Purpose
To investigate whether long-term protection from light exposure affects the rate of disease progression in patients with autosomal recessive Stargardt disease (STGD1), measured using fundus autofluorescence imaging.
Design
Longitudinal, retrospective, interventional case series.
Methods
Five patients with Stargardt disease protected 1 eye from light exposure by applying a black contact lens during waking hours for ≥12 months. Disease progression was followed by performing autofluorescence imaging at semi-regular intervals. Longitudinal changes in autofluorescence were studied by evaluating areas of decreased autofluorescence and areas of increased autofluorescence as a measure of retinal pigment epithelium damage and lipofuscin accumulation, respectively.
Results
We observed less progression of decreased autofluorescence in 4 out of 5 light-protected eyes relative to their respective nonprotected eyes. The progression of increased autofluorescence, on the other hand, was highly variable and did not respond consistently to treatment.
Conclusions
Areas of decreased autofluorescence may serve as a useful biomarker for measuring the progression of Stargardt disease. The reduced progression of decreased autofluorescence in the light-protected eyes suggests that light deprivation might be beneficial in patients with Stargardt disease.
Autosomal recessive Stargardt disease (STGD1) is the most common inherited juvenile macular degeneration. Most patients develop bilateral loss of vision in childhood or early adulthood. This subtype of Stargardt disease is caused by mutations in the ABCA4 gene, which encodes a retina-specific transporter protein (ABCR) in the rims of rod and cone photoreceptor outer segment discs. Retinal degeneration in ABCA4 -linked Stargardt disease is believed to result from the toxic effects of lipofuscin that accumulates in the retinal pigment epithelium (RPE) and the subsequent degeneration of photoreceptors.
Light can induce photochemical injury at the ocular fundus. Depending on the level and duration of the irradiance, the primary site of damage can be either the photoreceptors or the RPE. In ABCA4 -linked retinopathies, products generated by the visual cycle accumulate and contribute to retinal damage via both direct toxic effects and increased photosensitivity. A major fluorophore of lipofuscin, bis -retinoid N -retinylidene- N -retinyl-ethanolamine (A2E), accumulates with other, currently unidentified lipofuscin constituents within the RPE. Thus, an excessive accumulation of A2E has been observed in both Abca4 -/- mice and patients with Stargardt disease. Lipofuscins (and A2E in particular) are potent photosensitizers that can induce oxidative damage, thereby accelerating light-induced retinal damage and RPE atrophy. This oxidative damage may affect the rate of disease progression in patients with Stargardt disease.
The total quantity of A2E oxiranes in Abca4 -/- mice increases in response to light exposure. In addition, a recent review of light-induced and inherited retinal degenerations suggested that light exposure might modify the disease course of genetically well-defined retinal dystrophies, including Stargardt disease. An early study used functional and ophthalmoscopic examinations to test the effect of 5 years of unilateral light deprivation in a patient with autosomal recessive retinitis pigmentosa and in a patient with autosomal dominant retinitis pigmentosa; the author observed symmetrical disease progression. However, in the absence of a genetic diagnosis, and without clear insight into the pathogenesis of these 2 RP patients, no conclusions can be drawn with respect to patients with Stargardt disease.
Because the aforementioned results suggest that patients with Stargardt disease might be more sensitive to light, we considered using light protection as a means to slow disease progression in patients with Stargardt disease. Therefore, we retrospectively examined the effects of using a single black contact lens in an attempt to spare disease progression in 1 eye in patients with Stargardt disease. We followed disease progression in 5 patients using the fundus autofluorescence imaging data, as this test provides a sensitive, noninvasive measure of RPE abnormalities and lipofuscin accumulation in patients with Stargardt disease.
Methods
This study was a longitudinal, retrospective, interventional case series of patients diagnosed with Stargardt disease. The intervention was applied in the context of clinical care at the request of the patients (or legal guardian) and not in the context of a prospective clinical trial. Therefore, nonstandard analyses (eg, routine imaging) were performed to ensure internal quality control and to detect any adverse events in a timely manner. All patients had been diagnosed previously as having Stargardt disease with at least 1 ABCA4 mutation, and all patients presented with the typical clinical symptoms associated with this retinal dystrophy.
All genetic analyses were performed by the Department of Human Genetics at Radboudumc (Nijmegen, the Netherlands). Patients were screened for known ABCA4 mutations using the arrayed primer extension microarray (Asper Biotech, Tartu, Estonia), and exon duplications and/or deletions were detected using multiplex ligation-dependent probe amplification (P151 and P152; MRC-Holland, Amsterdam, the Netherlands). If no mutations—or only a single heterozygous mutation—were identified, the exons and intron-exon boundaries were sequenced using the Sanger method to screen for mutations in the other allele. All identified mutations were confirmed using Sanger sequencing.
The study was performed in accordance with the tenets established by the Declaration of Helsinki, and informed consent was obtained from all patients (or from the legal guardians of underage patients) for a retrospective analysis of therapy-related data. Patients were previously advised regarding the potential benefits of wearing sunglasses, avoiding direct light exposure, and limiting their dietary intake of vitamin A. Complete protection from light exposure was suggested as a treatment option to patients who had a pressing request for any potentially effective treatment, given the current absence of suitable treatments for Stargardt disease. The institutional review board (Commissie Mensgebonden Onderzoek, Region Arnhem-Nijmegen) retrospectively approved this study (2013/062) after the last patient’s final visit and during the study.
Patients were enrolled in the study and followed from January 2006 through December 2009 at the clinical practice of the Department of Ophthalmology, Radboudumc, Nijmegen. After eliminating any medical concerns regarding the use of contact lenses, we suggested that the patients protect their intuitively best eye (determined at the time of enrollment) during waking hours for at least 1 year using a black contact lens. The age of disease onset was defined as the age at which visual loss was first recorded. Best-corrected visual acuity (BCVA) was measured using a Snellen chart or the finger-counting method. To provide the most complete light protection, a customized, black, soft contact lens designed to cover the entire cornea (Ercon, Assen, the Netherlands) was applied; this lens blocked >90% of light in the visible spectrum.
Patients underwent a routine ophthalmologic examination, including BCVA, slit-lamp examination, binocular funduscopy, and confocal fundus autofluorescence imaging of both eyes with a Spectralis device (Heidelberg Engineering, Heidelberg, Germany). After pharmacologic pupil dilation to ≥6 mm with phenylephrine 2.5% and tropicamide 0.5% eye drops, we recorded fundus autofluorescence images centered on the fovea and including part of the optic disc; images were obtained using an excitation wavelength of 488 nm and a barrier filter (≥500 nm) in high-resolution mode (30-degree field of view, 1536 pixels × 1536 pixels). Baseline and follow-up images of both eyes were captured with equal system sensitivity settings. Clinical phenotyping was performed in accordance with Fishman and associates (1999) by an experienced clinician (author R.H.) who was masked with respect to the study results. Fundus autofluorescence was used to phenotype the disease stages as described by Cideciyan and associates.
Fundus autofluorescence images were acquired using a standard procedure as follows. First, focusing was performed using the near-infrared reflection mode of the Spectralis. To account for chromatic aberrations, slight refocusing in the autofluorescence mode was performed, and 9 separate images were acquired after sensitivity adjustment, thereby covering the entire macular area and including part of the optic disc. To increase the signal-to-noise ratio, all images were aligned and a mean image was calculated using the internal software (without normalization by histogram stretch). All other image processing and analysis was performed using scripts written in MatLab (R2006; MathWorks, Natick, Massachusetts, USA). Initial and final fundus autofluorescence images were automatically registered as reported previously. The alignment of each image pair was manually confirmed; if necessary, registration was adjusted iteratively until a satisfactory result was obtained. Pixel size was normalized to the fovea-disc margin distance.
Increased autofluorescence and/or decreased autofluorescence relative to the image background was quantified by an observer (author M.L.; this author was masked with respect to the treated eye) based on non-normalized mean images as published previously. In brief, each fundus autofluorescence image was leveled by subtracting a 12-zone quadratic polynomial mathematical model of the image’s background autofluorescence, which was calculated for each image. The threshold for increased or decreased autofluorescence was set at 1.5 × σ above or below, respectively, the mean pixel intensity of the resulting image. Consistent with previous reports, we found that this threshold provided the best detection of visually evident autofluorescence abnormalities. In some cases, the precise location of the fovea could not be determined owing to pathologic changes; in such cases, determining where to place the center of the quadratic polynomial model was questionable. Therefore, we adjusted the model’s position slightly until the selection of any visually evident autofluorescence abnormalities was optimized. For some images, multiple repositioning of the model was required in order to capture all autofluorescence abnormalities; in these cases, we combined the resulting pixels showing increased and/or decreased autofluorescence, respectively. Pixels that were identified incorrectly in vessels and/or bifurcations were removed manually.
The total number of pixels showing increased and/or decreased autofluorescence was measured in each image, and the annual rate of change in decreased autofluorescence (change in decreased AF) in serial image pairs of the right eye (OD) was calculated as follows:
% Change in decreased AF ( OD ) / yr = ( Decreased AF post ( OD ) − Decreased AF pre ( OD ) Decreased AF pre ( OD ) + Decreased AF pre ( OS ) ) . 100 % . [ Time between images ( yr ) ] − 1 .
The annual rate of change in the left eye (OS) was calculated as follows:
% Change in decreased AF ( OS ) / yr = ( Decreased AF post ( OS ) − Decreased AF pre ( OS ) Decreased AF pre ( OD ) + Decreased AF pre ( OS ) ) . 100 % . [ Time between images ( yr ) ] − 1 .
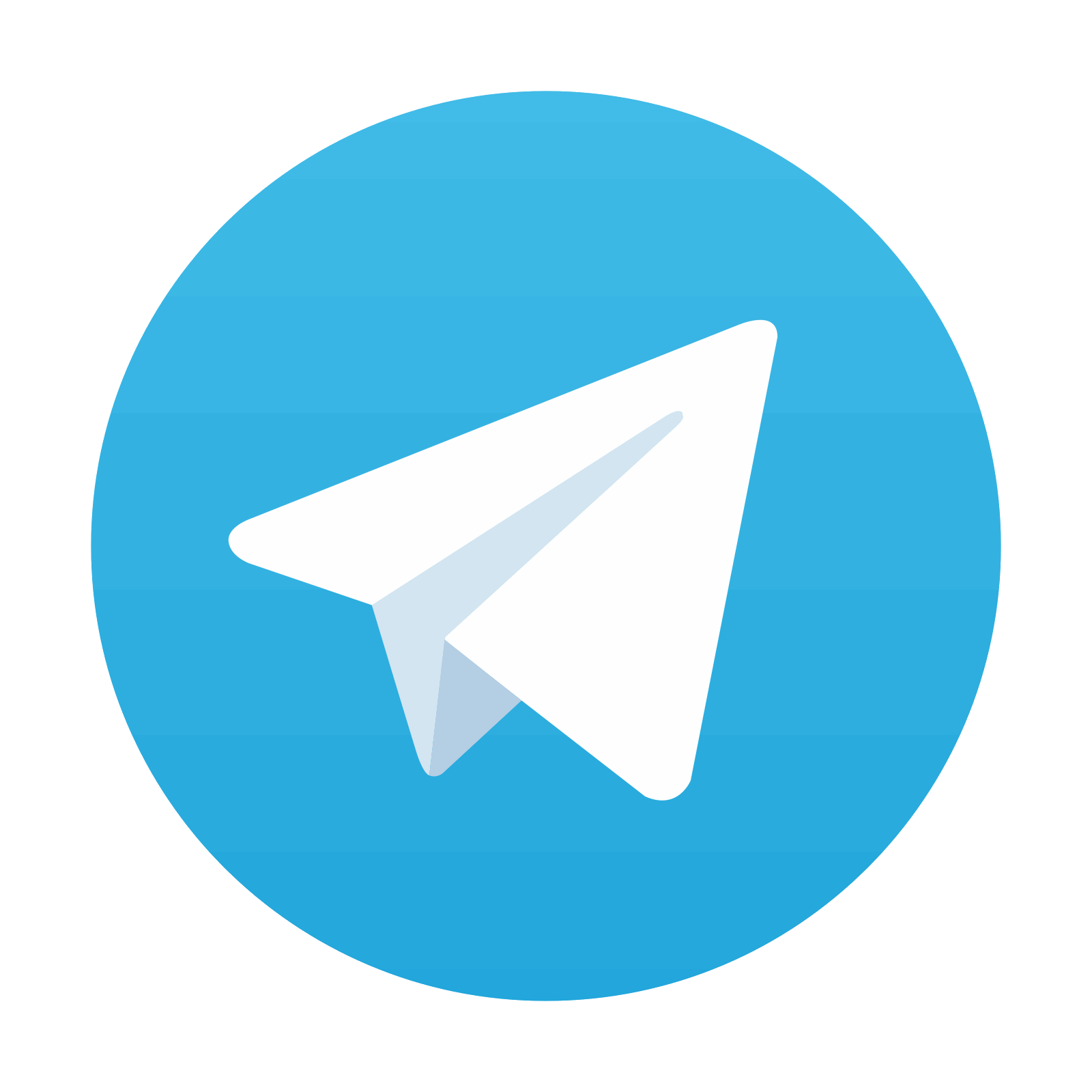
Stay updated, free articles. Join our Telegram channel

Full access? Get Clinical Tree
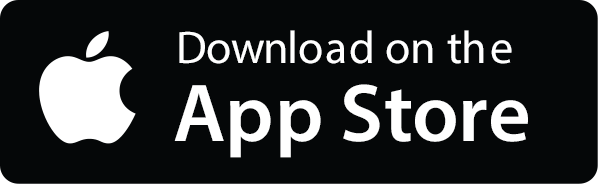
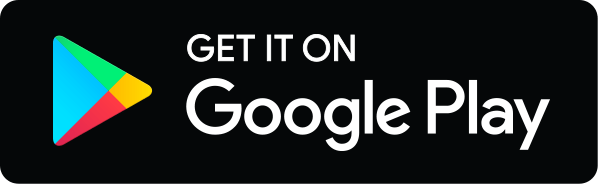