The Biology of Corneal Refractive Surgery
Steven E. Wilson
Marcelo Netto
Renato Ambrósio Jr.
The results of corneal refractive surgical procedures are influenced by the biologic responses to the surgical injury, including corneal wound healing and biomechanics. Intensive research has provided a relatively complete understanding of the healing response, complications associated with wound healing and tissue changes, and the possibility of using pharmacologic agents to modulate the response to corneal refractive surgery. Understanding the biology of the cornea and the interactions between its cellular components allows for better appreciation of anomalous results and complications that may occur after laser in situ keratomileusis (LASIK), photorefractive keratectomy (PRK), and other refractive surgical procedures. In the absence of such knowledge, it is possible to attribute these outcomes incorrectly to external factors.
This chapter outlines our understanding of the relevant cells and structures that are involved in corneal wound healing and biomechanics. In addition, the biology of specific corneal refractive surgical procedures is described, along with the biologic mechanisms that underlie surgical outcomes and complications.
CORNEAL STRUCTURES INVOLVED IN WOUND HEALING AFTER REFRACTIVE SURGERY
The systems that orchestrate the response to refractive surgical procedures evolved to maintain and restore vision after mechanical injury and infection. The cellular elements of the cornea that participate in responses to refractive surgical procedures include the epithelium, stroma, and nerves (1,2). The epithelium is stratified squamous. Epithelial stem cells are thought to reside in the limbus (3). The normal stroma is populated by keratocytes. These cells differ in density from the anterior to the posterior stroma, with the density being higher in the anterior stoma (4,5). Keratocytes may differ in differentiated characteristics from the anterior to the posterior and the center to the peripheral stroma.
The endothelium may participate in some types of injuries, such as deep incisions or laser burns. Inflammatory cells from the immune system also contribute to the response to all injuries (6, 7, 8, 9).
Structural elements include the basement membrane of the epithelium, Bowman’s layer (in humans and some other species), corneal lamellae, proteoglycans, and other extracellular matrix components. Bowman’s layer is the acellular anterior layer of the stroma that lies below the epithelial basement membrane. Bowman’s layer has a different arrangement of collagen fibers compared with deeper layers of the stroma.
The cornea-sensory nerve-central nervous system-motor nerve-lacrimal gland-tear film axis also participates in the corneal wound healing response (10). Tears contain many components that modulate the epithelial and stromal wound healing responses, including cytokines and growth factors (11, 12, 13).
CORNEAL WOUND HEALING
The corneal wound healing response comprises a complex cascade of processes involving the corneal epithelium, stroma, nerves, inflammatory cells, lacrimal gland, tears, and their components. The overall response is similar after various types of injuries, but important variations occur in different surgical procedures that may affect refractive outcomes and complications.
The earliest corneal event noted after traumatic or surgical injury to the corneal epithelium is keratocyte apoptosis (7,14,15) (Fig. 67-1). The disappearance of keratocytes after epithelial injury was first noted by Dohlman and coworkers (16) and rediscovered several times by other investigators. Many years passed before it became clear that the disappearance of keratocytes was mediated by apoptosis or programmed cell death, occurring in every species examined to date, including rabbits, rats, mice, chickens, monkeys, and humans (15,17).
The initial keratocyte apoptosis response to epithelial injury is surprisingly rapid. If the epithelium of a freshly enucleated eye is scraped with a scalpel and the eye is then immersed into an electron microscopy fixative, cellular changes characteristic of apoptosis can be seen by transmission electron microscopy (15). The terminal deoxyribonucleotidyl transferase-mediated dUTP-digoxigenin nick and labeling (TUNEL) assay for fragmented DNA characteristic of apoptosis peaks at approximately 4 hours after epithelial injury, when nuclear DNA degradation has peaked (7,15) (Fig. 67-1). TUNEL-positive stromal cells can be detected for approximately 1 week after PRK or LASIK (7,18). Transmission electron microscopy demonstrates that almost all cells dying in the stroma at 4 hours after epithelial injury are undergoing apoptosis, with cell shrinkage, chromatin condensation, and formation of apoptotic bodies (7,14,15) (Fig. 67-1). At time points later than 4 hours, an increasing proportion of dying cells in the stroma undergo necrosis (7). These cells include keratocytes, wound healing fibroblasts, myofibroblasts, and inflammatory cells.
Localization of the keratocyte undergoing apoptosis varies with the surgical procedure (Fig. 67-1). For example, with PRK and laser subepithelial keratomileusis (LASEK), it occurs in the anterior subepithelial stroma. In uncomplicated LASIK, it occurs just anterior and posterior to the lamellar interface in the deeper stroma. After a LASIK buttonhole flap, it occurs along the superficial lamellar interface and in the anterior stromal region where the blade perforates the epithelium. With incisional procedures it is noted along the depth of the stromal incision (Wilson SE, unpublished data, 1995). Reports of stromal cell death after collagen shrinkage procedures, corneal rings, or stromal inlays are lacking. The localization of the early apoptosis response may be important in determining the localization of later events in the wound healing process (7). The location of the stromal wound healing response relative to the epithelium is probably a critical determinant of differences in regression, haze, and other complications between PRK and LASIK (7).
The mechanism through which epithelial injury induces keratocyte apoptosis remains controversial. Many studies have demonstrated that cytokines expressed by the corneal epithelial cells trigger keratocyte apoptosis in vitro and after in vivo microinjection. For example, interleukin (IL)-1 is expressed at high levels in the corneal epithelium and can be released only through death of, or injury to, the epithelial cells (Fig. 67-2). Microinjection of mouse IL-1α into the quiescent central stromal of the mouse cornea induces keratocyte apoptosis (15). Many other cytokines produced by the corneal epithelium can trigger keratocyte apoptosis. These include tumor necrosis factor-α (TNF-α), Fas ligand, and bone morphogenic proteins 2 and 4 (19, 20, 21, 22). Importantly, the effect of a cytokine such as IL-1 depends on the concentration of the cytokine and the milieu of the cell. Thus, IL-1 may trigger apoptosis in a quiescent keratocyte in the normal stoma, whereas it upregulates metalloproteinase production and secretion of keratinocyte growth factor (KGF) in a myofibroblast during wound healing. A possible mechanism of keratocyte apoptosis thus would be active stimulation of cell death by the epithelium or by epithelial-derived factors (23,24).
A potential mechanism through which keratocyte apoptosis may be mediated is autocrine suicide. Keratocytes constitutively produce the cell death receptor Fas, but not the Fas ligand in the unwounded cornea (22). Fas ligand is expressed in corneal epithelium (22). However, Fas ligand is a membrane-associated cytokine and requires cell-cell (juxtacrine) contact between the cells for induction of apoptosis. This contact does not appear to be the predominant mechanism of keratocyte apoptosis. However, when corneal fibroblasts in culture are exposed to IL-1, they begin expressing Fas ligand (19). This induction of Fas ligand could trigger keratocyte cell death through autocrine suicide because the keratocytes would be producing the Fas ligand and receptor. This mechanism has been found to function in several other cells (19).
Zhao and coworkers (25) suggested that the tears contain the factors that trigger keratocyte apoptosis. Keratocyte apoptosis, however, still occurs in the enucleated eye washed free of tears, and many tear factors are themselves derived from the epithelium (26). Intentional introduction of corneal epithelium beneath a LASIK flap increases keratocyte apoptosis (23). In addition, an epithelial defect is not required for induction of keratocyte apoptosis. Viral infection of intact epithelium (24) or even pressure on the cornea with a cloning cylinder (27) induces keratocyte apoptosis underlying the site of epithelial injury. So although tear factors derived from corneal or lacrimal gland epithelium could contribute to induction of keratocyte apoptosis, they do not appear to be the only factors involved in mediating this process.
Epithelial healing starts almost immediately after epithelial injury. It is regulated by autocrine cytokine networks in the epithelial cells; cytokines produced by stromal cells like keratocytes and wound healing fibroblasts (2,11,13,28, 29, 30); and lacrimal gland-derived factors secreted into tears (11,13,28). Early after surface ablation procedures like PRK, the anterior stroma is devoid of cells (15). Tear cytokines derived from the lacrimal glands probably regulate early corneal epithelial cell proliferation and migration. The stromal cells probably become the most important regulators of epithelial healing as this layer is repopulated with keratocytes, corneal fibroblasts, and myofibroblasts. Important cytokines likely include hepatocyte growth factor (HGF) and KGF produced by the stromal cells and lacrimal gland (11,13,30). HGF and KGF are classic mediators of stromal-epithelial interactions. They are produced by the stromal cells and regulate proliferation, migration, and differentiation of the receptor-bearing epithelial cells. Autocrine, paracrine, and juxtacrine (requiring cell-cell contact) epidermal growth factor, transforming growth factor (TGF)-α, and TGF-β are also likely among the complex array of cytokines that modulate corneal epithelial proliferation, migration, and differentiation during corneal wound healing (31). Healing of the epithelium can be accompanied by epithelial hyperplasia that can persist indefinitely.
Inflammatory cells invade the corneal stroma as early as 8 to 12 hours after epithelial injury (7,8). These cells include monocytes (Fig. 67-3), granulocytes, T cells, and other inflammatory cells (32) (Wilson SE, Huang J, Possin D, et al., unpublished data, 2002). These cells function to eliminate microorganisms and debris produced by the injury and wound healing response. They persist for up to several weeks after PRK and LASIK (7).
Inflammatory cells are drawn into the cornea by chemokines such as monocyte chemotactic and activating factor (MCAF) and granulocyte colony-stimulating factor produced keratocytes in response to cytokines like IL-1 and TNF-α released from injured corneal epithelial cells (6,9). Other chemokines are derived from epithelial cells and possibly other sources.
Beginning 12 to 24 hours after epithelial injury, proliferation of residual keratocytes can be detected in the stroma by immunocytochemistry for the mitosis-specific antigen Ki67 (7,33). In PRK and LASEK, this proliferation occurs in a band of peripheral and posterior stroma surrounding the zone of earlier keratocyte apoptosis (7,33) (Fig. 67-4A). In LASIK, it occurs surrounding the lamellar interface (34) (Fig. 67-4B). Currently it is thought that these proliferating keratocytes give rise to all activated keratocytes, myofibroblasts, and corneal fibroblasts that repopulate the depleted stroma. However, investigations are ongoing to determine whether infiltrating monocytes could also give rise to some of the stromal participants in the wound healing process, analogous to monocyte differentiation into osteoclasts in bone (Wilson SE, Mohan RR, unpublished data, 2002). The same cytokines that regulate the transformation from monocyte to osteoclast are also expressed in the corneal stroma. Our working hypothesis is that monocytes give rise to fibroblastic cells that participate in stromal remodeling in the cornea during wound healing (keratoclasts).
Many changes occur in the corneal stroma in the weeks after corneal injury. Most of the changes are poorly characterized at the molecular and cellular level. Stromal cells called myofibroblasts (express alpha smooth muscle actin) may appear immediately beneath the epithelium a week or two after PRK (7). These cells are presumably derived from proliferating keratocytes under the influence of TGF-β (35, 36, 37). They become most prominent approximately 1 month after PRK (Fig. 67-5). Whether they appear depends on the level of correction in PRK (7). In LASIK, myofibroblasts are usually detected only in the periphery of the cornea, where the lamellar interface intersects the epithelium (7). However, they may be detected in the central cornea along the lamellar interface if epithelial debris is retained in the intralamellar space at the time of surgery (21). Myofibroblasts are important in contraction of the wound in incisional procedures (35,36). Their function in a broad wound like PRK is not apparent, although on immunohistologic studies they produce high levels of growth factors like HGF that modulate proliferation and differentiation of the overlying epithelial cells (2,30,38). They contribute to haze (opacity) in procedures like PRK because the myofibroblasts themselves are opaque and produce disordered collagen (35,36). Recent studies have demonstrated that these cells are generated after PRK for high myopia, but not PRK for low myopia, in the rabbit (7). They are rarely seen in the central cornea in LASIK, even in LASIK for high myopia (7), in the absence of epithelial debris or inflammation. Myofibroblasts do appear on both sides of the lamellar interface if epithelial debris is retained beneath the flap in LASIK (23).
![]() FIGURE 67-4. Keratocyte proliferation at 72 hours after photorefractive keratectomy (PRK) (A) and 72 hours after laser in situ keratomileusis (LASIK) (B) in rabbits. Keratocytes undergoing mitosis were detected using immunocytochemistry by expression of the Ki-67 mitosis-specific antigen (7). Note that keratocytes undergoing mitosis are more superficial in PRK (arrows in A) than in LASIK (arrows in B). There are epithelial cells undergoing mitosis in both the eye that had PRK and the eye that had LASIK. There is epithelial hyperplasia in the eye that had PRK (original magnification ×400). (Courtesy of Audrey E. K. Hutcheon and James D. Zieske, with permission.) |
Quantitative studies in rabbits have demonstrated a correlation between the level of keratocyte apoptosis, keratocyte proliferation, and myofibroblast generation in PRK and LASIK (7). In high PRK corrections (−9 diopters of myopia), there were higher levels of all three wound healing parameters than in lower PRK corrections (−4.5 diopters). The levels of keratocyte apoptosis, keratocyte proliferation, and myofibroblast generation were still lower in LASIK, even when comparing high correction for −9 diopters of myopia in LASIK with lower correction of −4.5 diopters in PRK.
It remains to be determined whether there is a cause-and-effect relationship between early keratocyte apoptosis and later events such as keratocyte mitosis and myofibroblast generation. If pharmacologic agents that inhibit keratocyte apoptosis without triggering keratocyte necrosis can be developed, it should be possible to determine whether these relationships are casual.
At present, little information is available regarding the wound healing response after LASEK. It is likely to be similar to PRK, but further study will be needed to determine if parameters like keratocyte apoptosis, keratocyte proliferation, and myofibroblast generation are different in PRK and LASEK for the same level of correction.
One study suggested that the level of haze after PRK was determined by the volume of stromal tissue removed (39). It remains to be determined, however, whether the volume of stromal tissue or other cellular response variables that would also be increased with higher levels of correction are the more important factors.
In vivo confocal microscopy is a valuable method for monitoring the effect of PRK and LASIK on corneal nerves, overall opacity, flap thickness, and the density of stromal cells over time. It, however, cannot reliably distinguish live from dead cells, apoptosis from necrosis, or even specific cell types such as keratocyte, corneal fibroblasts, myofibroblasts, monocytes, or polymorphonuclear cells. Thus, its utility in understanding the cellular changes that contribute to the wound healing response is limited.
Epithelial hyperplasia may occur after PRK (40, 41, 42) or LASIK (43, 44, 45, 46). Epithelial hyperplasia can cause regression of the effect of correction of myopia, hyperopia, and astigmatism. It also will likely influence the custom corneal ablation with LASIK, LASEK, or PRK because the features that will be applied to correct higher-order aberrations will often be measured in microns (47).
The factors leading to epithelial hyperplasia are complex. An abrupt change in corneal contour or a rough surface can be associated with epithelial hyperplasia. However, epithelial hyperplasia can occur in corneas with a smooth surface and may occur when there is epithelial scrape injury without refractive ablation (48). In some eyes, the hyperplasia may be related to production of high levels of cytokines like HGF and KGF by keratocytes, corneal fibroblasts, or myofibroblasts. There could be genetic factors that regulate the response of the epithelium to these cytokines in a particular eye. HGF and KGF are candidate modulators of epithelial hyperplasia because these growth factors stimulate proliferation and inhibit terminal differentiation of epithelial cells (2,11,29,30).
Another late event that occurs after refractive surgical procedures is stromal remodeling. This includes deposition and resorption of disorganized collagen laid down in the stroma by keratocytes, corneal fibroblasts, or myofibroblasts (49, 50, 51). Disorganized collagen may be replaced by ordered collagen characteristic of clear corneas. Remodeling could also include changes in glysosaminoglycans in the corneal stroma (44,45,52,53). Such stromal remodeling could also be associated with regression of the effect of all refractive surgery procedures.
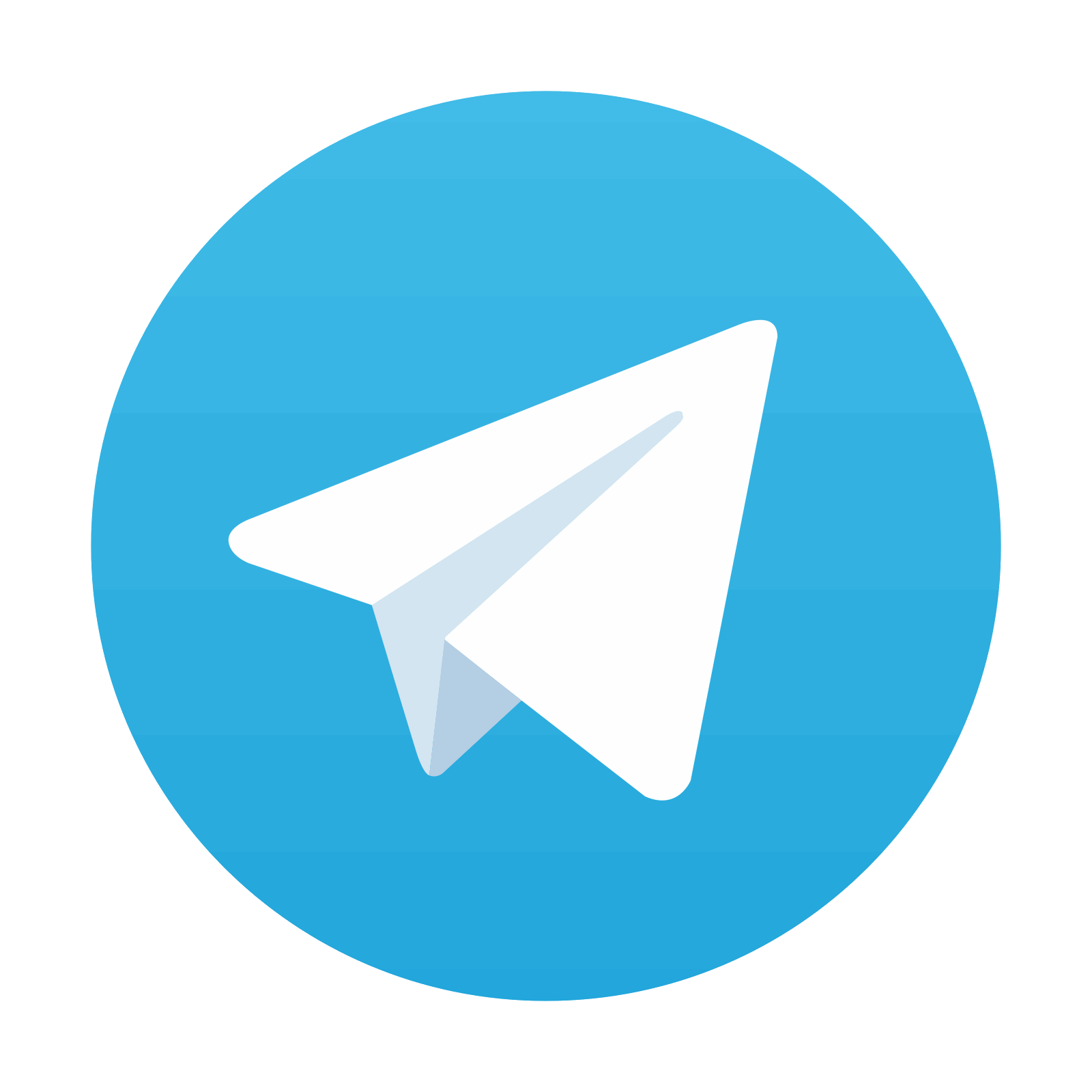
Stay updated, free articles. Join our Telegram channel

Full access? Get Clinical Tree
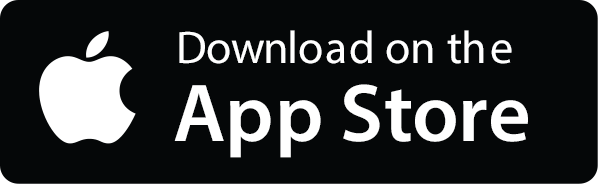
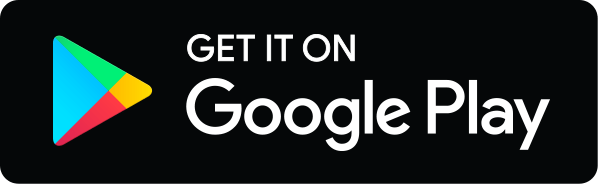