ANATOMY AND OVERVIEW OF PATHOPHYSIOLOGY
The iris
Sympathetic innervation of the pupil
Parasympathetic innervation of the Pupil
The afferent pupillary pathway and midbrain connections
AFFERENT DISORDERS: THE RELATIVE AFFERENT PUPILLARY DEFECT
MIDBRAIN DISORDERS AFFECTING THE PUPILS
Light-near dissociation
Midbrain relative afferent pupillary defects
THE PUPIL AND EFFERENT DISORDERS OF THE VISUAL SYSTEM
Pupil states without anisocoria
Anisocoria
The iris and local factors
Oculosympathetic paresis (Horner syndrome)
Parasympathetic disorders of the pupil: oculomotor nerve
Parasympathetic disorders of the pupil: tonic pupil
The pharmacologically dilated pupil
Transient pupillary dilation
Clinical evaluation of anisocoria: overview
KEY POINTS
The pupils constrict relative to the amount of light perceived by the eye; therefore, the pupil can be used as a measure of afferent visual function. This principle is the basis of the relative afferent pupillary defect (RAPD) test. When the pupillary efferent system is normal, the pupils in the right and left eye are clinically the same size as each other, even though their size is constantly changing in response to ambient light and accommodative tone, and to other factors such as mood and state of alertness.
Pupil size is ultimately determined by the balance of efferent sympathetic and para-sympathetic flow to the pupil musculature. Disorders involving these autonomic systems can cause an asymmetry in pupil size (anisocoria). The pupil, therefore, is particularly important in neuroophthalmology because it is affected in both afferent and efferent disorders of the visual system.
Pupillary signs in neuro-ophthalmic disorders can be mastered by understanding the afferent and efferent systems that control the pupil. The neuroanatomic discussion begins with the iris and efferent pupillary systems followed by discussion of the pupillary light reflex and midbrain connections. Disease processes are briefly mentioned at pertinent points in the initial discussion of the neuroanatomy, but will be addressed in detail in the remainder of the chapter.
The iris stroma readily contracts and expands in response to the forces generated by the intrinsic iris musculature (Figure 11–1). The pupillary constrictor muscle (iris sphincter) consists of a band of muscle fiber bundles encircling the pupillary aperture. Activation causes the diameter of the sphincter (and the pupil) to constrict (miosis). The muscle fiber bundles of the sphincter are segmentally innervated by parasympathetic autonomic nerves that travel from the midbrain to the orbit as part of the third cranial nerve (CN III).
Figure 11–1.
Location and orientation of iris musculature.
The parasympathetically innervated pupillary constrictor muscle runs circumferentially around the pupillary aperture at the pupillary margin. The pupillary dilator muscle is sympathetically innervated and consists of radially oriented muscle fibers.

The dilator muscle fiber bundles are radially oriented. The muscle fibers are anchored at the iris root and stretch centrally toward the papillary aperture. Constriction of these muscle fibers causes the iris to retract toward the iris root, dilating the pupil (mydriasis). The dilator muscle of the iris is segmentally innervated by sympathetic autonomic nerves.
Similar to most opposing muscle systems in the body, there is dynamic sympathetic and parasympathetic pupillary tone at all times. The balance of the two efferent systems determines the pupil size at any given moment. However, the iris sphincter muscle is much stronger than the dilator muscle, so the parasympathetic innervation of the pupil plays the dominant role in controlling pupil size.
The oculosympathetic chain begins with first-order neurons located in the posterolateral hypothalamus, with axons that descend through the brainstem and into the intermediolateral cell column of the spinal cord. These axons synapse at the ciliospinal center (of Budge) located at the C8 to T2 level in the spinal cord (Figure 11–2). Axons from these second-order (preganglionic) neurons then exit the spinal column primarily at the Tl level in the chest cavity, arch over the apex of the lung, and travel under the subclavian artery (ansa subclavia) to ascend with the cervical sympathetic plexus associated with the carotid arteries. These second-order axons pass through the stellate ganglion and ascend to synapse in the superior cervical ganglion located at the carotid bifurcation, at the level of C3/C4 and the angle of the jaw. From the superior cervical ganglion, most third-order (post-ganglionic) axons travel with the internal carotid artery through the cavernous sinus and eventually to the eye, with the remainder following the external carotid to supply vasomotor and sudomotor (sweat gland) innervation to the face. Therefore, asymmetric facial flushing and sweating are important accompanying clinical signs with localizing value.
Figure 11–2.
Oculosympathetic pathway.
The origin and course of first-order (central), second-order (preganglionic), and third-order (postganglionic) components of the oculosympathetic pathway are diagrammed. (Modified with permission from Weinstein JM. The pupil. In Slamovits TL, Burde R, associate editors. Neuro-ophthalmology, vol 6. In Podos SM, Yanoff M, editors: Textbook of ophthalmology. St Louis, 1991, Mosby.)

Sympathetic nerves bound for the eye travel as a plexus surrounding the internal carotid artery. When the third-order axons reach the cavernous sinus they condense, briefly traveling with the sixth cranial nerve (CN VI) before entering the orbit through the superior orbital fissure with the nasociliary branch of the ophthalmic division of the trigeminal nerve.
The sympathetic fibers enter the eye via the long ciliary nerves, which pierce the sclera and travel in the suprachoroidal space to innervate individual segments of the radially oriented dilator muscle. Sympathetic nerves in the orbit also travel to the upper and lower eyelids. In the upper eyelid, a small sympathetically innervated accessory muscle called Müller muscle provides 1 to 2 mm of lift to the upper eyelid. A similar but more rudimentary muscle is also found in the lower eyelid.
An oculosympathetic palsy, therefore, causes the affected pupil to be smaller (miotic) than the unaffected pupil because the parasympathetic tone to the constrictor is unopposed, and also causes an ipsilateral ptosis of the upper eyelid of 1 to 2 mm. In some patients, a slight elevation of the lower eyelid is also present, further narrowing the palpebral fissure (which contributes to the impression of enophthalmos, without being truly present).
The clinical implications of the long circuitous route of the oculosympathetic chain should be evident: Diseases in the spinal cord, chest, and neck (remote from the eye) can present with a purely ocular sign— Horner syndrome.
The pupillary constrictor muscle is innervated by the parasympathetic component of CN III. Parasympathetic pupillomotor fibers originate from the midline but paired Edinger-Westphal nuclei, which occupy the most dorsal position in the CN III nuclear complex. Fascicles from this parasympathetic motor nucleus join other fascicles from the CN III nuclear complex and traverse the midbrain tegmentum, exiting the brainstem at the interpeduncular cistern to form CN III. As described in Chapter 9, this nerve has a short but eventful course in the subarachnoid space, passing between the superior cerebellar and posterior cerebral arteries to travel both adjacent and parallel to the posterior communicating artery (see Figure 9–11B). The pupillary fibers occupy a superficial position in the nerve and are more vulnerable to external compression of CN III (eg, aneurysm or uncal herniation). CN III traverses the cavernous sinus, entering the orbit through the superior orbital fissure where the nerve bifurcates. The parasympathetic pupillary axons travel in the inferior division of the oculomotor nerve, along with fibers innervating the inferior oblique muscle. As the inferior division courses along the lateral border of the inferior rectus, the parasympathetic fibers exit midway as the motor root of the ciliary ganglion, to synapse in the ciliary ganglion (Figure 11–3). The motor root is short because the ciliary ganglion lies close to the inferior division of CN III and adjacent to the inferior rectus muscle. The ciliary ganglion is the origin of axons that enter the posterior aspect of the globe as the short posterior ciliary nerves, traveling in the suprachoroidal space to innervate both the pupillary sphincter (for pupillary constriction) and the ciliary muscle within the ciliary body (for accommodation). Many more postganglionic axons innervate the ciliary muscle than the pupillary sphincter (ratio of 30:1); this fact is important in the discussion of Adie tonic pupil later in this chapter.
Figure 11–3.
Parasympathetic pathway in the orbit.
Parasympathetic fibers travel as part of cranial nerve III, inferior division, synapsing in the ciliary ganglion. From the ciliary ganglion, postganglionic fibers enter the eye as short posterior ciliary nerves, traveling in the suprachoroidal space to innervate the iris sphincter.

Interruption of the parasympathetic pupillary pathway produces a paresis of pupillary constriction and hence a dilated pupil. As discussed in Chapter 9, a dilated pupil is a very important clinical sign when it accompanies the motility disturbances produced by a CN III palsy.
The response of the photoreceptors to a light stimulus is processed in the retina and conveyed to the brain by the retinal ganglion cell axons. There is a specialized subset of retinal ganglion cells that send their output directly to the pupillary centers in the midbrain. Evidence suggests these pupillomotor ganglion cells are melanopsin-containing, and have some intrinsic ability to respond to light on their own, in addition to input from the photoreceptors. Axons from these specialized ganglion cells travel along with axons carrying visual information in the optic nerve, chiasm, and optic tract. Similar to the axons subserving vision, the pupillary fibers undergo a slightly uneven hemidecussation in the chiasm, with a few more fibers crossing (from each nasal hemiretina) than those remaining ipsilateral (from each temporal hemiretina). This asymmetry of pupillary input is the reason that an optic tract lesion can cause a contralateral RAPD: A tract lesion disrupts input from the ipsilateral temporal hemiretina but also from the more powerful contralateral nasal hemiretina. Thus, an RAPD is present in the contralateral eye (the eye with the temporal visual field defect). Pupillary axons leave the optic tract before it terminates at the lateral geniculate nucleus (LGN), exiting via the brachium of the superior colliculus to synapse in the midbrain pretectal olivary nucleus. The pretectal olivary nuclei provide input to the parasympathetic motor nuclei (the Edinger-Westphal nuclei) (Figure 11–4).
Figure 11–4.
The pupillary light reflex.
The pupillary light reflex consists of the afferent limb from the retina to the midbrain pretectal nuclei and midbrain interconnections, with parasympathetic innervation of the pupillary sphincter (via the oculomotor nerve) serving as the efferent limb. (Reproduced with permission from Weinstein JM. The pupil. In Slamovits TL, Burde R, associate editors. Neuro-ophthalmology, vol 6. In Podos SM, Yanoff M, editors: Textbook of ophthalmology. St Louis, 1991, Mosby.)

Each Edinger-Westphal nucleus receives innervation from both the contralateral and ipsilateral pretectal nuclei. Input from the contralateral pretectal nucleus decussates at the posterior commissure. Thus, the pupillary light reflex undergoes a double hemidecussation—fibers from the right and left eyes undergo a hemidecussation at the chiasm, and fibers from each pretectal nucleus hemidecussate in the midbrain—ensuring a fairly equal distribution of light stimulation to each of the Edinger-Westphal nuclei regardless of the eye of origin. Consequently, in the normal state, the right and left pupils are essentially the same size, moving equally in concert with each other regardless of any difference in light input to each eye. Therefore, visual loss in an eye (or any other afferent visual disorder) does not cause a difference in pupil size. Anisocoria is always the result of uneven efferent (motor) innervation of the pupil. The other clinical implication of the double hemidecussation of the pupillary light reflex pathway is that light shone in one eye alone will cause equal constriction of both the ipsilateral pupil (the direct pupillary response) and the contralateral pupil (the consensual pupillary response).
The afferent pupillary pathway provides input to the Edinger-Westphal nuclei, which in turn (via the parasympathetic portion of the oculomotor nerve) drives the pupillary sphincter. This circuit constitutes the pupillary light reflex, in which the pupils constrict (in concert) with an amplitude that is proportional to the amount of light perceived by the afferent limb of the light reflex (see Figure 11–4).
The pupillary light reflex is the basis of the RAPD test: A light stimulus is alternated between the two eyes, and the pupillary response serves as a “light meter” to compare the light sensitivity of each eye. Details for performing the RAPD test are given in Figures 2–26 and 2–27.
As discussed in Chapter 2, the RAPD test is a powerful, objective clinical test. Unlike most tests of afferent visual function, the patient is not required to consciously respond during RAPD testing, and only a minimal degree of cooperation is required.
One would anticipate finding an RAPD in any disorder that causes a significant difference in visual function between the eyes, essentially any unilateral or asymmetric bilateral disorder of the optic nerve or retina. Comparing the relative difference in visual field defects between the eyes is the best predictor of the presence and magnitude of an RAPD. However, optic nerve disorders tend to produce RAPDs of relatively greater magnitude than retinal disorders.
Disorders of the ocular media (corneal scars, cataract, and vitreous hemorrhage) usually do not cause an RAPD despite poor vision, because the scattered light still reaches the retina and participates in the light reflex. In fact, a unilateral cataract distributes the stimulus light more effectively than a clear lens, producing a small apparent RAPD (usually <0.6 log unit [LU]) in the eye without cataract (Table 11–1).
CONDITIONS OTHER THAN AFFERENT VISUAL LOSS THAT CAN CAUSE A RELATIVE AFFERENT PUPILLARY DEFECT
Condition | Observation | Explanation |
---|---|---|
Cataract in one eye, clear media in the other | Small (0.6 LU) RAPD in the eye opposite the cataract | Cataract scatters the light and illuminates more of the retina than the eye with clear media. |
Ptotic eyelid or patched eye | Transient RAPD in the uncovered eye; will recover after both eyes equilibrate to the same light exposure | Retinal sensitivity is increased in a light-deprived eye. |
Unilateral exposure to a bright light | Transient RAPD in the dazzled eye; will recover after both eyes equilibrate to the same light exposure | A bright light will temporarily bleach the photoreceptors, reducing sensitivity. |
Marked anisocoria | Small RAPD in the eye with the larger pupil | The eye with the smaller pupil is relatively light deprived (develops greater sensitivity). |
Midbrain or brachium of superior colliculus lesion | RAPD in the eye opposite the lesion | Asymmetric disruption of midbrain input from the right and left eyes. |
Visual field loss from disorders of the chiasm may produce a RAPD when the visual field defects are asymmetric. Optic tract lesions may produce a small RAPD in the eye with the temporal visual field loss because the nasal retina provides greater pupillary input than the temporal hemiretina. One would not anticipate a RAPD in LGN or retrogeniculate disorders because they do not participate in the pupillary reflex arc. Why developmental amblyopia occasionally produces a small RAPD is unclear because amblyopia is considered to be of cortical origin.
In addition to the pupillary light reflex afferents, the Edinger-Westphal nuclei receive input from other midbrain nuclei that coordinate the near synkinetic reflex triad of convergence, accommodation, and miosis. Accommodation and miosis are mediated by the Edinger-Westphal nucleus, which innervates both the iris sphincter (miosis) and the ciliary muscle (accommodation) via the ciliary ganglion. The third function, convergence, is mediated by the medial rectus subnucleus.
Midbrain lesions in the region of the posterior commissure can disrupt the dorsally located pupillary light pathway by affecting the pretectal nuclei and their communication with the Edinger-Westphal nuclei, without disturbing the more ventral near-reflex fibers as they travel to the Edinger-Westphal nuclei. These disorders result in a bilateral light-near dissociation, in which the pupillary constriction to light is poor or absent, but the pupil still constricts normally to a near stimulus (Box 11–1). Examples of this condition include the dorsal midbrain syndrome and Argyll Robertson pupils. In the dorsal midbrain syndrome, the pupils are mid-dilated, and the associated findings are invariably present (see Table 10–1). Argyll Robertson pupils are small and irregular, and are a manifestation of neurosyphilis. Given the variable manifestations of neurosyphilis, testing for syphilis should be performed whenever a light-near dissociation is present. Diabetes, chronic alcoholism, encephalitis, and extensive panretinal photocoagulation can also produce Argyll Robertson–like pupils.
BOX 11–1. HOW TO LOOK FOR A LIGHT-NEAR DISSOCIATION
The pupil’s response to light is best tested by having the patient look at a distant target with both eyes open, first in dim lighting, then with bright light. If the patient is noted to have a poor pupillary response to light in one or both eyes, the pupil response to a near stimulus should be observed. A light-near dissociation may be unilateral or bilateral.
To gauge the pupil response to near, the patient is asked to focus on a visual target (with both eyes) at reading distance. The goal is to have the patient invoke the near synkinetic reflex triad of accommodation, convergence, and miosis. Therefore, it is best if an accommodative stimulus is used, such as text, or a picture, or toy for younger patients.
A proprioceptive stimulus, such as having a patient look at his or her own thumb, may be needed for the patient with poor vision.
The patient who generates a greater degree of miosis with near focus than with a bright light is said to have a light-near dissociation.
Getting the patient to generate an adequate near focus is entirely effort dependent: It may require a great deal of encouragement and several attempts. Thus, a poor near pupillary response may only mean poor patient effort.
If the pupils respond normally to light, there is no need to test pupillary reaction to a near stimulus.
Blindness is another cause of a light-near dissociation, resulting in poor pupillary response to light but pupillary constriction when attempting to look at a near object (eg, the patient’s own finger). Other causes of light-near dissociation are the result of aberrant regeneration: Adie tonic pupil and aberrant regeneration of the oculomotor nerve, discussed in a later section (Table 11–2).
CAUSES OF A LIGHT-NEAR DISSOCIATION OF THE PUPIL
Disorder | Pupil Appearance | Associated Signs and Symptoms | Pathophysiology |
---|---|---|---|
Blindness (deafferented pupil) | Bilateral normal-sized to large, no anisocoria | Poor vision, RAPD if asymmetric | Poor afferent visual input to midbrain nuclei, intact near-response input. |
Dorsal midbrain lesion | Bilateral mid-dilated to large, anisocoria possible | Other components of dorsal midbrain syndrome | Afferent pupillary input to E-W nucleus (dorsal) interrupted in midbrain; near response input to E-W (ventral) is spared. |
Argyll Robertson pupils | Bilateral small, irregularly shaped | Signs and symptoms of neurosyphilis | Afferent pupillary input to E-W nucleus interrupted (as above) by neurosyphilis (rarely MS, sarcoidosis). |
Tonic (Adie pupil) | Anisocoria with affected pupil larger than unaffected (over time the pupil can become small) | Blurred vision from accommodative abnormalities; hyporeflexia in some patients | Aberrant regeneration from ciliary ganglion. Accommodative fibers reinnervate iris sphincter. |
Aberrant regeneration of CN III | Anisocoria, affected pupil can be smaller or larger | Other signs of CN III dysfunction, aberrant regeneration of the eyelid common | Aberrant regeneration of peripheral CN III. Medial rectus fibers reinnervate pupillary sphincter (via the ciliary ganglion), activated with convergence (or horizontal gaze). |
Autonomic neuropathy | Irregularly shaped pupils | Other evidence of autonomic neuropathy | Diabetes, retinal photocoagulation (affecting the neurovascular bundle travelling in the suprachoroidal space). |
Rarely, unilateral or asymmetric midbrain lesions that interfere with the pupillary axons after they leave the optic tract, the pretectal nuclei, or the interconnections between the pretectal nuclei and the Edinger-Westphal nuclei can cause an apparent RAPD. This RAPD can occur in the absence of any visual field defect or other evidence of afferent visual loss (see Table 11–1). Similar to tract lesions, the RAPD is usually on the side opposite the midbrain lesion. Other signs of dorsal midbrain involvement are typically present, such as vertical gaze disturbance.
Normal pupil size can vary over a broad range: The pupils are large when subjects are frightened or anxious (sympathetic effect), and can become quite small with near tasks such as reading (parasympathetic component of the near synkinetic triad). The average pupil size tends to become smaller with age. Abnormally small (miotic) pupils can occur with pontine hemorrhage and opiate intoxication. Pilocarpine eyedrops for glaucoma cause a marked miosis, but this medication is now only rarely used as treatment for chronic glaucoma. Bilaterally large pupils can occur from topical or systemic drugs with anticholinergic properties such as atropine or tricyclic antidepressants.
A difference in the pupil sizes of the eyes is called anisocoria. As previously discussed, clinically significant anisocoria is always caused by an efferent (motor) disorder, not by a difference in vision between the eyes. The examiner’s task in the patient with anisocoria is to determine if the pupillary inequality is clinically significant, to discover which pupil (or both) is abnormal, to derive a differential diagnosis, and to plan an appropriate evaluation.
When the pupils are different sizes, the larger pupil may be abnormal or the smaller pupil may be abnormal. When the larger pupil is abnormal (does not constrict well), the anisocoria is greatest in bright light. When the smaller pupil is abnormal (does not dilate well), the anisocoria is greatest in darkness (Figure 11–5).
Figure 11–5.
Determining the abnormal pupil.
Observing an anisocoria in darkness and in bright light can determine which pupil is abnormal. (A) In this illustration, an anisocoria is observed in room light with the right pupil larger than the left. (B) If the smaller (left) pupil is the abnormal one, both pupils will constrict well in bright light. In darkness, the left pupil will not dilate well, maximizing the anisocoria. (C) If the larger (right) pupil is the abnormal one, both pupils will dilate well in darkness. In bright light, the anisocoria is maximized by the right pupil’s failure to constrict. (D) In an essential anisocoria, a small amount of anisocoria is present in both dark and light, and an abnormal pupil cannot clearly be determined. (E) A pupil that will not dilate or constrict is usually obvious. The anisocoria can be made to reverse, depending on the lighting conditions.

Essential, or physiological, anisocoria is a difference in pupillary size (generally <0.5 mm) that may be present in normal individuals. A pupil difference of 0.3 mm is clinically noticeable, and 20% of the normal population may have at least this amount of anisocoria at any given time. In essential anisocoria, the pupillary inequality changes from day to day and may even switch sides. This condition can be distinguished from pathological states because the degree of anisocoria remains relatively constant in light and dark (see Figure 11–5D).
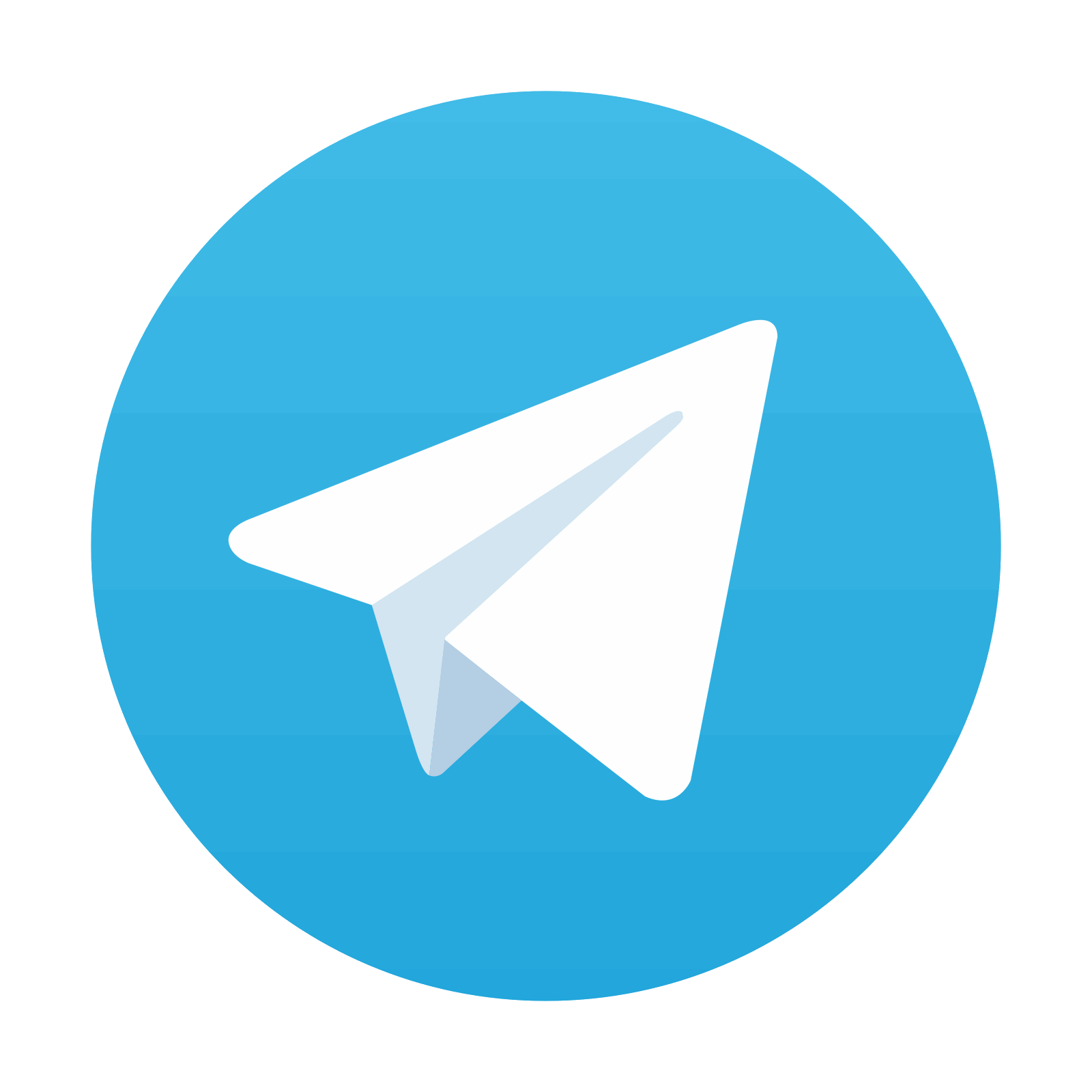
Stay updated, free articles. Join our Telegram channel

Full access? Get Clinical Tree
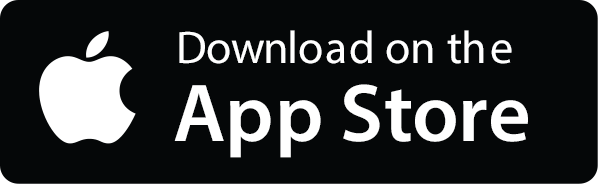
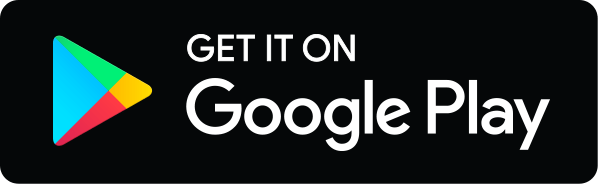