obesity, and raised low density lipoprotein–cholesterol (LDL– cholesterol) in serum.
2.Obliteration of artery due to arteritis: Disorders that may give rise to retinal arteritis include giant cell arteritis, systemic lupus erythematosus (SLE), and polyarteritis nodosa.
3.Obliteration due to embolus from heart (cardiac embolism): It results from cardiac valvular diseases comprising calcium (calcific emboli) or septic emboli from cardiac valves in bacterial endocarditis.
4.Obliteration due to embolus from carotid arteries (carotid embolism): Embolus arises from an atheromatous plaque at the bifurcation of common carotid artery. Emboli may be cholesterol emboli (Hollenhorst plaques) containing cholesterol, or fibrinoplatelet emboli consisting of platelet and fibrin. Since ophthalmic artery is the first branch of internal carotid artery (ICA), embolus from heart or carotid arteries has a fair chance to occlude retinal artery.
5.Obliteration due to raised IOP: It is seen in acute angle closure glaucoma and excessive pressure on globe during RD surgery or neurosurgical procedures.
Clinical Features
Clinical features in arterial occlusion will depend on the size and location of obstructed vessel.
Central Retinal Artery Occlusion (CRAO)
In CRAO, there is sudden, painless loss of vision. Transient loss of vision (amaurosis fugax) due to occlusion by minute emboli may be the premonitory symptom.
If macula and papillomacular bundle is supplied by a cilioretinal artery, arising from posterior ciliary circulation, central vision may be preserved. The remainder of the field of vision is lost.
Clinical signs of CRAO are:
•Pupil: There is no direct pupillary light reaction, that is, relative afferent pupillary defect (RAPD) is present.
•Fundus reveals the following findings:
1.Retinal arteries appear thread-like but no change in caliber of retinal veins.
2.Retina becomes milky white and edematous.
3.Cherry red spot at fovea, since the vascular choroid is visible underneath in contrast to the surrounding cloudy-white retina (Fig. 13.18 and Flowchart 13.3).
4.If occlusion is incomplete, a gentle pressure upon globe may break up the blood column in retinal veins into red beads (segmentation of blood column), moving in a jerky way. The beads of blood column in the veins move sometimes in normal direction of blood flow and sometimes in opposite direction–“cattle-truck” appearance.
Fig. 13.18 Cherry red spot in CRAO. Abbreviation: CRAO, central retinal artery occlusion.
Flowchart 13.3 Signs in CRAO. Abbreviations: CRAO, central retinal artery occlusion; GCL, ganglion cell layer; NFL, nerve fiber layer.
Cherry red spot is seen in: CRAO
•Trauma (Berlin’s edema).
•Niemann-Pick disease.
•Gaucher’s disease.
•Tay-Sach’s disease.
•Sandoff’s disease.
Mnemonic–Cherry Tree Never Grow Tall in Sand
After several weeks, retinal cloudiness and cherry red spot gradually disappear as retinal edema subsides. Retina regains its transparency but its inner layers are completely atrophic. However, retina appears nearly normal owing to the viability of its outer layers which receive their nourishment from the choroid. Optic disc becomes atrophic because of the atrophy of NFL, so there is no direct pupillary reaction to light.
Branch Retinal Artery Occlusion (BRAO)
BRAO causes ischemia with edema in the area supplied by the obstructed artery. The ischemic retina appears white, with narrowing of arterioles. The atrophy of inner layers of involved retina causes permanent sectorial visual field defect.
Investigations
The following investigations are helpful to determine arterial occlusion:
•Carotid artery imaging by color Doppler ultrasonography.
•Serum lipid profile.
•Echocardiogram: To evaluate cardiac valves.
•Full blood count and erythrocyte sedimentation rate (ESR).
Treatment
Treatment is very unsatisfactory but attempts should be made to restore retinal circulation by dislodging emboli. Calcific emboli are much more dangerous than cholesterol and fibrin–platelet emboli. Retinal tissue cannot survive ischemia for than a few hours. So, the following treatment may be tried if patient reports early:
•Massaging the globe intermittently for at least 15 minutes.
•Anterior chamber paracentesis.
•IV acetazolamide (500 mg) to lower IOP.
•Inhalation of amyl nitrite produces vasodilatation.
•Systemic steroids in high dosage if giant cell arteritis is the underlying cause.
To confirm giant cell arteritis, ESR is measured, and biopsy of temporal artery is performed.
Complications
Some eyes with CRAO develop rubeosis iridis and neovascular glaucoma (NVG).
■Retinal Vein Occlusion (OP8.1, OP8.2, OP8.4)
Both arterial and venous diseases contribute to retinal vein occlusion.
Predisposing Factors
Predisposing factors for retinal vein occlusion are arteriosclerosis, hypertension, hyperviscosity of blood (as a result of hypercellularity in polycythemia vera or leukemia or as a result of changes in plasma proteins in macroglobulinemia or myeloma), hyperlipidemia as in hypothyroidism, occlusive periphlebitis retinae as in sarcoidosis and Behcet’s disease, raised IOP as in primary open-angle glaucoma, and diabetes mellitus. There are some miscellaneous causes too, for example, oral contraceptives, secondary hypertension (e.g., Cushing syndrome) and smoking.
An artery (or arteriole) and a vein share a common adventitial sheath at A–V crossings in retina and just behind lamina cribrosa. Thickening of an artery (or arteriole) compresses the vein, resulting in retinal vein occlusion. Therefore, arteriosclerosis is an important causative factor for retinal vein occlusion.
At A–V crossing posterior to lamina cribrosa, central retinal vein is compressed, resulting in CRVO. At A–V crossings in retina, arteriosclerosis of retinal arteriole compresses the venule and results in BRVO.
Thus, retinal vein occlusion may be CRVO or BRVO.
Central Retinal Vein Occlusion (CRVO)
According to clinical features and prognosis, CRVO may be divided into nonischemic and ischemic forms. In nonischemic CRVO (venous stasis retinopathy) or partial CRVO, there is venous stasis with delayed venous drainage, so there is no retinal ischemia. Complete CRVO is accompanied with retinal ischemia, hence the name ischemic CRVO (Table 13.3).
Table 13.3 Differentiating features between nonischemic and ischemic CRVO | ||
Nonischemic CRVO | Ischemic CRVO | |
1. Visual impairment | ++ | ++++ |
2. Afferent pupillary conduction defect | ± | ++ |
3. Vitreous hemorrhage | Never | Frequent |
4. Prognosis | Reasonably good | Extremely poor |
Abbreviation: CRVO, central retinal vein occlusion. |
Nonischemic CRVO
It is the most common type and occurs in young persons. The cause is unknown. It probably results from phlebitis affecting central retinal vein within ONH. Patient complains of unilateral, mild-to-moderate fogginess of vision.
Fundus Examination
During early stages, venous stasis causes nonischemic CRVO, disc edema, engorged, tortuous and dilated veins and retinal hemorrhages (superficial and deep) more in peripheral retina than at posterior pole (Fig. 13.19).
Fig. 13.19 Nonischemic CRVO. Abbreviation: CRVO, central retinal vein occlusion.
In late stages when venous stasis is chronic (6–12 months), fundus reveals cotton-wool spots and chronic CME. Vitreous hemorrhage is never present.
Fluorescein Angiography (FA)
FA shows venous stasis with delayed A–V transit time (i.e., delayed venous drainage) and good retinal capillary perfusion.
Treatment
Systemic corticosteroids are withheld unless there is macular edema. Intravitreal triamcinolone is given for chronic macular edema.
Prognosis
It is reasonably good. Visual acuity returns to normal or near normal. Main cause for poor vision is chronic CME.
Ischemic CRVO
It usually occurs in elderly people with cardiovascular disorders. It is characterized by sudden complete occlusion of central retinal vein just behind lamina cribrosa due to thrombus formation. Patient complains of sudden and severe, painless visual impairment (it is not as sudden as found in CRAO). There is relative afferent pupillary conduction defect in affected eye which is marked.
Fundus Examination
In early stage, fundus reveals the following:
•Markedly engorged and tortuous retinal veins.
•Disc edema and hyperemia.
•Retinal hemorrhages. These are so extensive that entire retina is covered with them (splashed tomato appearance).
•Cotton-wool spots.
•Retinal and macular edema (Fig. 13.20).
•In late stage: Marked NVE and NVD are seen (Flowchart 13.4).
•Sheathing around veins and collateral around disc are found.
Fig. 13.20 Ischemic CRVO. Abbreviation: CRVO, central retinal vein occlusion.
Flowchart 13.4 Pathogenesis of ischemic CRVO. Abbreviation: CRVO, central retinal vein occlusion.
Fluorescein Angiography (FA)
No useful information can be obtained because of marked retinal hemorrhages. In less severe cases, there are extensive areas of capillary nonperfusion.
Clinical Course of Disease
Acute signs resolve after 6 to 12 months. Retina undergoes atrophy and pigmentary changes. Macular degeneration (cystoid degeneration) occurs. Neovascularization may be seen at disc (NVD) or elsewhere in retina (NVE). Cilioretinal collaterals at disc can be seen.
Treatment
No treatment is effective. Laser pan retinal photocoagulation (laser PRP) should be performed to prevent development of NVG. If media is hazy, anterior retinal cryopexy may prevent NVG.
Prognosis
It is extremely poor.
Complications
Complicated CRVO results in optic atrophy, recurrent vitreous hemorrhage and NVG. Vitreous hemorrhage occurs due to neovascularization of retina. NVG occurs following rubeosis iridis and neovascularization at the angle of anterior chamber. It appears in 50% cases within 3 months of occlusion, so it is also known as 90-day glaucoma.
Branch Retinal Vein Occlusion (BRVO)
BRVO is common in superotemporal branch due to numerous crossings by artery.
Clinical features
Symptoms depend on the location of occlusion. Vision is affected only when macula is involved and is characterized by sudden blurring of vision and metamorphopsia.
BRVO is characterized by the following clinical signs:
•Dilated and tortuous veins distal to the site of occlusion and attenuation proximally (Fig. 13.21a).
•Retinal hemorrhages–superficial, dot and blot are confined to the area drained by the vein (Fig. 13.21b).
•Retinal edema is also confined to the area drained by the vein.
•Cotton-wool spots.
•Relative visual field defect: Visual defect is partial but not exactly sectorial, as in the case of BRAO.
Fig. 13.21 (a) Different sites of vein occlusion. (b) BRVO (inferotemporal). Abbreviation: BRVO, branch retinal vein occlusion.
Blockage of superior temporal (ST) vein frequently involves macula leading to visual impairment.
Prognosis
It depends on the integrity of perifoveal capillary network. It is good if perifoveal capillary network is intact or complete and prognosis is poor if it is incomplete or broken.
Complications
Two main vision threatening complications are:
•CME.
•Neovascularization: NVD and NVE.
Neovascularization may cause recurrent vitreous hemorrhage.
Treatment
At least 3 months’ time should be given for spontaneous resolution of edema and hemorrhages. If there is good macular perfusion and visual acuity is improving, no treatment is required. If perifoveal capillary network is broken on FA, it is unlikely to benefit from laser therapy.
For macular edema, grid laser photocoagulation and intravitreal triamcinolone acetonide are helpful.
For neovascularization, scatter laser photocoagulation is done to prevent vitreous hemorrhage.
■Primary Retinal Telangiectasia
It comprises a group of rare, idiopathic, and congenital retinal vascular anomalies characterized by:
•Dilatation and tortuosity of retinal vessels.
•Formation of multiple aneurysms.
•Vascular leakage.
•Deposition of hard (lipid) exudates.
Retinal telangiectasias always involve capillary bed, although arterioles and venules may also be affected. These may become symptomatic later in life. Primary retinal telangiectasia includes Coats’ Disease and Leber’s miliary aneurysm. Leber’s miliary aneurysm is a localized and less severe form of Coat’s disease in adults.
Because the condition is not associated with any other systemic or ocular disease, it should be distinguished from secondary telangiectasias, which are associated with other retinal vascular disorders such as retinal vein occlusion, DR, retinopathy of prematurity and sickle-cell retinopathy.
Coats’ Disease (Exudative Retinopathy of Coats)
It is the severe form of retinal telangiectasia. It is a rare, idiopathic, nonhereditary, uniocular condition. It typically presents in the first decade of life. It is more common in boys (75%). It usually presents as unilateral visual loss, strabismus and leukocoria (white pupillary reflex, leuko = white, coria = pupil). It is characterized by telangiectatic blood vessels, that is:
•Dilated and tortuous retinal blood vessels at the posterior pole temporal to fovea and at the retinal periphery (Fig. 13.22).
•Large, raised, yellowish-white area of exudation.
•Retinal hemorrhages.
•Multiple small aneurysms. The condition usually progresses to exudative RD. Probable complications include cataract, glaucoma and pthisis bulbi.
Fig. 13.22 Coats’ disease. Source: Coats’s Disease. In: Lang G, ed. Ophthalmology. A Pocket Textbook Atlas. 3rd ed. Thieme; 2015.
Differential Diagnosis
It includes other causes of unilateral leukocoria and RD in children such as retinoblastoma and toxocariasis.
Treatment
Laser photocoagulation prevents progression, if applied in early stages. It is most effective in eyes without RD. In eyes with RD, the treatment is less effective. Cryotherapy may result in marked reaction with increased leakage. Therefore, laser photocoagulation is still the preferred option.
▃Vascular Retinopathies
Retinal manifestations in systemic vascular diseases are termed as retinopathies. A retinopathy is usually bilateral, noninflammatory in origin, and associated with changes in retinal vessels in conditions like diabetes, hypertension, toxemia of pregnancy and Blood dyscrasias.
■Diabetic Retinopathy (DR) (OP8.1, OP8.2, OP8.4)
Diabetes mellitus occurs in two forms:
•Type 1 or insulin-dependent diabetes mellitus (IDDM).
•Type 2 or noninsulin-dependent diabetes mellitus (NIDDM).
Diabetes mellitus (DM) results in:
•Macrovascular complications affecting heart, brain and limbs.
•Microvascular complications (due to microangiopathy) affecting kidneys, eyes and peripheral nerves.
Ocular signs in diabetes mellitus are listed in Table 13.4.
Table 13.4 Ocular signs in diabetes mellitus | |
1.Transient change in refraction | Hyperglycemia → Increased sorbitol in lens cortex ↓ Imbibition of water with increased thickness of lens ↓ Refractive index of lens increases ↓ Myopic shift of refraction Hypoglycemia → refractive index of lens decreases ↓ Hypermetropic shift of refraction |
2.Ocular movements | Third nerve palsy with pupillary sparing. |
3.Lids | Stye or internal hordeolum. |
4.Cornea | •Infective keratitis with delayed healing. •Decreased corneal sensation due to trigeminal neuropathy. |
5.Lens | Snowflake cataract, posterior subcapsular cataract. |
6.Vitreous | Vitreous hemorrhage. |
7.Retina | Diabetic retinopathy, CRVO. |
8.IOP | Increased incidence of POAG and NVG. |
Abbreviations: CRVO, central retinal vein occlusion; IOP, intraocular pressure; NVG, neovascular glaucoma; POAG, primary open-angle glaucoma. |
Risk Factors
Risk factors for the development of DR include:
1.Type of diabetes: DR is commoner in type 1 than in type 2.
2.Duration of diabetes: DR develops in approximately 15 years and rarely develops within 5 years of the onset of diabetes.
3.Poor glycemic control: Poor glycemic control (raised HbA1c) is relevant to the development and progression of retinopathy.
4.Conditions like hypertension, poor renal status (nephropathy) and smoking also pose as a risk factor for the development of diabetic retinopathy.
Pathogenesis
DR is essentially a microangiopathy involving retinal precapillary arterioles, capillaries and postcapillary venules. DR includes both, microvascular occlusion and leakage. Retinal capillaries have two types of cells: endothelial cells and pericytes.
Vascular changes in diabetes mellitus are (Flowchart 13.5).
Flowchart 13.5 Pathogenesis of vascular changes in diabetic retinopathy. Abbreviations: IRMA, intraretinal microvascular abnormalities; NVD, neovascularization at disc in retina; NVE, neovascularization elsewhere in retina; RBC, red blood cells; VEGF, vascular endothelial growth factor.
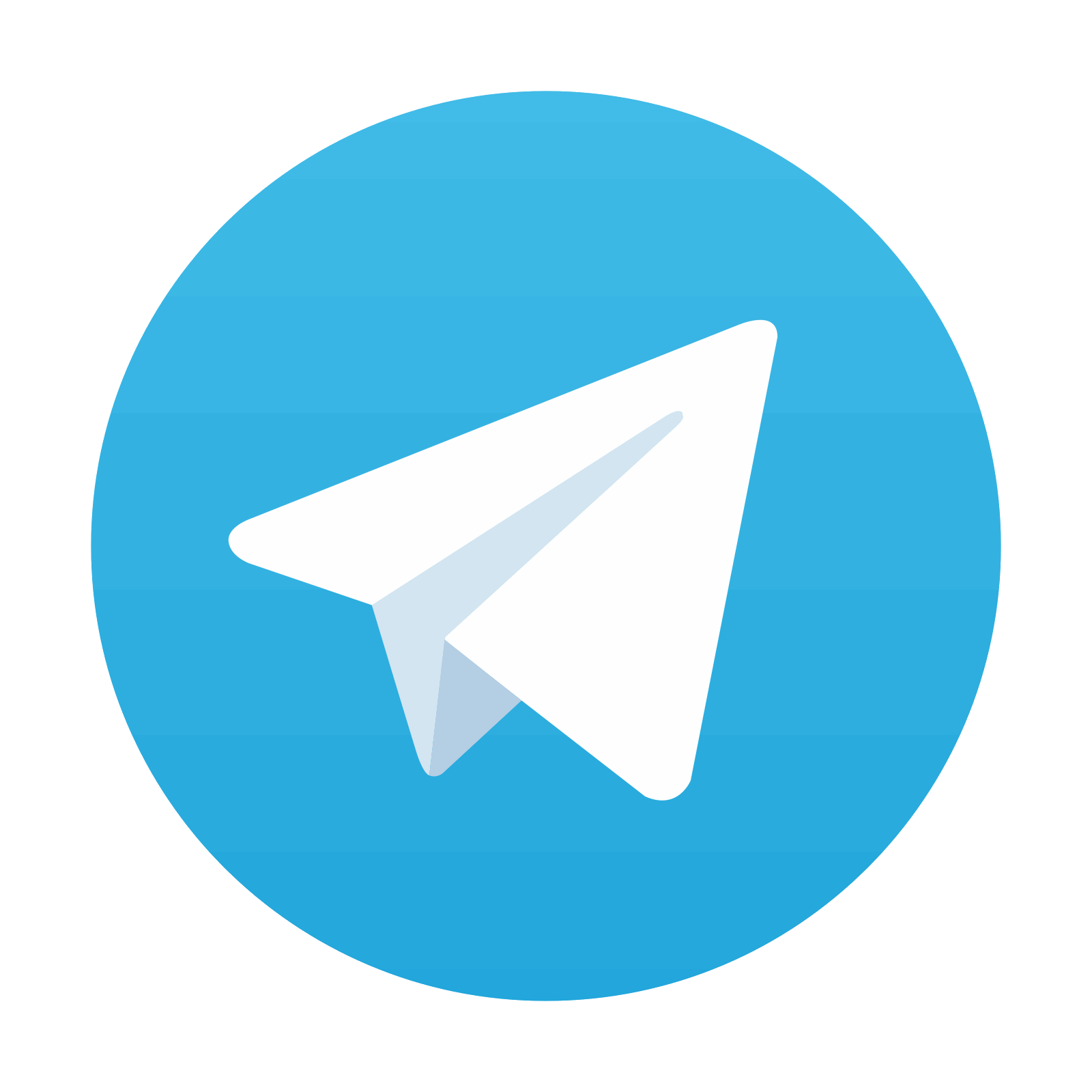
Stay updated, free articles. Join our Telegram channel

Full access? Get Clinical Tree
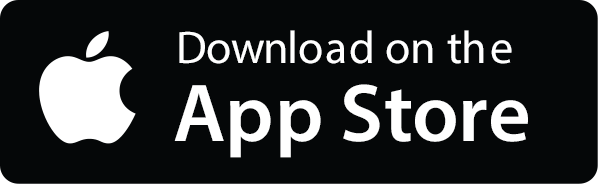
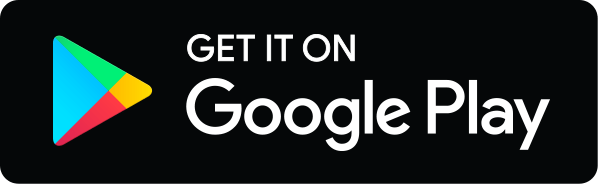