© Springer Japan 2015
Juichi Ito (ed.)Regenerative Medicine in Otolaryngology10.1007/978-4-431-54856-0_22. The Inner Ear
(1)
Department of Otolaryngology-Head and Neck Surgery, Graduate School of Medicine, Kyoto University, Kyoto 606-8507, Japan
Abstract
Diseases- and symptoms-related inner ear dysfunctions such as sensorineural hearing loss (SNHL), tinnitus, and vertigo are very difficult to treat. One of the main reasons is caused by the difficulty that inner ear sensory cells have little ability to regenerate after damage. There are more than 400,000 deaf or highly hearing-impaired people in Japan. One of 1,000 newborn babies is deaf. Therefore, recovering inner ear disorders has always been one of the most important priorities in the field of otolaryngology. In this chapter, the possible therapeutic strategies for inner ear regeneration are summarized. In early-phase cochlear damage, it is crucial to rescue auditory cells from cell death and promote self-repair cell activity. Induction of transdifferentiation is the next useful strategy. If no cell sources remain in the inner ear, cell transplantation then becomes the only choice to restore cell growth through regeneration. Using bionic materials is another remaining possible alternative approach. Detailed related inner ear regeneration is already described in another edition (Ito J. Regenerative medicine for the inner ear. Tokyo: Springer, 2014).
Keywords
Inner earRegenerationStem cellsTransdifferentiationTransplantationArtificial auditory epithelium2.1 Anatomy of the Inner Ear
Inner ear of mammals consists of the cochlea, the vestibule, and three semicircular canals. The lumen of the otic capsule is called bony labyrinth and the inner membranous wall is called membranous labyrinth. The space between the bony and membranous labyrinth is filled with perilymph. Inside the membranous labyrinth is called endolymphatic space, and is filled with endolymph. The perilymph is sodium rich and the endolymph is potassium rich.
The sensory epithelium of the cochlea is the organ of Corti, in which sensory hair cells stay in array. The axis of the cochlea is known as the modiolus, in which the cochlear nerve runs. The cross section of the membranous labyrinth in the cochlea forms a triangular shape bordered by the basilar membrane, the stria vascularis, and the Reissner’s membrane [1]. The endolymphatic space inside the cochlea is called scala media. The perilymphatic space facing the Reissner’s membrane is called scala vestibuli, and the space facing the basilar membrane is called scala tympani. The basilar membrane in the basal turn is narrower and stiffer, leading to higher natural resonance frequency, while the apical turn leads to lower resonance. The organ of Corti is composed of hair cells and several types of supporting cells. When the sound vibration displaces the basilar membrane, the shearing force between the basilar and the tectorial membranes deflects the hair bundle at the apical surface of the inner hair cells and then is conveyed as the release of the neurotransmitter.
Approximately 3,500 inner hair cells exist in one human cochlea. The outer hair cells appear as three rows, and 12,000 outer hair cells exist in one human cochlea. The outer hair cells are innervated by type II afferent neurons with unmyelinated axons.
Loose connective tissue on the outer wall of the cochlear duct is the spiral ligament. The stria vascularis is a vascular-rich structure on the spiral ligament spreading from the attachment of Reissner’s membrane to the spiral prominence. The stria vascularis functions to maintain the high potassium concentration and positive potential of the endolymph.
The vestibule composed of the utricle and saccule functions to sense linear acceleration. The space near the semicircular canals is called the utricle, while the other located near the cochlea is called the saccule. The sensory epithelium of the vestibule is located in the macula of the utricle and saccule. The utricular macula is located horizontally with a free margin. The saccular macula is attached to the medial wall of the saccule. Hair cells in the maculae are classified into type I and II hair cells, which are morphologically distinguishable, but the functional difference is not fully understood.
The three semicircular canals (lateral, superior, and posterior) contribute to the stimulus perception of three-dimensional head rotation. In each semicircular canal, the membranous labyrinth presents as a tube-like structure. At one end of each semicircular canal, the tube is dilated to form a spindle-like structure, which is known as the ampulla. A crescent-shaped structure (crista) is located inside the ampulla. The crista is attached to the outer wall of the ampulla, and the hair cells of the semicircular canals are located in the sensory epithelium of the crista.
2.2 Regeneration of the Inner Ear
2.2.1 Stem Cells
2.2.1.1 Inner Ear Stem Cells
Mammalian inner ears were reported to contain cells that had the ability to proliferate clonally and to differentiate into several types of inner ear cells. Regeneration was reported to occur in vestibules, while the mammalian cochlear cells have been reported to have little ability to regenerate after damage. The inner ear has limited ability to regenerate, while there are other evidences indicating the existence of inner ear stem cells. Potential candidates of stem cells are supporting cells [2], tympanic border cells [3], and other candidates. The total number of them in the inner ear is estimated to be very small, and reported to decrease as the inner ear matures. Tympanic border cells identified as slow-cycling cells have the characteristics of stem cells [3]. Immunohistochemical analysis indicated that these cells stained positive for immature cell marker nestin. The number of slow-cycling cells in the tympanic border cells decreased dramatically in about 2 weeks after birth as the cochlea matured.
2.2.1.2 Embryonic Stem Cells (ESCs)
ESCs are widely used for the generation of knock-out/knock-in animals, chimeras, and clones. Nowadays, these genetically modified animals are indispensable tools for biological research and animal industry. Extending this study, ESCs were induced to differentiate into a wide variety of cell types including hepatocytes, pancreatic islet cells, blood cells, chondrocytes, cardiomyocytes, dopaminergic neurons, Schwann cells, retinal pigmented epithelium and so on, and expected to be applied in clinics.
2.2.1.3 Induced Pluripotent Stem Cells (iPSCs)
iPSCs are somatic-cell-derived stem cells similar to ESCs in terms of self-renewal and pluripotency [4]. iPSCs are similar to mouse ESCs in many points such as an expression of stem cell. The regeneration of the auditory pathway by transplants of fetal brain tissue was first demonstrated [5]. Since then several stem cell-based approaches to cure deafness using mesenchymal stem cells, neural stem cells, ESCs, and iPSCs have been adopted. Current major targets are cochlear hair cells and spiral ganglion neurons (SGNs), which are primarily compromised and unregenerable cells in sensorineural hearing loss. Inner ear hair cells of the mammals have very little capacity of regeneration [6]. This is one major reason for the difficulty in recovering from permanent profound hearing loss. One suggested modality for the recovery from the hearing impairment is the regeneration of hair cells. Hair cell induction from pluripotent stem cells has gradually become a reliable method. Hurdles still exist before the realization of hair cell regeneration. It is still necessary to improve the induction rate, and to develop the method to specifically induce cochlear hair cells. More fundamental difficulty is the in vivo application of induced hair cells. It is not realistic to transplant mature hair cells and to arrange them in the cochlear epithelium [7]. Introduction of stem cells that are programmed to differentiate to inner ear sensory fates and introduction of stem cell-based factor releasing cells may also be other possible strategies.
2.2.2 Transdifferentiation
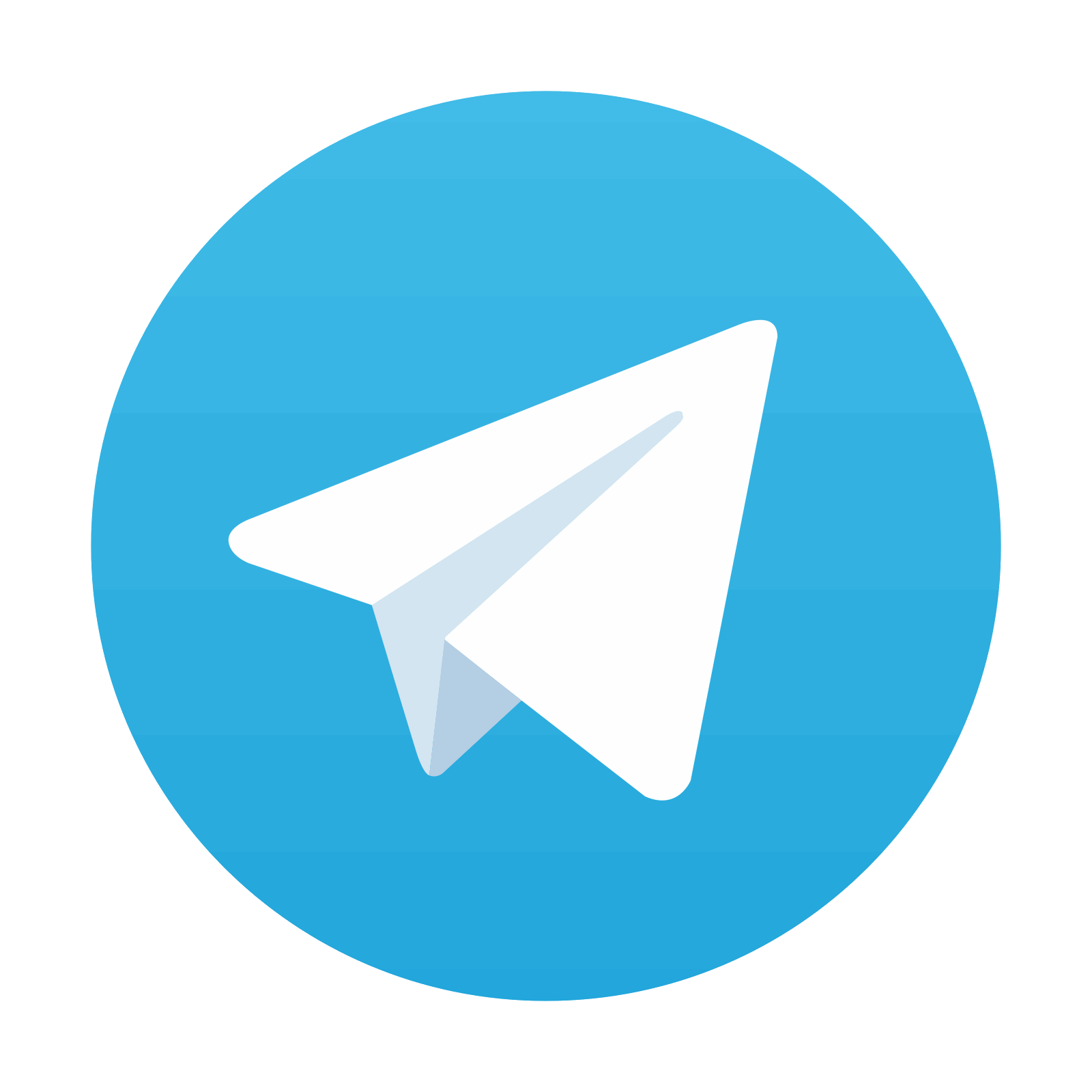
Stay updated, free articles. Join our Telegram channel

Full access? Get Clinical Tree
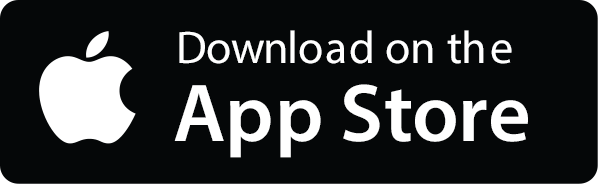
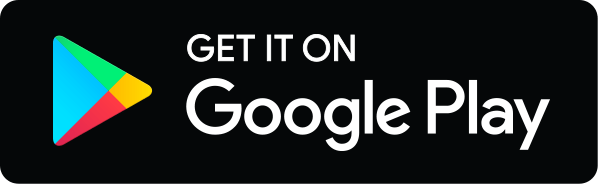