The Conjunctiva–Structure and Function
Darlene A. Dartt
INTRODUCTION
The conjunctiva is a thin, semitransparent mucous membrane that covers the posterior surface of the eyelids and is then reflected onto the eyeball, where it extends to the limbus of the cornea. The conjunctiva takes its name from the fact that it conjoins the eyelids with the eyeball. This junction is indirect, with the conjunctiva forming a fornix on three sides of the globe and an extendible plica on the fourth side. Such an arrangement allows the globe and the eyelids to move independently of each other. When viewed by light microscopy, the conjunctiva is revealed as a nonkeratinizing squamous epithelium containing mucin-secreting goblet cells. This epithelium overlies a loose connective tissue, the substantia propria, which is highly vascularized, contains afferent, sensory, and efferent (sympathetic and parasympathetic) innervation, and is well endowed with lymphoid tissue. Not only does the conjunctiva function to enable independent motion of the globe and eyelids, it also protects the cornea and hence the interior of the eye from the external environment by secreting mucins, antibacterial proteins, electrolytes, and water to form the inner mucous layer of the tear film and perhaps a portion of the aqueous layer. Without these normal quantitative and qualitative conjunctival secretions, a variety of mucous-deficiency diseases develop that in the worse possible case lead to extensive damage to the cornea and a sight-threatening condition.
THE TEAR FILM
The tear film is composed of three layers: The innermost mucous layer is secreted by the conjunctiva; the middle aqueous layer is secreted by the main lacrimal gland and accessory lacrimal glands; and the outer lipid layer is secreted by the meibomian glands (Fig. 1). Between the mucous layer and the corneal and conjunctival epithelia there is a mucin-containing glycocalyx that extends from the apical membrane of the superficial cells of these epithelia, which acts as an interface between the ocular surface cells and the tears (Fig. 2). The lipid, aqueous, and mucous layers have been measured to be 0.1 μm, 7 to 10 μm, and 0.2 to 1.0 μm thick, respectively.1 Measurements by Prydal et al2 indicated a far thicker mucous layer of about 30 μm and an aqueous layer of 10 to 11 μm; however, it is thought that these measurements included a portion of the epithelium, and they have not been reproduced. In fact, recent studies again found a thinner tear film and a pre-lens tear film layer that averaged 2.7 μm.3,4 One hypothesis about the structure of the tear film is that the mucous and aqueous layers are not distinct, but rather are a gradient of decreasing mucous and increasing aqueous content from the ocular surface to the lipid layer. Another suggests that the lipid layer is a monolayer consisting of two phases: a polar phase adjacent to the aqueous layer, and a nonpolar phase at the tear film–air interface.5 Additional experiments are required to resolve this controversy.
Since the many exocrine glands surrounding the orbit (the ocular adnexa) and the ocular surface epithelia secrete the tear film, it is a necessarily a complicated fluid. The functions of the tear film are varied and vital to vision. Tears are the first refractive surface encountered by light rays, and therefore they must remain clear to form a refractive surface that will allow light to reach the retina. The transparency of the cornea, the second refractive surface that light encounters, is maintained by the tears. Another crucial role of tears is to protect the ocular surface and to maintain its health and normal function. The tear film protects the ocular surface from the external environment by responding to wide-ranging exterior conditions and potential threats to the eye. The environmental stresses placed on the eye are a result of desiccation, bright light, extreme thermal conditions, physical injury, and noxious chemicals, and infection by bacteria, viruses, and parasites. Tears also lubricate the surface of the eye to avoid mechanical damage from the high pressures generated by the blink. Tears transport oxygen and provide a limited number of other nutrients to the avascular cornea. They also contain proteins and other molecules that are involved in the defense of the ocular surface and regulate cellular functions of the conjunctiva and cornea. To meet the needs of the cornea and conjunctiva, and to respond to changes in the external environment, the volume, composition (including the electrolyte composition, osmolality, protein composition, and pH), and structure of the tears are extraordinarily well regulated.
Three factors control the volume and composition of the tear film: control of secretion by the lacrimal and accessory glands, the cornea, and the conjunctiva; changes in the evaporation of tears; and regulation of tear drainage. The control of secretion and absorption of tears by the conjunctiva and meibomian glands is covered in the present chapter, and the control of lacrimal and accessory lacrimal gland secretion and tear drainage is discussed in another chapter,”The Lacrimal Gland.”
ANATOMY OF THE CONJUNCTIVA
The conjunctiva starts at the posterior third of the meibomian (also known as the tarsal) gland openings and along the palpebral margin of each eyelid (Fig. 3). In this area, the cells change from the keratinized stratified squamous epithelium of the eyelid to the nonkeratinized stratified squamous epithelium of the marginal conjunctiva. This forms a mucocutaneous junction that is covered with lipids secreted by the meibomian glands. The lipids restrict the tears behind the openings of the glands, resulting in a division of the eyelid margin into an anterior, dry, keratinized part and a posterior, wet, nonkeratinized conjunctival portion. The tarsal conjunctiva is continually covered with tears, and it is this area that the lacrimal puncta open to connect the conjunctival sac with the nose (Fig. 4). The conjunctiva then passes over the eyelid margin, forming a right angle to the tarsi forming the superior and inferior fornix, which allows for a sweeping mechanism to clean the bulbar conjunctiva and the cornea. From the fornix, the conjunctiva proceeds to the eyeball, forming the anterior portion. The conjunctiva ends at the corneal limbus. This arrangement forms a mucous membrane-lined sac, with the eye positioned in the posterior part of the sac. The palpebral fissure is the only opening to the external environment.
![]() Fig. 3. Schematic representation of the anatomy of the conjunctiva. (Reprinted from Lemp MA: The Dry Eye: A Comprehensive Guide. Heidelberg, Germany: Springer Verlag, 1992.) |
For descriptive purposes the conjunctiva may be divided into three major subdivisions (as shown in Fig. 3):
Tarsal, or palpebral, lining the eyelids
Forniceal, lining the upper and lower the fornices
Bulbar, overlaying the sclera on the anterior portion of the globe
Approximately 2 mm from the eyelid on the tarsal conjunctiva lies a shallow (less than 1 mm deep) groove called the subtarsal groove. This groove runs parallel to the eyelid margin for most of the length of the tarsal conjunctiva. The groove marks the area of transition from the nonkeratinized, stratified squamous epithelium of the eyelid margin to the cuboidal epithelium of the tarsal conjunctiva (Fig. 3). The groove provides an ideal anatomical trap for small foreign objects before they can reach the cornea and bulbar conjunctiva. The objects trapped in the subtarsal groove are almost immediately covered with a thick coating of mucus and are moved medially by the blink to be carried out on, and trapped by, the hairs of the caruncle. There are multiple smaller ridges and grooves between the eyelid margin and the tarsal groove that contain a series of small saccular mucus glands. Each of these glands has a tubular lumen 15 to 30 μm in diameter that opens onto the conjunctival surface. These glands are formed by an invagination of the conjunctival epithelium and are lined with mucus-secreting epithelial cells.6
The nasal tarsal conjunctiva contains numerous structures known as Henle’s mucus crypts. These crypts change in the midtarsal conjunctiva to form a network of subepithelial grooves. The grooves become tunnels with multiple interconnections that have small openings onto the ocular surface. The grooves and tunnels divide the surface of the conjunctiva into irregular papillae averaging approximately 100 μm in diameter (Fig. 5). At the orbital margin of the tarsus, the tunnel system opens onto the surface to form a network of grooves that divide the surface into bulges averaging 300 μm in diameter. In the fornix, these grooves and clefts are called Stieda’s cleft and plateau system. These structures are generally larger and more prominent on the temporal side,7 and are usually more prominent in the upper eyelid than in the lower fornix. The patterns made by these clefts and grooves differ greatly between the conjunctivas of different individuals, as well as between the eyes of one individual. Local or systemic disease processes may result in hypertrophy of the underlying lymphoid layer, with follicle formation and profound alterations in the surface features of the conjunctiva. The conjunctival papillae that are normally present may also hypertrophy in response to infectious or toxic agents, and thus further modify the surface topography.7
This complex groove, crypt, and gland system covering the tarsal and forniceal conjunctivas provides an efficient trap system whereby small foreign bodies, bacteria, and cellular debris are sequestered, coated with mucus, and neutralized. These conjunctival surface folds greatly increase the total surface area and at the same time decrease the area of contact between the bulbar and palpebral conjunctiva, thereby reducing friction force as the two surfaces glide over one another during blinking and rotation of the globe.
The bulbar conjunctiva is smoother than the palpebral conjunctiva and its surface features are less consistent. There are often four to six low broad papillae about the corneoscleral limbus that range in size from barely visible irregularities to the hypertrophic follicles that are occasionally seen in vernal conjunctivitis. The palisades of Vogt are structures around the limbus that radiate outward from the cornea. These structures consist of a series of 1.5- to 2-mm ridges and are formed by the epithelial rete ridges and the underlying stromal condensations. One of their functions is to trap small foreign objects that were on the cornea and were subsequently dislocated by blinking. Often the palisades are outlined by a fine brown line that radiates from the cornea. This is caused by the production of pigment from an increase in the local melanocyte population, which makes these structures easy to identify.
The basal conjunctival epithelial cells are attached by desmosomes to a basement membrane. The tarsal conjunctiva is firmly attached to the tarsi and requires careful dissection. The upper tarsus is usually more tightly adhered than the lower tarsus. Meibomian glands are visible in both the upper and lower eyelids as yellow streaks running perpendicular to the eyelid margin. The conjunctiva of the fornix forms an almost continuous sac that is interrupted medially by the plica semilunaris. From the fornices the conjunctiva sweeps posteriorly to attach to and cover the eyeball, approximately 10 mm posterior to the limbus superiorly, 12 to 14 mm posterior to the limbus laterally, and 8 to 10 mm from the limbus inferiorly. Medially it variably covers the globe for approximately 7 mm posterior to the limbus.
Four fornices are formed by the conjunctiva where it is reflected from the eyelids to the globe. The superior fornix is the largest in both size and volume. It is formed and maintained by fine smooth muscle slips that pass from the levator palpebral superioris muscle and insert into the conjunctiva. This attachment prevents the conjunctiva from folding downward over the cornea. It also prevents the fornix from developing sags as the globe moves upward, which might obstruct vision. The temporal fornix is attached by fine fibrous slips to the tendon of the lateral rectus muscle, again maintaining the relative position of the fornix during horizontal movements of the globe. The inferior fornix is attached to the tendon of the inferior rectus, which prevents its movement. The plica semilunaris performs the same function as a fornix medially, and is a reversed fornix with the fold of conjunctiva lying externally (Fig. 3). During a medial gaze, the fornix has a variable depth. This occurs because the fibrous slips that link the conjunctiva to the tendon of the medial rectus muscle insert onto the deep surface of the plica and caruncle. Contraction of the medial rectus tightens these slips, forming a cul-de-sac medially as the globe adducts. On maximal medial rotation, the plica partially unfolds to form a true fornix similar to that present in other areas.
The conjunctival epithelium changes from cuboidal shaped at the anterior surface to nonkeratinized-stratified squamous epithelium 2 to 3 mm from the corneal limbus. It is in this area, where the conjunctival stratified squamous epithelium becomes continuous with the corneal epithelium, that the corneal epithelial stem cells reside. It is well known that if the corneal epithelial cells are removed but the limbus remains intact, the cornea will re-epithelize and remain clear. If the limbus and its stem cells are lost, the cornea will vascularize.8 In addition, goblet cells can be observed migrating onto the corneal surface with the new epithelial cells after the corneal epithelium, including the limbus, is completely removed. As the new epithelium matures and becomes isotopic with normal corneal epithelium, the goblet cells sometimes disappear. It is not known what processes the goblet cells use to migrate, or what effects they have on the corneal epithelium.
While it is well established that the corneal stem cells are located in the corneal limbus, the site of the stem cells in the conjunctival epithelium is controversial. Clonal cultures of human conjunctival epithelial cells suggest that stem cells are dispersed throughout the epithelium.9 This has been substantiated by studies in mice containing green fluorescent protein in the conjunctival epithelial cells.10 Because the amount of this protein varies between cells, these studies were able to track specific cells and monitor their mitotic activity. The results indicated that the epithelial cells of the bulbar conjunctiva are mitotically active and are generally immobile in the lateral direction, which suggests that these cells are self-sufficient. Other investigators observed that label-retaining cells had a uniform distribution, which implies that stem cells are uniformly distributed in the bulbar conjunctiva.9 Still other studies have suggested that there are stem cells in the conjunctival fornix, as well as at the mucocutaneous junction.
CONJUNCTIVAL MICROSTRUCTURE
The nonkeratinized conjunctival epithelium consists of several layers of stratified squamous cells that do not substantially vary over the different regions of the conjunctiva.11 The often-reported variation in the number of cell layers is thought to be the result of variable stretching of the elastic conjunctiva.11 The basal layer of the epithelium undulates along with the rippling of the underlying substantia propria. Scattered within the epithelium of the conjunctival surface are numerous mucin-secreting goblet cells and mucous glands (Fig. 6). Human conjunctiva contain intraepithelial clusters of cells known as the glands of Manz and the crypts of Henle.12 Goblet cells are not evenly distributed throughout the conjunctiva, and range from 1,000 to 56,000 per square millimeter of conjunctival surface depending on the presence or absence of inflammation and the area studied.13 The fornices contain more goblet cells per unit area than the tarsal and bulbar conjunctivas. The inferior conjunctiva contains more goblet cells compared to the superior conjunctiva, and the nasal conjunctiva contains more than the temporal conjunctiva.14
The apical cells of the conjunctival epithelium are not flattened like those of the cornea. However, like the apical squamous cells of the cornea, the stratified squamous cells have a complicated arrangement of microvilli and microplicae on the apical-most membranes.15,16 The microvilli are 0.5 μm in diameter and 0.5 to 1 μm high. The microplicae are of a similar size, measuring 0.5 μm in width, 1 to 3 μm in length, and 0.5 μm in height. This system of outfoldings is present over the entire conjunctival surface, and is continued over the apical membrane of the goblet cells. In these cells the apical cell membrane disintegrates during secretion and is released into the tear film along with the secretory granule membranes and their components. After the secretory process is completed, the goblet cell resynthesizes mucin by packaging it into secretory granules that are separated from the tear film by the apical membrane. This complex system of micro-outfoldings of the surface cell membranes may aid in supporting, stabilizing, and anchoring the tear film against the effects of gravity and eyelid movement, thereby preventing irregular streams and flow patterns from developing that would interfere with clear vision. In addition, membrane-spanning mucins are inserted into the microplicae of the apical membrane to form the glycocalyx. Notable in the stratified squamous cells of the conjunctiva are numerous clear vesicles in the cytoplasm, which give the cells a lacy appearance. These vesicles contain mucin that is perhaps being transported to the apical membranes.
The total surface area of an adult conjunctival sac in one eye, including the cornea, averages 16 cm2, or roughly 17 times more surface area than the cornea. Because of its large surface area, the conjunctiva may be a significant source of electrolytes and water in the tear film.17 The blood vessels and the epithelial cells of the conjunctiva may transport fluid across the conjunctiva. An increase in vascular permeability of the blood vessels may contribute fluid to tears by leaking plasma. This may occur in pathologic conditions, such as inflammation or topical application of drugs, that increase vascular permeability. Epithelial cells can also be a source of fluid for tears. Under normal conditions, the goblet cells and the stratified squamous cells are the primary sources of fluid transport in the conjunctiva. The conjunctiva can also absorb electrolytes and water.18,19 In addition, the conjunctiva absorbs drugs that are applied to the ocular surface.20 The conjunctiva is permeable to hydrophobic molecules up to 40 kDa21 and actively transports drugs using nucleoside, monocarboxylate, dipeptide, and sodium-dependent transporters.22,23,24 Conjunctival epithelial cells can also absorb compounds by endocytosis.25 For a comprehensive review of conjunctival fluid secretion, see Dartt.26
The conjunctival epithelium is a low-resistance, permeable epithelium, particularly in contrast to the high-resistance, impermeable corneal epithelium. The permeability of the conjunctival epithelium arises from the attachment of the stratified squamous and goblet epithelial cells to each other by tight junctions and desmosomes. Furthermore, the plasma membranes of adjoining cells do not totally interdigitate, and thus leave large intercellular spaces. These intercellular spaces provide antibodies, other plasma constituents, and inflammatory cells from the abundant underlying conjunctival capillaries ready access to the surface of the conjunctiva, where irritative and infectious processes arise. This pathway may operate in the reverse direction, such that infectious agents on the conjunctival surface may gain access to the circulatory system. Proteins applied topically to the conjunctiva percolate easily down to and into the lumen of the conjunctival capillaries, and appear shortly thereafter in the systemic circulation.27 Certain topically applied ophthalmic medications may accumulate intra- or extracellularly within the conjunctival epithelium, resulting in discoloration or an accumulation of deposits that may be mistaken for various disease processes. An example of this process is the accumulation of brownish black deposits that are often encountered clinically after topically applied epinephrine is used as an antiglaucomatous medication.28
INNERVATION OF THE CONJUNCTIVA
The external portion of the visual system–the eyebrows, eyelids, conjunctiva, and cornea–is richly endowed with sensory nerve endings. This abundant and highly integrated sensory system is necessary for the protection, coordination, and general well-being of the eyeball and the aforementioned periorbital structures.
The conjunctiva has a rich sensory innervation, but because it lacks muscular structures and innervated glands, no motor nerves are present. Autonomic fibers are present surrounding the blood vessels, and may on occasion supply twigs to scattered and inconsistently present smooth muscle fibers in the plica semilunaris. The sensory nerve supply to the conjunctiva is derived from the first or ophthalmic branch of the trigeminal nerve. This sensory system is closely associated with that of the eyelids, eyebrows, and cornea. The same main functional classes of sensory afferents that have been identified and well-studied in the cornea also innervate the bulbar conjunctiva.29,30 Because the corneal and conjunctival nerves are similar, and because both arise from the trigeminal ganglion, only the characteristics of the corneal nerves are described here.
Ocular surface nerves can be classified as thin myelinated (A-δ type) or unmyelinated (C type). These two types of nerves differ in that the former conduct nerve impulses faster than the latter. They also differ in their passive and active electrophysiological properties. All of these peripheral axons lose their myelin sheath when they enter the stroma. They then branch extensively, forming a subepithelial plexus.29 Thin branches derived from this plexus ascend up into the basal layer of the epithelium, where they run parallel to the surface and form leashes that terminate in the apical layers of the epithelium. It is of functional importance that the sensory nerves do not surround the goblet cells but are restricted to the stratified squamous cells. Morphologically, the sensory nerves appear homogeneous: approximately 58% of the sensory nerves contain the neuropeptide CGRP in their nerve endings and axonal fibers, and about 20% contain Substance P.31 Electrophysiologically, these nerves are very different and can be divided into four categories:
Mechanoreceptor (low threshold)
Mechano-nociceptor (high threshold)
Polymodal nociceptor
Cold receptor
About 20% of the sensory nerves are mechanoreceptor and mechano-nociceptor, and respond to mechanical forces that are similar to those required to damage the cornea. These receptors are likely responsible for the acute, sharp sensation of pain that occurs with mechanical contact with the cornea. The majority of sensory nerves (about 70%) are polymodal nociceptors that are activated by mechanical energy, heat, exogenous chemical irritants, and endogenous chemical mediators released by damaged tissue, resident inflammatory cells, or plasma leaking from blood vessels.29 Most of these nerves are of the C type, and they signal both the presence of the stimulus and its intensity and duration. These nerves contribute to the sharp pain that occurs when the cornea is mechanically stimulated, as well as to the pain caused by chemical irritation, heat, cold, or inflammation. The final category of nerves, which comprises 10% to 15% of the sensory nerves, consists of cold-sensitive receptors that contain both A-δ and C fibers. The cold-sensitive nerves fire continuously, but increase their rate of firing as the temperature of the surface decreases because of evaporation, application of cold solutions, or blowing of cold air.
The parent axons of trigeminal ganglion nerves branch extensively when they enter the stroma, and cover an area of tissue surface called a “receptive field.”29 The functional receptive fields of the mechano-nociceptors and polymodal nociceptors are large. In contrast, the cold receptors have small receptive fields.
Tissue injury and inflammation modify the activity of sensory nerves. An important feature of ocular-surface polymodal nociceptor nerves is that when a noxious stimulus is repeated within a short time, the impulse-firing threshold decreases and the mean firing frequency in response to a given stimulus increases. Often a low spontaneous firing activity develops. These characteristics are called “sensitization.” Sensitization develops because tissue injury from the noxious stimulus releases endogenous inflammatory mediators from damaged cells and neighboring resident inflammatory cells activated by the injury. These inflammatory mediators cause changes in the ion channels that are responsible for the electrical activity of the nerve. Prostaglandin and bradykinin are two inflammatory compounds know to cause sensitization of corneal nerves. Another consequence of an injurious stimulus is that the stimulus causes the nerve endings of the polymodal nociceptors to release their neuropeptide content as a consequence of membrane depolarization and antidromic (opposite direction) conduction of the nerve impulse. The released sensory neuropeptides, CRRP and Substance P, produce vasodilation and plasma extravasation, and stimulate cytokine release, leading to a local inflammatory response known as neurogenic inflammation. Although the noxious stimulus may be limited to a restricted area, the excited endings produce impulses that propagate centripetally and antidromically stimulate other, noninjured branches of the parent axon. Neuropeptides are subsequently released from nerve endings that were not originally injured. This antidromic stimulation appears to be the origin of the extension to noninjured conjunctiva of the neurogenic inflammation that follows a limited corneal or conjunctiva injury.
The development of the gas esthesiometer by Belmonte et al32 has enabled researchers to study the application of controlled mechanical impulses, irritant chemical stimuli, and hot or cold air pulses separately in a limited area of the conjunctiva, and to measure the psychophysical characteristics for each type of stimulus. In one study the same responses were elicited from human conjunctiva and cornea, except that the overall sensitivity was comparatively lower in the conjunctiva and the light stimuli were not felt as irritating.30 The sensations produced by heat and suprathreshold mechanical or chemical stimulation always included a component of irritation, although each sensation was identified as being distinct from the others.
Other types of nerves that innervate the conjunctiva include the efferent sympathetic and parasympathetic nerves.12,33,34 These unmyelinated nerves send free nerve endings into blood vessels and epithelium around goblet cells and between stratified squamous cells.12 External stimuli activate the different types of sensory nerves in both the cornea and the conjunctiva. However, one study found that the polymodal nociceptors in the cornea are the primary nerves that stimulate reflex tear production.35 In that study, activation of the corneal mechanoreceptors and cold receptors was less effective, and activation of the conjunctival sensory receptors did not induce tear secretion. When corneal sensory receptors are activated, they use a local neural reflex conducted in the dromic direction to stimulate the parasympathetic and sympathetic nerves in the conjunctiva to release their neurotransmitters (Fig. 7). These neurotransmitters may then activate conjunctival stratified squamous cells to increase electrolyte and water transport, and goblet cells to secrete mucins.36
CONJUNCTIVAL EPITHELIAL CELL ELECTOLYTE AND WATER TRANSPORT
Under basal conditions, fluid secretion from conjunctival epithelial cells into tears prevails over absorption to account for the volume of the tear film.37,38 Studies on fluid secretion from the conjunctiva have employed a mixed population of both stratified squamous cells and goblet cells. Ion transporters, which are necessary for electrolyte and water secretion, have been localized to both of these cell types. Thus, both must be considered as secreting electrolytes and water. The movement of two ions (Cl–and Na+) drives conjunctival epithelial fluid transport (Fig. 8). Under short-circuit conditions, Cl–secretion (in a basolateral to apical movement) accounts for about 80% of the ion transport, while Na+ absorption (apical to basolateral) accounts for about 20% of the ion transport measured.39,40 Because the Na+/glucose transporter SGLUT1, NKCC, and Na+, K+-ATPase are all located on conjunctival stratified squamous cells and goblet cells, it appears that the same cell type is both secretory and absorptive. There are no conjunctival cells that have only SGLUT1 and not NKCC or Na+, K+-ATPase. This is unique to the conjunctiva.41,42
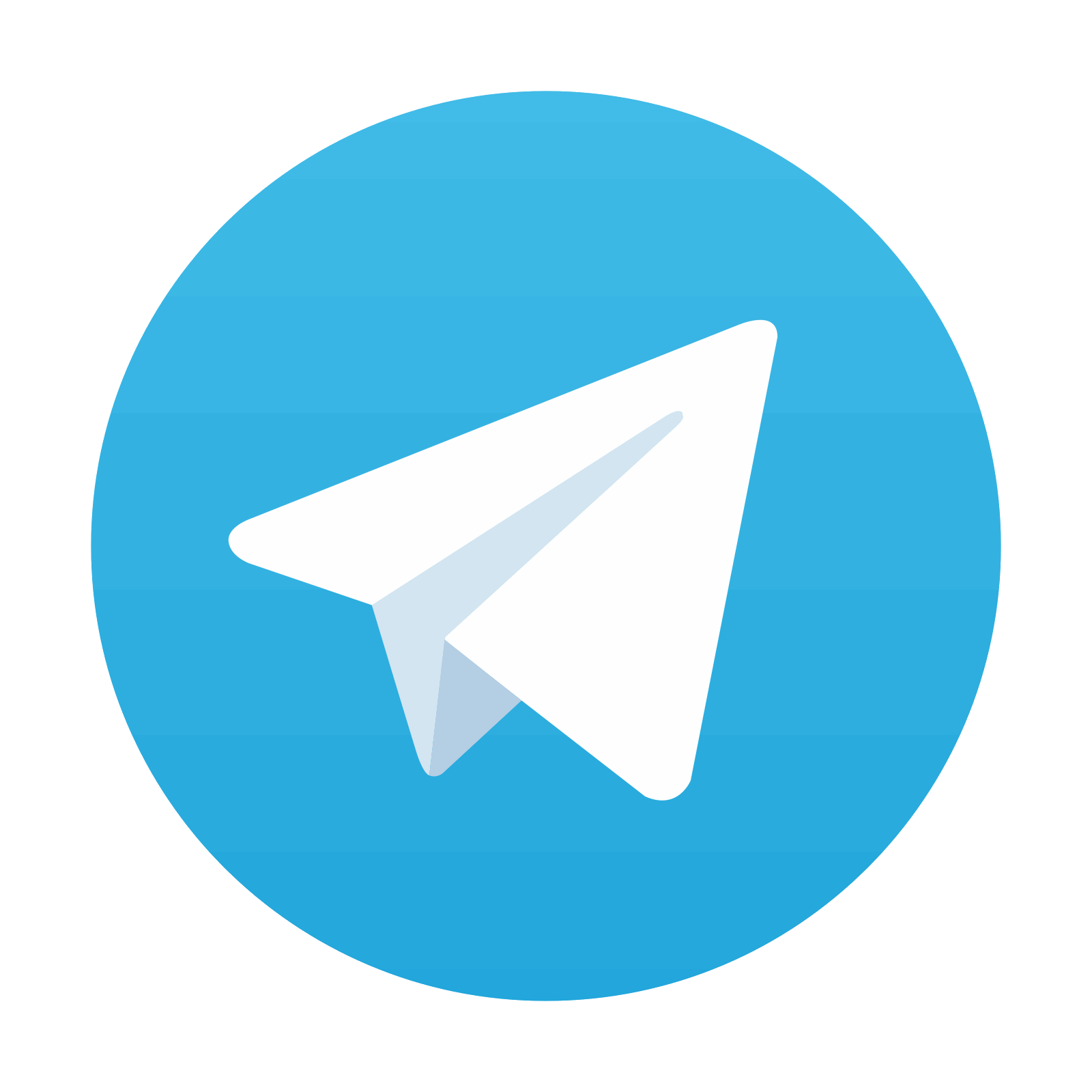
Stay updated, free articles. Join our Telegram channel

Full access? Get Clinical Tree
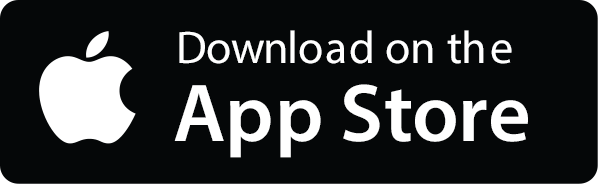
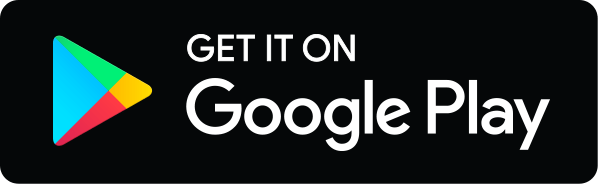