PURPOSE
To describe the clinical phenotype of a cohort of patients with Wolfram syndrome (WS), focusing on the pattern of optic atrophy correlated with brain magnetic resonance imaging (MRI) measurements, as compared with patients with OPA1 -related dominant optic atrophy (DOA).
DESIGN
Retrospective, comparative cohort study.
METHODS
We reviewed 25 patients with WS and 33 age-matched patients affected by OPA1 -related DOA. Ophthalmologic, neurologic, endocrinologic, and MRI data from patients with WS were retrospectively retrieved. Ophthalmologic data were compared with data from patients with OPA1 -related DOA and further analyzed for age dependency dividing patients in age quartiles. In a subgroup of patients with WS, we correlated the structural damage assessed by optical coherence tomography (OCT) with brain MRI morphologic measurements. Visual acuity (VA), visual field mean defect (MD), retinal nerve fiber layer (RNFL), and ganglion cell layer (GCL) thickness were assessed by OCT and MRI morphologic measurements of anterior and posterior visual pathways.
RESULTS
Optic atrophy was present in 100% of patients with WS. VA, MD, and RNFL thickness loss were worse in patients with WS with a faster decline since early age as compared with patients with DOA, who displayed a more stable visual function over the years. Conversely, GCL sectors were overall thinner in patients with DOA since early age compared to patients with WS, in which GCL thickness started to decline later in life. The neuroradiologic subanalysis on 11 patients with WS exhibited bilateral thinning of the anterior optic pathway, especially the prechiasmatic optic nerves and optic tracts. Optic tract thinning was significantly correlated with GCL thickness but not with RNFL parameters.
CONCLUSIONS
Our results showed a generally more severe and diffuse degeneration of both anterior and posterior visual pathways in patients with WS, with fast deterioration of visual function and structural OCT parameters since early age. The pattern observed with OCT suggests that retinal ganglion cell axonal degeneration (ie, RNFL) precedes cellular body atrophy (ie, GCL) by about a decade. This differs substantially from DOA, in which a more stable visual function is evident with predominant early loss of GCL, indirectly supporting the lack of a primary mitochondrial dysfunction in patients with WS.
W olfram syndrome (WS, OMIM 222300 ) is a rare autosomal recessive neurodegenerative disease that in the majority of cases is associated with bi-allelic WFS1 mutations. , WS is characterized by childhood-onset diabetes mellitus (DM), optic atrophy (OA), diabetes insipidus (DI), and deafness (D), which represent the cardinal clinical features of the disease (the previous acronym was DIDMOAD). Other common clinical manifestations of the disease may include urinary tract dysfunction (UD) and neurologic and psychiatric disorders, which eventually lead to premature death.
WFS1 encodes for the endoplasmic reticulum (ER) membrane protein Wolframin that has a role in the regulation of cellular and ER Ca2 + homeostasis, Na/K ATPase function, and regulation of the ER stress response. Wolframin is now confirmed to be enriched in the mitochondrial-associated membranes, areas of close juxtaposition between the ER and mitochondria regulating numerous key interorganelle exchanges, in particular Ca2 + flux, which is affected by WFS1 mutations. , The mechanism and functional relevance to neurodegeneration of the mitochondrial involvement in WS remains largely unsolved, despite the longstanding debate and series of controversial reports on this issue. , ,
DM and OA are diagnosed within the first decade of life and represent the 2 major criteria for a diagnosis of WS. DM is caused by pancreatic β-cell degeneration leading to a reduction of insulin levels, and it is usually the first manifestation of the disease, even if patients with WS with OA but without DM are also reported. In fact, the genetic and clinical landscape of WS recently expanded, as recessive and dominant forms with virtually identical clinical features may occur, and next-generation sequencing increasingly uncovers recessive forms of isolated OA.
A complex neurologic involvement is also frequent in WS, recently estimated as the third most frequent manifestation after DM and OA, with an early mean age at onset of 15 years. A comprehensive case series described a combination of hypo/anosmia, ataxia, seizures, dysarthria, dysphagia, neurogenic bladder, central apnea, neurogenic upper airway collapse, as well as psychiatric disturbances. Moreover, magnetic resonance imaging (MRI) scans of the brains of patients with WS typically reveals thinning of the optic nerves, optic chiasm, and tracts, but also atrophy of the cerebral and cerebellar cortex, as well as of the hypothalamic region, with prominent brainstem atrophy in particular of the pons, present in a majority of cases. , However, neurologic symptoms are often underestimated and are characterized by a progressive course, with unclear correlation with MRI findings. , ,
Pathophysiologically, Wolframin has been implicated in brain development and neurodegeneration, also impacting myelination as primary neuropathologic features. ,
OA with or without DM is an early defining manifestation of the disease required for diagnosis. Optical coherence tomography (OCT) studies demonstrated retinal nerve fiber layer (RNFL) thinning in patients without visual loss. , , Wolframin is expressed in retinal ganglion cells (RGCs) and in the unmyelinated intraretinal RGC axons. , The degeneration of RGCs leading to axonal loss has been postulated as a pathogenetic mechanism for OA in WS, as widespread axonal pathology is documented in the central nervous system , including the retinal and optic nerve RGC axons. However, only a few studies evaluated longitudinal changes of OA in WS, as well as the correlation of OA with brain MRI findings. , , Overall, the characteristics of OA in WS, how this develops, and the similarities or differences with classical mitochondrial optic atrophies like dominant optic atrophy (DOA) are currently poorly explored. DOA, a relatively more common form of inherited optic neuropathy, is a paradigm for a pathogenic mechanism leading to axonal degeneration consequent to mitochondrial dysfunction, which for the frequent OPA1 mutation is centered on dysfunctional mitochondrial fusion and dynamics.
We analyzed RGC neurodegeneration in a cohort of patients with WS compared with a well-established mitochondrial optic neuropathy, ie, DOA, proposing the possible course and pattern of RGC/axonal degeneration in both disorders. We also correlated RGC structural damage, as assessed by OCT, with brain MRI features in a subgroup of patients with WS. Finally, we evaluated the correlation, if any, between the WS phenotype and the WFS1 genotype.
METHODS
STUDY POPULATION
The clinical and genetic data of 25 patients carrying recessive biallelic WFS1 pathogenic variants were retrospectively analyzed and ophthalmologic, neurologic, endocrinologic, and MRI data were included. The ophthalmologic data of a second cohort, representative of a classical mitochondrial optic neuropathy, including 33 age- and sex-matched patients affected by OPA1 -related DOA (from here forward DOA), were compared with patients with WS. Patients with WS and DOA were evaluated at the San Raffaele Scientific Institute, Milan, Italy, and the IRCCS Istituto delle Scienze Neurologiche di Bologna, Bologna, Italy. The study adheres to the tenets of the Declaration of Helsinki and was approved by the San Raffaele Hospital, Milan (143/INT/2020) and Bellaria Hospital, Bologna (121/2019/OSS/AUSLBO – 1901), Ethics Committee. Informed consent was obtained from all participants.
CLINICAL ASSESSMENTS
Ophthalmologic, neurologic, and endocrinologic data were collected in patients with WS. Ophthalmologic data included best-corrected visual acuity (VA) using a Snellen chart, color vision tests (Ishihara test), slit-lamp biomicroscopy, Goldman applanation tonometry, color fundus photography, optical coherence tomography (OCT; DRI Triton, Topcon), automated visual field test (Humphrey Field Analyzer, protocol Sita Standard 30-2; Zeiss). OCT protocols included the evaluation of peripapillary RNFL thickness and ganglion cell layer (GCL) segmentation analysis of the macula (GCL is defined as the thickness from the inner boundary of the GCL to the outer boundary of the inner plexiform layer) (Supplementary Material, Figure S1). Only high-quality scans, defined as scans with signal strength ≥7, without RNFL discontinuity or misalignment, involuntary saccadic or blinking artifacts, and absence of algorithm segmentation failure on careful visual inspection, were used for analysis. The images were obtained using a 3-dimensional wide scan protocol with a size of 12 × 9 mm consisting of 256 B-scans, each comprising 512 A-scans. This allowed obtaining images of the macular and optic nerve head region in a single scan. Peripapillary RNFL thickness were measured using a 360° 3.4-mm–diameter circle scan with thicknesses measured across the superior, nasal, inferior, and temporal sectors and segmentation analysis of the macula measured across 6 sectors of the 6-mm–diameter circular annulus centered on the fovea included GCL.
Neurologic symptoms and signs were retrospectively retrieved from the clinical charts. In particular, the presence of cerebellar (including ataxia, dysarthria, nystagmus, and tremor), bulbar or pyramidal involvement, along with signs of polyneuropathy, myoclonus, and neurologic bladder were assessed and their frequency reported. Endocrinologic assessment was also performed to assess the occurrence of DM and DI.
NEUROIMAGING
MRI evaluation
Two experienced neuroradiologists (L.L.G., C.B.) retrospectively reviewed the available brain MRI scans of 11 patients with WS seen at both study centers. Patients were considered for the analysis if the MRI protocol included a volumetric T1-weighted image (1-mm isotropic voxel) and an axial T2 fluid-attenuated inversion recovery image. The MRI inspection analysis included morphometric and conventional evaluations.
Morphometric MRI evaluation of the anterior optic pathway
Assessments of the optic nerve and optic tract diameters were manually tracked based on the axial T1-weighted reformatted image obtained parallel to the optic nerves and tracts through the optic chiasm as previously reported , (Supplementary Material, Figure S2).
Conventional brain MRI evaluation of the optic radiation
Axial T2 fluid-attenuated inversion recovery images were visually inspected to evaluate the presence of white matter signal abnormalities (namely increased signal intensity) in the bilateral peritrigonal areas at the level of optic radiation and, if present, it was graded as slight or severe.
MRI morphometric measurements of patients with WS were compared with mean values of a population of adult healthy control subjects selected from the database of the Neuroimaging Laboratory (IRCCS Istituto delle Scienze Neurologiche di Bologna), designed to collect normative values of quantitative magnetic resonance parameters for clinical and research purposes and to normal values reported in the literature for normal control subjects 12 to 18 years of age.
GENETIC ANALYSIS
Genetic analysis was performed by direct sequencing of WFS1 or by a next-generation sequencing–based diagnostic panel designed for hereditary optic neuropathies, including WFS1 . Table 1 shows the genetic findings of patients with WS. OPA1 mutations were also confirmed in all patients with DOA (Supplementary Material, Table S1). To assess a possible genotype/phenotype correlation, different genotypic classes were defined based on the mutation type and their predicted effect on WFS1 expression, as described by de Heredia and associates 3 : class A, no WFS1 protein produced because of WFS1 mRNA degradation (A1) or because of mRNA and protein degradation (A2) or because of WFS1 protein degradation (A3); class B, reduced expression of a defective WFS1 protein; or class C, expression of a defective WFS1 protein.
Mutation | Mutation | |||||||
---|---|---|---|---|---|---|---|---|
Patient | DNA 1 | Protein 1 | Mutation Type 1 | DNA 2 | Protein 2 | Mutation Type 2 | Classification | Inheritance |
1 | c.605A>G | p.Glu202Gly | II | C.1289C>T | p.Ser430Leu | II | A3 | Recessive |
2 | c.409_424dupGGCCGTCGCGAGGCTG | p.Val142GlyfsTer110 | I | c.1628T>G | p.Leu543Arg | II | A2 | Recessive |
3 | c.2104G>A | p.Gly702Ser | II | c.1369A>G | p.Arg457Gly | II | A3 | Recessive |
4 | c.387G>A | p.Trp129Ter) | I | c.1675G>C | p.Ala559Pro | II | A2 | Recessive |
5 | c.2213C>A | p.Ala738Asp | II | c.2213C>A | p.Ala738Asp | II | A3 | Recessive |
6 | c.2452C>T | p.Arg818Cys | II | c.1515_1529del15 | p.Val509_Tyr513del | II | A3 | Recessive |
7 | c.2107C>T | p.Arg703Cys | II | c.2107C>T | p.Arg703Cys | II | A3 | Recessive |
8 | c.316-1G>A | Splice defect | I | c.757A>T | p.Lys253Ter | I | A1 | Recessive |
9 | c.409_424dupGGCCGTCGCGAGGCTG | p.Val142GlyfsTer110 | I | c.1381A>C | p.Thr461Pro | II | A2 | Recessive |
10 | c.605A>G | p.Glu202Gly | II | c.1628T>G | p.Leu543Arg | II | A3 | Recessive |
11 | c.2106_2113delCCGCTTCA | p.Arg703ValfsTer6 | III | c.2106_2113delCCGCTTCA | p.Arg703ValfsTer6 | III | C | Recessive |
12 | c.1553T>A | p.Met518Lys | II | c.1553T>A | p.Met518Lys | II | A3 | Recessive |
13 | c.409_424dupGGCCGTCGCGAGGCTG | p.Val142GlyfsTer110 | I | c.2104G>A | p.Gly702Ser | II | A2 | Recessive |
14 | c.977C>T | p.Ala326Val | II | c.977C>T | p.Ala326Val | II | A3 | Recessive |
15 | c.1928T>G | p.Ile643Ser | II | c.2194C>T | p.Arg732Cys | II | A3 | Recessive |
16 | c.1928T>G | p.Ile643Ser | II | c.2194C>T | p.Arg732Cys | II | A3 | Recessive |
17 | c.2002C>T | p.Gln668Ter | III | c.2126T>G | p.Val709Gly | II | B | Recessive |
18 | c.1628T>G | p.Leu543Arg | II | c.2104G>A | p.Gly702Ser | II | A3 | Recessive |
19 | c.370T>C | p.Cys124Arg | II | c.2213C>A | p.Ala738Asp | II | A3 | Recessive |
20 | c.1675 G>A | p.Ala559Thr | II | c.1381A>C | p.Thr461Pro | II | A3 | Recessive |
21 | c.605A>G | p.Glu202Gly | II | c.605A>G | p.Glu202Gly | II | A3 | Recessive |
22 | c.1541T>G | p.Leu514Arg | II | c.1541T>G | p.Leu514Arg | II | A3 | Recessive |
23 | c.387G>A | p.Trp129Ter | I | c.387G>A | p.Trp129Ter | I | A1 | Recessive |
24 | c.1381A>C | p.Thr461Pro | II | c.2099G>A | p.Trp700Ter | III | B | Recessive |
25 | c.2206G>A | p.Gly736Ser | II | c.2206G>A | p.Gly736Ser | II | A3 | Recessive |
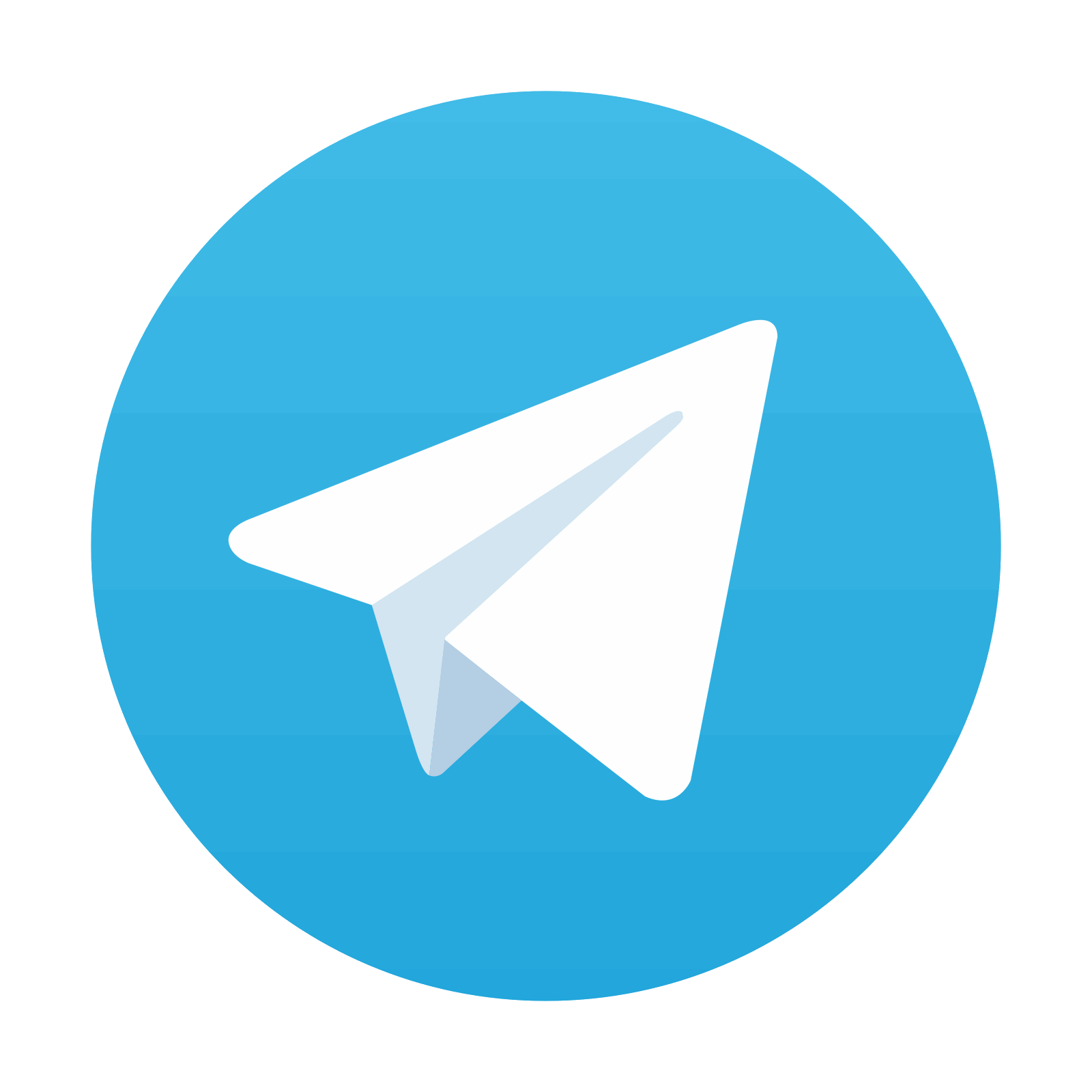
Stay updated, free articles. Join our Telegram channel

Full access? Get Clinical Tree
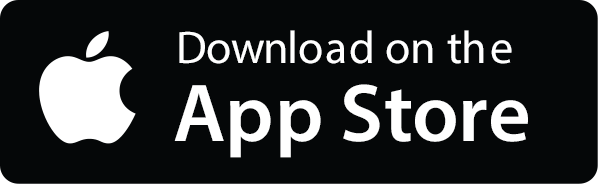
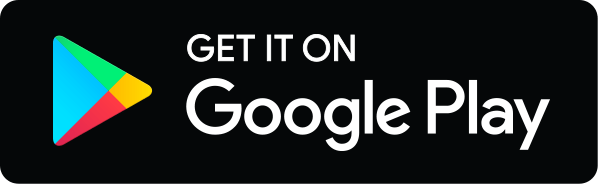
