27 The Femtosecond Laser and the Posterior Segment
Summary
Femtosecond lasers hold promise in OCT guided intraretinal ablation for removal of tissue with a new level of precision, treatment of vitreous floaters, induction of laser assisted posterior vitreous detachment as well as in glaucoma surgery by creating openings in the trabecular meshwork. However there are significant challenges yet to be overcome for laser delivery in these situations.
Keywords: Femtosecond laser, retina, posterior segment, vitreous, vitreous detachment, vitreous floaters, laser induced optical breakdown, intraretinal ablation, femtosecond laser trabeculectomy
27.1 Introduction
While the precision of femtosecond lasers has revolutionized the fields of cataract and refractive surgery, their use in the posterior segment is still at a very nascent stage. Various applications have been suggested for femtosecond lasers in the posterior segment, but none are as yet commercially available. There are several anatomical considerations that limit the applications of the femtosecond laser in the posterior segment and have prevented its commercialization so far. The tissue treated with the femtosecond laser needs to be avascular and transparent. This limits the use of the laser currently to the cornea, aqueous, lens, and vitreous. The sclera can potentially be made transparent with hyperosmotics, which would make scleral incisions possible. In order for the femtosecond laser to be used in the posterior segment, the energy level has to be low enough so as to not cause collateral thermal damage of the corneal or lens tissue along the path of the laser beam. The femtosecond laser does have a learning curve, but refractive and cataract procedures performed with the laser have shown that it is a short curve with highly reproducible results and this should be applicable to posterior segment delivery as well. In contrast to thermal energy produced by visible and infrared lasers, such as neodymium-doped yttrium aluminum garnet (Nd:YAG) systems, the high photon energies of femtosecond lasers can photodisrupt the tissue, without significant target tissue temperature elevation. The absorption of high-energy photons from femtosecond lasers released at photodisruptive fluence levels by the target tissues allows for this minimal collateral damage. In vitreoretinal surgery, one of the major advantages of the femtosecond lasers would be in selective and precise treatment of vitreous traction, and with low energy and no collateral thermal damage. The treatments have the potential to provide more precision and reproducibility than conventional manual surgery, or even a nonsurgical alternative, thereby expanding the armamentarium of retinal treatments.
This chapter reviews the current literature on the impact of femtosecond lasers on the posterior segment as well as several future potential applications of the femtosecond laser for vitreoretinal and glaucoma surgery.
27.2 Safety of Current Femtosecond Laser Delivery on the Posterior Segment
The effect of laser delivery using the current-generation femtosecond laser machines has been demonstrated to be safe on the macula. Postoperative macular thickness measurements using optical coherence tomography (OCT) have been shown to be similar with conventional phacoemulsification and femtosecond laser–assisted cataract surgery (LCS). 1
During the femtosecond laser procedure, a suction ring is applied to avoid eye movements and laser misdirection, exerting pressure on the pars plana region at the limbus. Previous experimental and clinical studies demonstrated that the application of the suction ring causes short but considerable fluctuations (up to 40 mm Hg with the LenSx platform, Alcon, Fort Worth, TX) in intraocular pressure (IOP), 2 which can induce several changes in ocular structure—from the deterioration of goblet cells of the conjunctiva up to the retina. During application of microkeratome suction in laser-assisted in situ keratomileusis (LASIK), where a greater increase in IOP occurs, a decrease in lens thickness and an increase of vitreous distance have been described, suggesting anterior traction on the posterior segment. 3 These alterations can cause posterior hyaloid detachment, transient choroidal circulation abnormalities, macular hemorrhage, and optic atrophy. 4, 5
27.3 Incidence of Cystoid Macular Edema with LCS
With rising expectations from cataract surgery, the incidence of subclinical macular edema after uneventful cataract surgery has become an important consideration. An increase of the perifoveal retinal thickness with OCT can be detectable from the first week up to 6 months and peaks 4 to 6 weeks after surgery, in pseudophakic eyes. 6, 7
In a study of 220 eyes, Conrad-Hengerer et al 8 reported that LCS did not influence the rate of postoperative cystoid macular edema (CME). The center point thickness was similar at 4 days, 1, 3, and 6 months postoperatively in the LCS and conventional phacoemulsification groups. Laser flare photometry showed higher levels in the standard group at the first postoperative visit 2 hours after surgery compared with the laser group. Similar results were reported by Ecsedy et al 1 in a series of 20 eyes. Nagy et al reported that CME was detectable mainly in the outer nuclear layer in both LCS and conventional phacoemulsification groups but was significantly less using the femtosecond laser platform. 9
27.4 Future Applications: Review of Current Patents
This section reviews the currently filed patents to predict the future applications of femtosecond lasers in vitreoretinal surgery.
Femtosecond pulses of appropriate intensity can induce nonlinear absorption in the focus of the laser beam, causing disruption of tissue at the focus while leaving tissue outside of the focus mostly intact. Ocular aberrations anterior to the target location can cause spatial distortion in the laser beam, resulting in an increase of pulse energy threshold: for photodisruption and corresponding damage to surrounding tissue. Dispersion within the eye anterior can cause a temporal extension in the laser pulse, providing a second source of distortion resulting in an increase of pulse energy threshold for photodisruption and corresponding damage to surrounding tissue. This is the reason why it has not been feasible to direct transcorneal pulses to retinal and preretinal structures. 10
The ideal femtosecond laser system for vitreoretinal applications would be capable of precisely disrupting preretinal and retinal tissue, target location in the preretinal vitreous tissue or retinal microstructure, have an imaging element to image the target location to produce an in vivo image, and an adaptive optical element to correct laser pulses to compensate for the optical aberrations from ocular tissue anterior to the vitreous and retina. The adaptive optical element can comprise one of a deformable mirror, a phase modulator, and another suitable mechanism for adjusting the optical properties of the laser pulse. 10
The concept of an OCT-guided femtosecond laser to treat target tissue in the vitreous cavity of an eye has also been envisioned before. The target location can be imaged by an OCT imaging system to assist the operator in focusing the femtosecond laser pulses to a desired target location.
27.5 OCT-Guided Femtosecond Laser–Assisted Intraretinal Ablation
In a porcine study, Hild et al used near-infrared femtosecond lasers to irradiate porcine retinal specimens in vitro. The lasers were used for tissue removal as well as multiphoton laser scanning microscopy. Ablation of the nerve fiber layer was performed at pulse energies of 1.0 to 3.9 nJ, suggesting that nonamplified femtosecond lasers may allow precise surgery controlled by fast high-resolution imaging of the target. 11
Bausch & Lomb (Rochester, NY) filed a patent in 2012 for an OCT-guided femtosecond laser to measure the retinal surface and allow for intraretinal ablation. 12 The technique was designed for performing an ablation for the purpose of debulking scar tissue in the retina, which was first identified using OCT. In order for the retinal functionality to be preserved, it is important that the retinal surface not be unduly manipulated. This requires a removal of scar tissue from the retina with extreme precision and effectiveness. In the event, the removal of scar tissue from within the retina can require operational tolerances as small as 10 to 50 μm. Femtosecond laser systems can be operated to perform tissue ablation by laser-induced optical breakdown (LIOB) within such tolerances. The imaging unit includes an analyzer for creating and evaluating the three-dimensional retinal image.
Van de Velde filed a patent in 2010 for an electronic ophthalmoscope that combined a scanning laser ophthalmoscope and OCT coupled to a femtosecond laser for selective retinal photodisruption of the photoreceptor layer of the retina. 13 The femtosecond pulses are intended to limit the destructive laser tissue impact to the retinal pigment epithelium, by delivering the energy to the photoreceptor layer in a confined manner and sparing the choriocapillaris and the inner retinal layers.
27.6 Femtosecond Laser Surgery Device for the Vitreous Humor
Carl Zeiss Meditec (Jena, Germany) envisioned a device in 2011 for a femtosecond laser surgery device for the vitreous humor of the eye. 14 The device consists of an ultrashort pulse laser with pulse widths of approximately 300 fs, pulse energy approximately 1 to 2 μJ and pulse repetition rates of approximately 500 kHz. The laser system would be coupled to a scanner system to allow spatial variation of the focus in three dimensions and an OCT-based navigation system coupled to it. Measurement methods such as triangulation, and fringe projection would provide location information of the therapeutic target areas in the retina or the vitreous. The laser delivery in the vitreous body would be controlled so that it did not exceed the radiation exposure limits of the retina. The energy and power density are based on an optical model computed locally on the retina and the temporal and spatial sequence of the applied pulses are varied till the radiation exposure reaches the maximum limit.
The vitreous has three areas of maximum adhesion. These are Wieger’s ligament at the periphery of the posterior lens capsule, Salzmann’s vitreous base in the region of the ora serrata, and Martegiani’s ring near the papilla (▶ Fig. 27.1). These regions frequently are the points of origin of the maximum vitreoretinal traction. Using the femtosecond laser, minimally invasive “relief” incisions can be potentially performed in the region of these three zones. For the incision in the area of Salzmann’s vitreous base, a contact glass with integrated deflection mirrors (the so-called mirror contact glass) could be used to focus the laser radiation in the extreme periphery of the eye. An adaptive mirror system could be introduced in the beam path for compensating the wavefront distortions that occur during focusing of the laser radiation in the eye at the required acute angle of incidence and thus increasing the incision quality. According to the invention, with an incision near Wieger’s ligament, an anterior vitreous detachment can be induced. This can be performed in conjunction with cataract surgery to potentially reduce the rate of retinal tears and detachment.
Fig. 27.1 Diagram of the eye showing the relationship of the vitreous to the other intraocular structures.
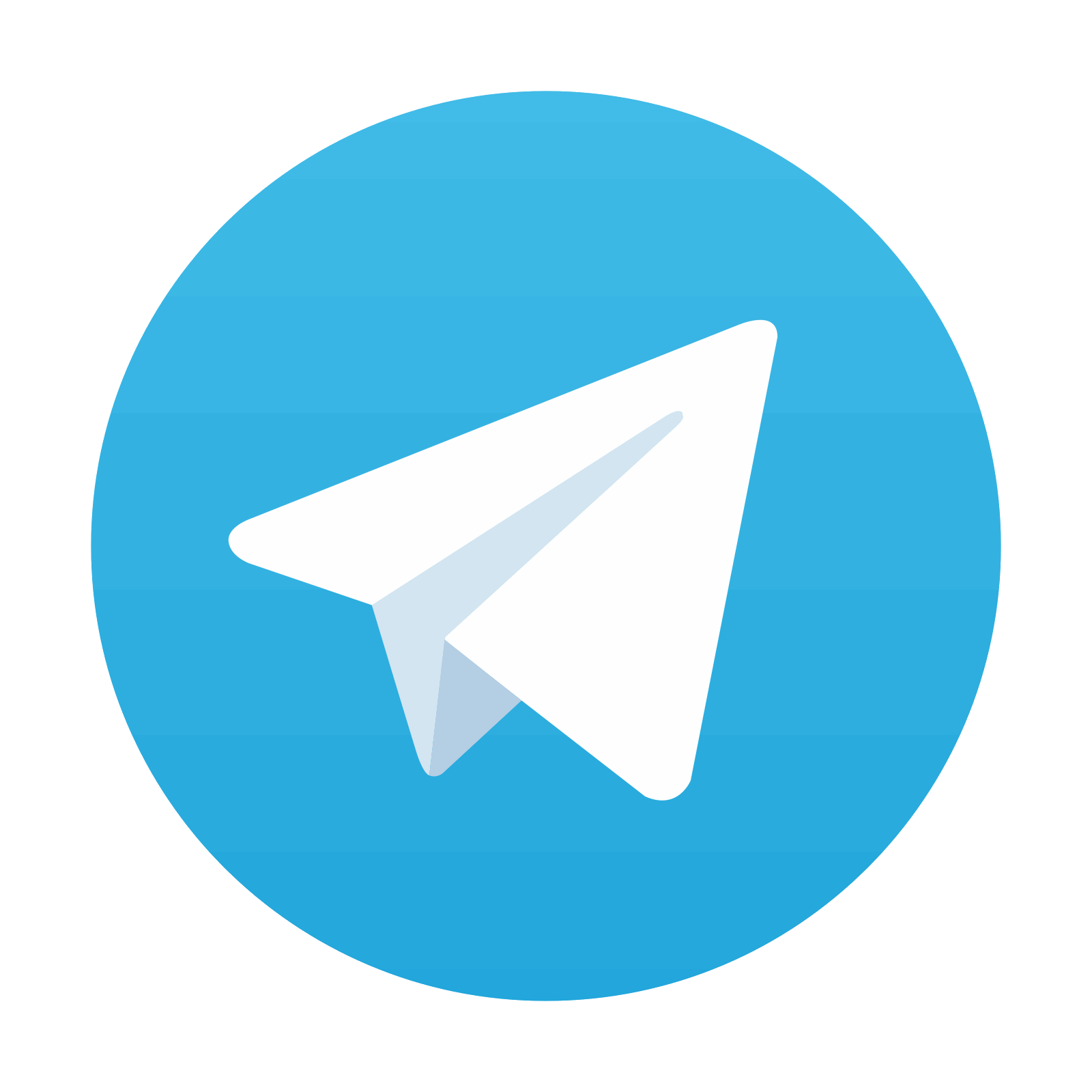
Stay updated, free articles. Join our Telegram channel

Full access? Get Clinical Tree
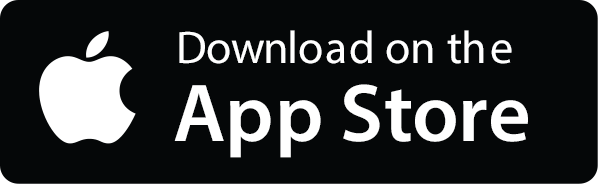
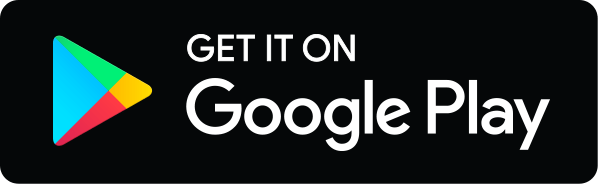