© Springer International Publishing AG 2017
Prem S. Subramanian (ed.)Ophthalmology in Extreme EnvironmentsEssentials in Ophthalmology10.1007/978-3-319-57600-8_22. The Effects of Extreme Altitude on the Eye and Vision
(1)
COL(R), US Army, Cooper Landing, AK, USA
(2)
Ophthalmology Division, The University of Tennessee Graduate School of Medicine, Knoxville, TN, USA
(3)
Department of Surgery, Knoxville, TN, USA
(4)
Coastal Eye Associates, Webster, TX, USA
Keywords
AltitudeEyeVisionCorneaRetinaIn this chapter, we will review the effects of altitude on the human visual system. In the interest of clarity, we will organize our review based on the anatomic region of the eye and its contribution to visual change during altitude exposure. We will describe the ocular anatomic and physiologic changes associated with altitude exposure and focus on the impact of these changes on visual acuity. When possible, in order to present some historical prospective, we will also include a brief history of how and when these altitude-related visual changes became known. We will also cite examples of how individual patients have been impacted by altitude-related visual changes.
The Cornea
In the normal cornea, constant functioning of an oxygen- and energy-dependent pump in the corneal endothelial monolayer is necessary to maintain corneal deturgescence and clarity. The corneal oxygen supply derives from ambient air, while the nutrients are supplied by the aqueous humor. If this pump is deficient, swelling of the corneal stroma occurs first but does not result in significant opacity. With further endothelial dysfunction, the edema spreads anteriorly into the corneal epithelium, causing profound vision loss and even pain. It is well documented that corneal stromal swelling is an expected physiologic response to high-altitude exposure and occurs as a direct result of hypoxia. A 1996 study done on subjects with normal corneas at 4270 m on Pikes Peak in Colorado described a statistically significant increase in peripheral corneal thickness after 72 h of altitude exposure and a reversal to normal 1 week after return to sea level [32]. In 2007, Morris described a significant increase in central corneal thickness (CCT ) in subjects exposed to 5200 m in Bolivia [36]. In 2010 Bosch documented an increase in CCT during a high-altitude ascent to 6300 m in Western China [6]. Specifically, in one group of climbers, the mean CCT increased from 534 to 563 μm, and decreased oxygen saturation was found to parallel the increase in CCT. Their findings suggested that, although the exact cause of the corneal swelling during ascent remains controversial, deficient delivery of oxygen to the aqueous humor may have a role in determining corneal oxygen levels [6]. In a normal cornea, such changes in corneal thickness during altitude exposure are not associated with changes in visual acuity [30]. This is because corneal-derived changes in visual acuity result from either changes in the curvature of the anterior and posterior corneal surfaces or from corneal opacification [35]. Altitude-related corneal edema thickens the normal cornea uniformly, perhaps from depressed endothelial function, but does not impact corneal curvature or clarity. Thus, even though large degrees of corneal thickening may take place during altitude exposure, there is no measureable change in visual acuity in a normal cornea. This contrasts with corneas that have preexisting anatomic abnormalities that may predispose them to develop, in addition to stromal edema, epithelial edema which can then cause corneal opacification during altitude exposure. This was well demonstrated in the 1984 report of a 77-year-old male with low endothelial cell counts who suffered unilateral corneal endothelial decompensation, necessitating corneal transplant, following exposure to 3800 m [22].
Surgical manipulation of the cornea may also set the stage for visual changes during high-altitude exposure. Oxygen to the cornea is thought to be largely supplied by ambient air. As noted above, altitude-related hypoxia causes corneal edema and a uniform increase in corneal thickness but no change in vision. However, surgical alteration of the normal corneal architecture may predispose the cornea to changes in curvature during altitude exposure with resultant visual changes [3, 25–33, 53–55]. This mechanical alteration of normal corneal structure may impact the corneal response to altitude following radial keratotomy (RK), photorefractive keratectomy (PRK) , and laser-assisted in situ keratomileusis (LASIK) refractive surgery as well as corneal transplantation .
Effects of Keratorefractive Surgery
RK was performed on millions of active myopic patients during the 1980s and 1990s. The target audience for this procedure was outdoor-oriented young people, to include high-altitude trekkers and mountaineers, who wanted to enjoy the outdoors without the need for glasses. The procedure usually consisted of four to eight radial corneal incisions in the peripheral cornea made at 90% depth with a diamond knife, sparing the central 3–4 mm. This procedure caused a steepening of the peripheral cornea and central flattening, thus decreasing myopia and improving distant vision. Although some degree of diurnal visual fluctuation occurred following this procedure [42], the initial sea level postoperative result was generally satisfactory. However, a very gradual hyperopic shift in refraction often occurs over years in patients after RK [42, 52]. While the surgery is rarely performed today, previously treated patients remain active in outdoor activities and are now aging, making them perhaps even more vulnerable to the adverse effects that altitude may have on their surgically altered corneas.
Following the initial success of the procedure, RK corneas were later found to undergo mechanical changes during altitude exposure leading to changes in vision. Such visual chang es have been well documented in the medical literature [9, 28–30, 32, 33, 49] and were also publicized in the book Into Thin Air [24] and later mentioned in the movie Everest. In one of the early cases, a hyperopic shift and corneal flattening were reported in an RK patient at an altitude of 9000 ft in Aspen, Colorado. The authors hypothesized that the direct mechanical effects of hypobaria were responsible for these changes [49]. Later research, performed in the 1990s, strongly suggested that hypoxia-induced corneal changes were a more likely etiology. Specifically, in 1995, four corneas that had undergone RK were compared to four controls after an overnight stay at two different altitudes in Bolivia [28]. The average increase in cycloplegic refraction spherical equivalent from sea level to 3657 m was +1.03 ± 0.16D and from sea level to 5183 m was +1.94 ± 0.26 D. This study suggested that the RK cornea appears to adjust constantly to changing environmental oxygen concentration, producing a new refractive error over a period of 24 h or more [28]. In contrast, an altitude chamber study in which post-RK subjects were exposed to a simulated altitude of 3660 m for 6 h demonstrated no change in refraction or corneal shape [38]. The extended time to produce visual acuity and corneal contour changes strongly suggests a metabolic origin as opposed to a purely mechanical, pressure-related, phenomenon . This theory was supported by data showing the effect of hypoxia on vision at sea level [63]. Forty subjects (20 post-RK and 20 controls) were fitted with airtight goggles such that one eye was exposed to anoxic conditions (humidified nitrogen, 0% oxygen), and the other was exposed to humidified room air. There was a hyperopic shift of +1.24 D and a +1.19 D change in corneal flattening in RK eyes after only 2 h of exposure to pure nitrogen. RK eyes exposed to humidified room air had no changes in refraction or corneal contour as did the normal eyes exposed to either nitrogen or room air. Both RK and non-RK eyes exposed to pure nitrogen had a significant increase in corneal thickness. All corneas in this study remained clear. These studies all support the notion that the RK visual changes documented during altitude exposure result from the effects of decreased oxygen partial pressure on corneal metabolism and not from the direct mechanical effect of decreased atmospheric pressure . Furthermore, a 2013 study noted that although significant corneal stromal edema was documented in 14 healthy subjects after exposure to 4559 m, there was no change in anterior chamber geometry [59]. This information suggests that the anterior segment anatomy is stable during altitude exposure, and the visual anomalies following RK and other refractive procedures occur solely as a result of corneal changes.
RK alters corneal biomechanics in a distinct manner that, in combination with hypoxic stromal swelling, leads to the characteristic hyperopic shift that has been described. The normal corneal stroma is composed of a meshwork of collagen fibrils extending from limbus to limbus. The collagen fibrils are oriented at various angles to one another in the horizontal plane. This orientation of fibrils contributes to maximum corneal strength within the corneal stroma [28]. Radial keratotomy incisions sever more fibrils that run generally perpendicular to the length of the radial incisions and leave most radially oriented fibrils intact [28]. Since stromal collagen does not completely regenerate after RK, a permanent decrease in corneal strength is predicted [42]. The preservation of radially oriented fibrils and permanent weakening of the circumferential oriented fibrils may lead to an increase in circumferential corneal elasticity. Therefore, as the corneal stroma expands with hypoxia, it remains clear [30], but there is a preferential elevation of the peripheral cornea with central cornea flattening and a resultant hyperopic (farsighted) shift in refraction. Altitude-induced corneal edema and concurrent corneal flattening usually occur only after >24 h at altitude and are not associated with acute exposure [28, 32]. Lid closure during sleep may add to the corneal hypoxia and exacerbate the visual changes [28]. Therefore the visual deterioration in an RK subject may become more evident following a night’s sleep at altitude. This may lead to a situation where a high-altitude traveler with RK may wake up in the morning having to cope with an unpredictable change in near and far visual acuity [28, 29, 31].
The impact of these RK-related refractive changes on vision at high altitude appears to be partially dependent on the subject’s age. A younger individual has greater accommodative reserve than an older subject and may better compensate for the hyperopic change at altitude. For example, a 20-year-old RK subject was documented to have a +3D shift in cycloplegic refraction from sea level to 5300 m with no subjective visual complaints [27]. In contrast, we documented a 46-year-old RK subject with crisp 20/20 uncorrected distant vision at sea level who experienced an overnight hyperopic shift at 5150 m that resulted in 20/50 distant vision [28]. He also suffered marked near vision loss that left him unable to read his watch, assemble a cook stove, or perform other near vision tasks without assistance [28].
Another important variable in predicting visual disability in RK patients with high-altitude exposure is the postoperative refractive error . An RK climber with residual myopia at sea level may actually enjoy an improvement in distant visual acuity with moderate altitude exposure but note decreasing vision with further exposure to higher altitudes. For example, an RK subject in his 40s with mild myopia at sea level noted an improvement in uncorrected distant vision in Fairplay, Colorado (elevation 3000 m) because his hyperopic shift at this altitude effectively negated his myopia [53]. However, this same individual experienced a decrement in distant vision at 6000 m on a climb in South America and required +1.50D glacier glasses to complete his climb (personal communication, Larry White MD). Neal Biedelman, a 38-year-old climber with a post-RK sea level refraction of −1.00D, safely ascended Mt. Everest without visual difficulty [27]. His lack of difficulty at extreme altitude was likely due to his baseline myopia and his pre-presbyopic accommodative reserve. In contrast, a 47-year-old RK climber with excellent uncorrected vision at sea level also ascended Mt. Everest but experienced poor near vision and some blurring at distance [33]. Finally, at age 50, Beck Weathers had excellent visual acuity at sea level but experienced a profound loss of vision while attempting to climb Mt. Everest in 1996. Unfortunately, Dr. Weathers did not have a dilated fundus examination after his climb, so it is possible that retinal pathology may also have impacted his visual change [27].
The unique corneal structural changes associated with PRK, LASIK, and corneal transplantation may set the stage for a narrower spectrum of visual changes during high-altitude exposure as compared to RK. Since the late 1990s, PRK and LASIK have largely replaced RK for the surgical correction of myopia and can correct hyperopia and astigmatism as well. PRK reshapes the anterior corneal surface using an excimer laser and does not affect directly the deeper stroma. The Pikes Peak study of 1996 examined the cycloplegic refraction, keratometry, computed video keratography, and central and peripheral corneal thickness in six subjects (12 eyes) who had undergone PRK, six subjects with RK (11 eyes), and nine myopic controls (17 eyes) during 3 consecutive days at 4300 m [32]. Pachymetry measurements demonstrated a significant peripheral corneal thickening in all three groups with a return to normal at sea level. As expected, the RK subjects experienced a significant, progressive, and reversible hyperopic shift in refraction. Subjects who had PRK and those with myopia had no change in refraction [32]. It was hypothesized that although hypoxia caused corneal edema in the PRK corneas, this corneal expansion was uniform across the area of the PRK, and the shape of the anterior corneal surface remained unchanged. Thus, the visual acuity in PRK patients was stable and even appeared suitable for aviators [25]. It is interesting to note that in 2008 an astronaut with bilateral PRKs completed a 12-day Russian Soyuz mission to the International Space Station. Although changing environmental conditions of launch, microgravity exposure, and reentry created an extremely dynamic ocular environment, he reported no visual anomalies [15]. PRK is no longer considered disqualifying for flight personnel in all three military services as well as NASA.
Several studies have examined the stability of post-LASIK surgery corneas during exposure to hypoxia [3, 10, 11, 55]. In the LASIK procedure, a thin flap is created in the cornea and elevated such that the excimer laser can reshape the underlying corneal stroma. The flap is then replaced. Nelson and colleagues used goggles to create an anoxic environment for post-LASIK and normal control eyes and documented symmetric corneal thickening in both LASIK and control subjects as well as a small myopic shift in LASIK corneas [37]. There was a trend toward corneal steepening by keratometry in LASIK subjects, but this was not statistically significant. Such a small myopic shift in an extreme, anoxic environment suggested that LASIK eyes should be relatively stable at high altitude. Several case reports have described variable responses to altitude exposure. White and Boes described LASIK climbers with decreased distant vision at altitudes of 5947 m and 6961 m, respectively [3, 55]. In contrast one LASIK climber noted no visual change during a climb to 4850 m [10]. Subsequently, Dimmig and Tabin followed six climbers (12 eyes) during a 2002 ascent of Mt. Everest [11]. All had 20/20 vision or better at sea level and maintained this visual acuity at the 5380 m basecamp. One climber reported mild blurring with ascent above basecamp that improved with descent and two other climbers reported blurred vision at 8180 and 8635 m that also improved with descent. Five of the six climbers reported no visual changes up to 8000 m. Three climbers summited the peak (8848 m) with no visual complaints. In summary, these reports suggest that LASIK appears to be a reasonable alternative to glasses, contact lenses, or RK for high-altitude exposure. LASIK is also no longer disqualifying for flight personnel in all three branches of the military and NASA.
Corneal Transplantation
Until about 10 years ago, most corneal transplantation involved the full-thickness trephination and removal of the central portion of a diseased cornea and its replacement with donor graft tissue. Invariably, eyes with such transplants stabilize with about a 50% reduction in the number of viable endothelial cells. Although changes in graft astigmatism and corresponding visual changes are common in the months following a transplant, the vision gradually stabilizes following removal of sutures. A 2001 case report described a successful corneal transplant patient who had stable post-op vision of 20/30 OD with a refraction of plano +2.75 × 030 with all sutures removed approximately 1-year post-corneal transplant for Fuchs endothelial dystrophy [23]. The subject lived at an altitude of 210 m; however, following a 3.5 month stay at 2800 m, the patient’s distant vision gradually declined to 20/200 with her spectacles. Manifest refraction was now −6.25 + 3.50 × 055 and best-corrected vision remained 20/30. Five weeks after she returned to her home altitude, she noted an improvement in uncorrected vision and a partial reversal of her myopic shift with a refraction of −3.25 + 2.25 × 050. The authors hypothesized that the relative hypoxia at 2800 m may have caused localized endothelial cell dysfunction and increased circumferential hydration along the graft-host junction. This may have caused an expansion in the peripheral graft with a concomitant central steepening as demonstrated by the rather prominent myopic shift. This case report serves to further illustrate that several varieties of incisional corneal surgeries may predispose patients to visual changes during high-altitude exposure.
More recently, it has become technically feasible to transplant only the stroma in cases of corneal stromal disease and only the endothelium in cases of isolated endothelial dysfunction, although some eyes still require full-thickness transplantation. In general, any patient who has had endothelial transplantation may be at risk of variable refractive status or even corneal opacification when exposed to hypobaric hypoxia .
The Iris and Ciliary Body
While no study has implicated actual dysfunction of the iris at high altitude, abnormalities of pupil dynamics have been described [61]. Transient reduction in percentage change of pupil size, increase in latency, and reduction in speed of contraction were found in climbers reaching 4770 m. These changes were attributed to cerebral effects of hypoxia, but a direct effect on the iris was not considered.
The Trabecular Meshwork
The trabecular meshwork is the primary regulator of intraocular pressure (IOP), which has been investigated extensively in climbers. No study has found glaucoma or other abnormalities of IOP control at high altitude. However, one study found that subjects with higher baseline IOP were at increased risk to develop high-altitude retinal hemorrhages (HARH) later (see below) [7].
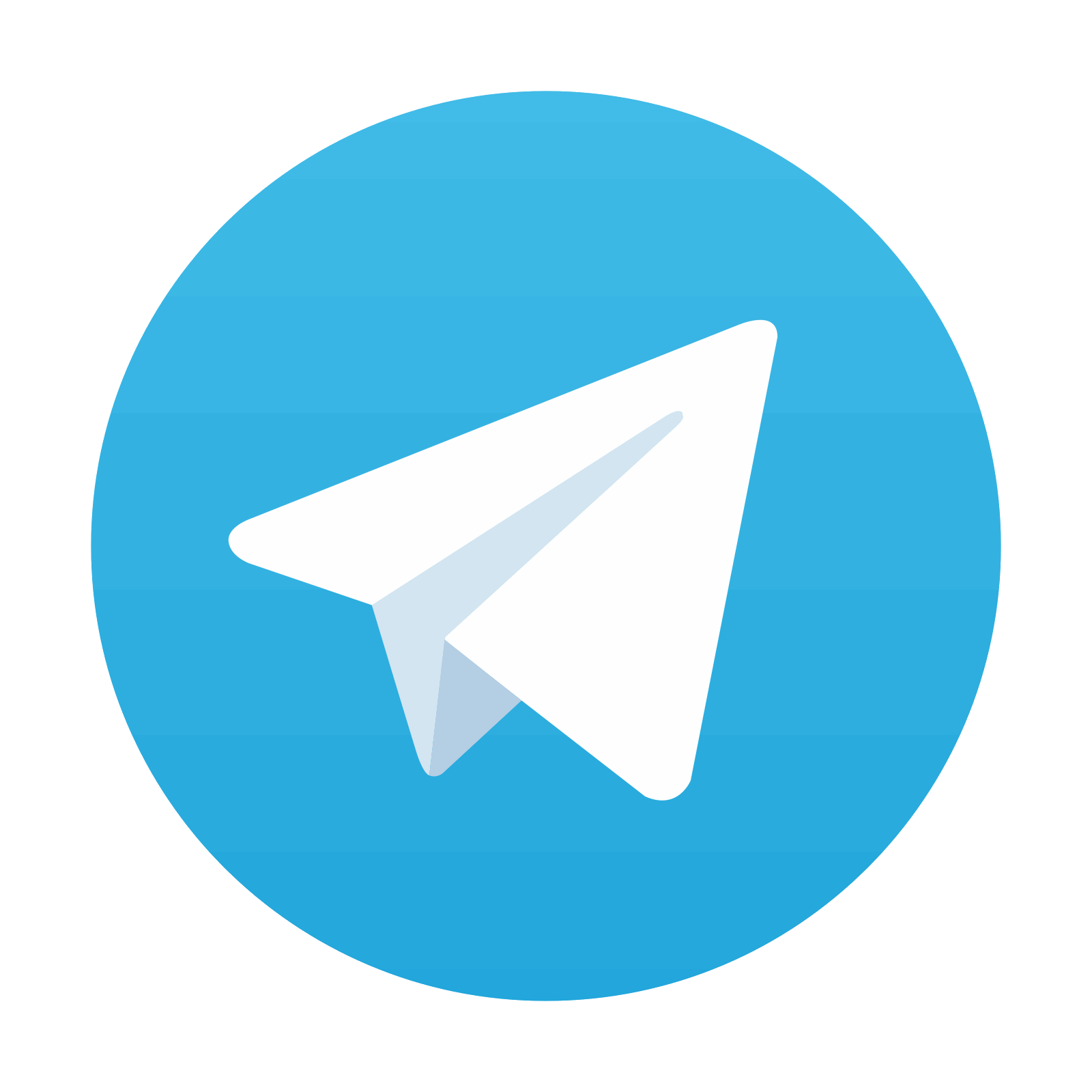
Stay updated, free articles. Join our Telegram channel

Full access? Get Clinical Tree
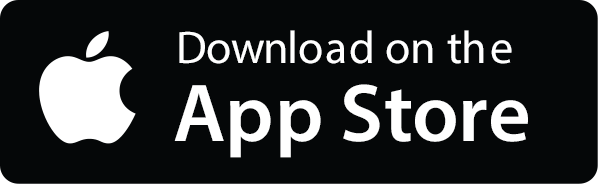
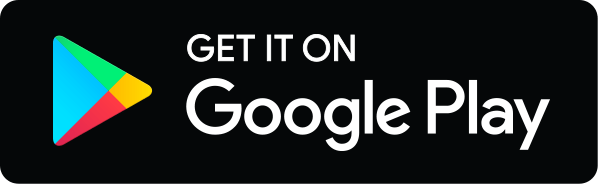