Fig. 1.1
Orbital cellulitis on the right side presenting with significant upper and lower eyelid edema (Image courtesy of Michael T. Yen, M.D.)
The classification of orbital cellulitis can be traced back to 1937, when Hubert published a seminal paper describing infections around the orbit arising from sinusitis [6]. In 1970, Chandler et al. modified Hubert’s classification and presented a five-level classification system for orbital cellulitis which is still used today [4]. In this classification, group I consists of patients with edema confined to the eyelids only. Group II represents true orbital cellulitis, manifested by edema of the eyelids, diffuse orbital edema, vision changes, and painful eye movements. Group III is characterized by the presence of a subperiosteal abscess (SPA), which is marked by accumulation of pus between the periosteum and the orbital bones. Group IV is distinguished by the presence of a true orbital abscess, which causes more severe proptosis and ophthalmoplegia. Group V is the end stage of orbital cellulitis with extension of the infection into the CNS, causing cavernous venous thrombosis [4, 7–9].
Perhaps the development that had the greatest impact in the evaluation and management of orbital cellulitis was the introduction of computed tomography (CT) in the late twentieth century (Fig. 1.2). Since then, imaging has played an important role in the diagnosis of orbital cellulitis and, in particular, subperiosteal abscesses [2]. Controversy among scientists regarding the use of CT as part of the initial workup of SPAs has existed since the introduction of the CT, especially since abscess formation is not visible on a CT scan when it is in the early stages [10, 11]. Some physicians maintain that, due to the aggressive nature of SPAs, CT scans should be obtained in all cases even if the initial presentation is consistent with preseptal cellulitis. Other authors have reported orbital ultrasound to have a better sensitivity for orbital abscesses [10]. However, the general consensus is that ultrasound has poor resolution at the orbital apex and does not allow the simultaneous visualization of the surrounding sinuses and intracranial tissues [10].


Fig. 1.2
Coronal CT scan demonstrating complete opacification of the maxillary and ethmoid sinuses associated with a subperiosteal abscess in the medial orbit on the right side (Image courtesy of Michael T. Yen, M.D.)
Etiology
In the early twentieth century, the most common etiology of orbital cellulitis in adults was frontal sinusitis; these patients would often present with downward and outward displacement of the globe [1]. In children, suppuration of the ethmoid sinus was the most common cause of orbital inflammation in the early twentieth century, and these patients presented with the globe displaced outward [1]. Prior to 1985, the most common causative organism in children was H. influenzae, and, until a specific organism was identified in children presenting with orbital cellulitis, initial therapy was always directed against this organism [12]. After introduction of the H. influenzae vaccine in 1985, however, Staphylococcus aureus and Streptococcus species emerged as the most common causative organisms.
Currently, sinus infections still remain the most common cause of orbital inflammation in children. About 60% of all orbital inflammatory processes extend from sinusitis, with ethmoiditis being the most common source [4]. In adults, modern-day orbital cellulitis is usually the result of a recent illness or trauma, such as orbital fractures and intraorbital foreign bodies [13].
Epidemiology
Prior to the 1940s, when antibiotics first came into widespread use, 17% of orbital cellulitis patients died, and 20% of the patients lost vision [3, 9]. Over time, however, with increased awareness of possible complications and better techniques of diagnosis and management, these rates have declined dramatically. By the late twentieth century, the rate of blindness dropped to 3–11%, while the rate of mortality was reduced to 1–2.5% [3]. In cases where infection spreads to the cavernous sinus or to the intracranial area, however, the rate of morbidity and mortality still remains high with incidences cited between 10–20% [14]. Fortunately, intracranial complications have now become a rare occurrence, and in recent years, the incidence of these complications has decreased to less than 1% [9].
Globally, orbital cellulitis is primarily a disease of children and adolescents. In the United States, the condition is reported to be most common in patients under 15 years of age, with the median age being 7 years [15]. The high incidence of the disease in children can be explained by the underdeveloped immune system, especially the underproduction of IgGs in infants between the ages 1 and 5 years, which makes them more susceptible to infections by encapsulated organisms such as Haemophilus influenzae and Streptococcus species [13, 16, 17]. The incidence of orbital cellulitis due to H. influenzae has significantly decreased since the early 1990s due to widespread immunization, resulting in a decrease in the overall incidence of orbital cellulitis [15, 16].
Medical Management
The medical and surgical management of orbital cellulitis has undergone shifts over the course of time. In the early twentieth century, treatment options were limited. Since the introduction of antibiotics to the treatment of orbital cellulitis in the 1940s, the overall incidence of complications of orbital cellulitis has decreased.
In the mid-twentieth century, the most common pathogens causing orbital cellulitis in children were identified as aerobic bacteria, namely, Haemophilus influenzae, streptococci, and staphylococci [12]. Other less common organisms included Klebsiella, Micrococcus, and Enterococcus [12]. The mainstay for initial therapy at this time included empiric treatment with penicillin in combination with penicillinase-resistant antibiotics, such as methicillin, or in combination with other synergists, such as streptomycin, sulfonamides, or chloramphenicol [12, 18–21]. In some cases, high doses of ampicillin were initially administered, along with intranasal decongestants, sedatives, and analgesics [4]. Cases of orbital cellulitis that were complicated by an abscess were reported to be successfully managed with antibiotic injections, penicillin ointment, and hot fomentations, which caused the abscess to burst and allowed the pus to drain [22]. Visual changes and proptosis were seen to improve within days of this intervention [22]. In adults, anaerobic coverage was added to the coverage against aerobic organisms [23]. In general, anaerobes were susceptible to penicillin, metronidazole, and chloramphenicol [24]. While cefoxitin and clindamycin also provided good coverage against anaerobes, they had the disadvantage of poor CNS penetration [24].
In the late twentieth century, antibiotic recommendations changed with the introduction of new generations of pharmacodynamically optimal antibiotics, such as third-generation cephalosporins [2, 25]. Ceftriaxone replaced the older combinations of antibiotics and helped avoid the bone marrow suppression previously caused by chloramphenicol [2]. In patients allergic to penicillin or cephalosporin, vancomycin was used as an alternative medication [2, 7, 15]. For anaerobic coverage, cephalosporins were used in combination with clindamycin [2].
The introduction of H. influenzae vaccine has been an important preventative tool in decreasing the incidence of H. influenzae as the most common causative organism for orbital cellulitis, as well as decreasing the incidence of orbital infection in the pediatric population as a whole [10, 26]. The H. influenzae vaccine, however, does not provide protection against nontypeable H. infleunzae [15]. Consequently, the most likely pathogens now causing orbital cellulitis are nontypeable H. influenzae and Staphylococcus and Streptococcus species, with Streptococcus anginosus emerging as a pathogenic group in the pediatric population [15, 27]. Moraxella catarrhalis and anaerobic bacteria have also been identified, although less commonly, as causative bacteria of orbital cellulitis [28]. In the twenty-first century, adequate antibiotic coverage for pediatric orbital cellulitis includes a combination of beta-lactamase-resistant penicillin for Streptococcus or Staphylococcus species, clindamycin for anaerobes, and a third-generation cephalosporin for H. influenzae, Moraxella, and resistant pneumococci [15].
In recent years, newer fluoroquinolones , such as gatifloxacin and moxifloxacin , have shown promising results in the treatment of orbital infections [29]. Older fluoroquinolones preferentially inhibited DNA gyrase in gram-negative organisms and DNA topoisomerase IV in gram-positive organisms. In contrast, the new generation of fluoroquinolones has a dual mechanism of action, where they are able to inhibit both enzymes involved in bacterial DNA synthesis in both gram-positive and gram-negative organisms [29].
In recent years, bacterial resistance to antibiotics has been a major concern, particularly due to the rising incidence of community-acquired methicillin-resistant Staphylococcus aureus (MRSA). About 20% of S. aureus isolates from orbital and sinus cultures are identified as MRSA [9]. A study conducted in France found 30% of H. influenzae and 80% of Moraxella resistant to beta-lactams [10, 28]. Over time, pneumococci also became increasingly resistant to macrolides and penicillin G [28]. In efforts to combat the rising resistance against antibiotics, the Centers for Disease Control and Prevention and the World Health Organization have proposed some strategies to delay the development of antibiotic resistance [29]. Current recommendations include the fact that antibiotics should be prescribed only when needed and should be selected based on the identification of causative pathogens and their sensitivity testing, eventually switching to oral therapy [27, 29]. Furthermore, the course of antibiotic therapy should be completed in its entirety, and the use of antibiotics for optimization of animal and agricultural growth should be avoided [29].
Surgical Management
Over the years, controversy over the necessity and timing of surgical intervention in the management of orbital cellulitis and SPAs has persisted. Clinical signs of developing an abscess include increasing edema of the eyelid, worsening of visual acuity, fixed displacement of the globe, and severe pain [1, 4]. As early as the 1930s, recommendations for initial therapy of orbital cellulitis with SPA were to treat with intranasal medications until localization of the abscess occurred, followed by drainage of the abscess [19, 30]. A 1935 paper by Layton suggested that acute cases of orbital cellulitis with SPA should be managed with the least amount of surgical intervention in order to prevent the seeding of adjacent structures with bacteria from orbital infections and reduce the risk of complications such as osteomyelitis and cavernous sinus thrombosis [30].
Prior to the 1980s, surgical management of SPAs involved rapid decompression of the abscess [9]. In the pre-antibiotic era, progressive orbital signs were an indication for external drainage that involved the opening up of sinuses, removing the infected bone, and draining any medially located SPAs [31]. During this time period, the surgical approach to managing SPAs secondary to sinusitis depended on the sinuses involved. Frontal sinuses were opened externally by making a Moore’s incision underneath the eyebrow. Rubber tubes were then placed to drain the sinuses into the nasal fossa [32]. Ethmoid sinuses, on the other hand, were drained intranasally by performing external ethmoidectomy, which involved creating Lynch incisions between the nasal bridge and the eye to enter the sinus and then removing the middle turbinate [18, 32]. Maxillary sinuses were visualized by the Caldwell-Luc approach, where an incision was made underneath the lip along the upper gumline [3]. The sinus was then drained by performing an intranasal antrostomy, and a rubber drain was left in place before loosely closing the external incision [18]. Suction apparatus supplemented the passive flow of discharge from the nose and incision wounds [33]. Lastly, any fluctuating area in the temporo-malar region, indicating the presence of a superficial abscess, was incised and drained [32]. While these procedures provided good visualization for evacuation of SPAs, they also caused significant discomfort and prolonged recovery. By the late twentieth century, surgical exploration and drainage of the sinuses were reserved for patients who did not respond to medical treatment within 24–48 h [23, 34, 35].
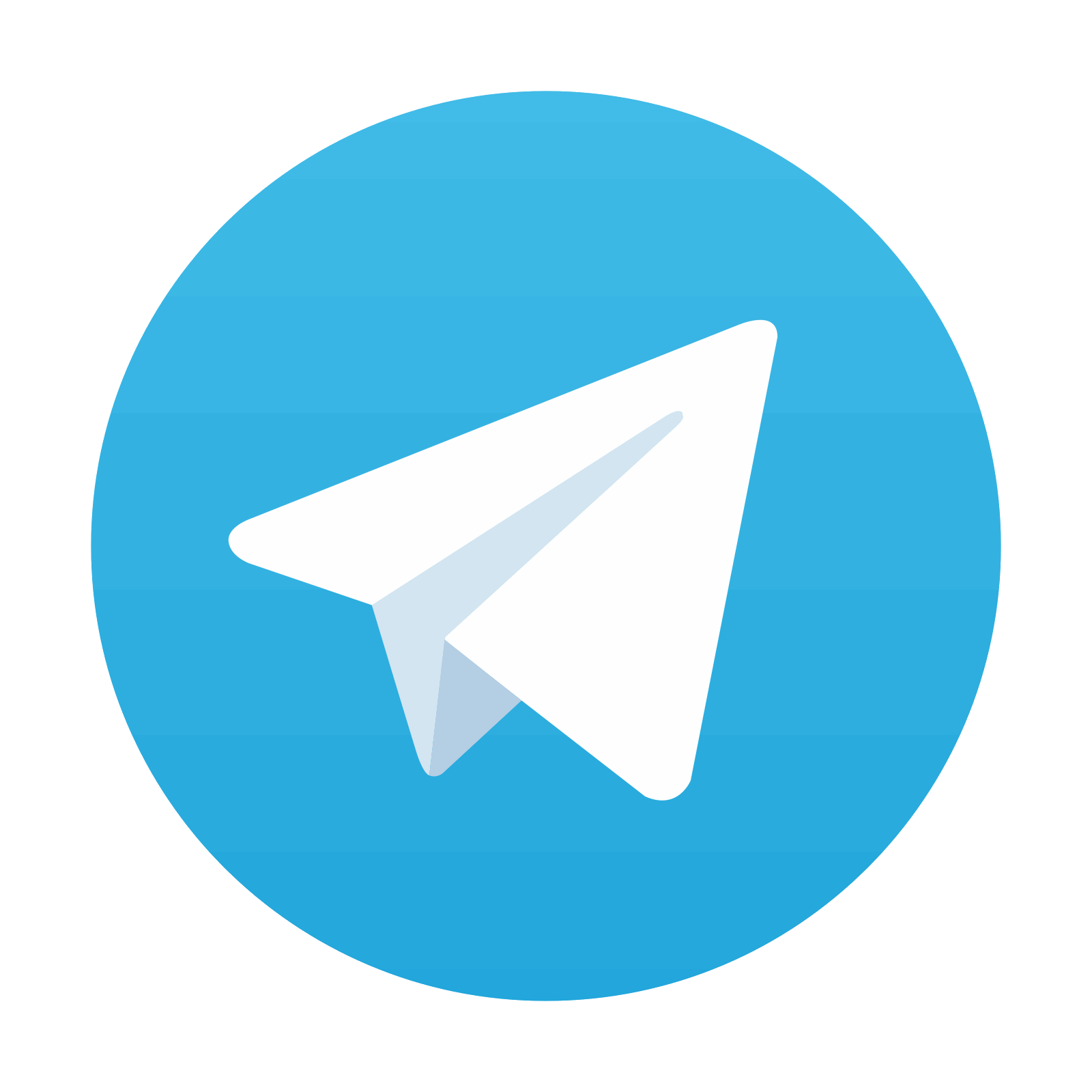
Stay updated, free articles. Join our Telegram channel

Full access? Get Clinical Tree
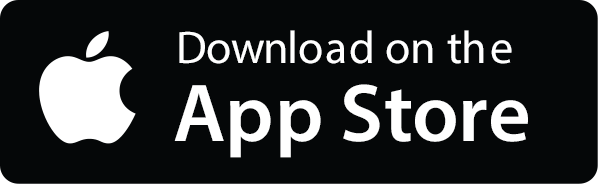
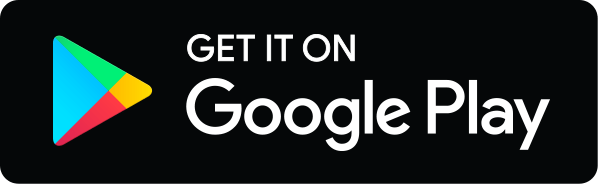