The Basic Science and Mechanism of Laser Components
Neal Whittle
Aaron B. Zimmerman
The word laser is an acronym for the words light amplification by stimulated emission of radiation.1 Each of these terms describes a laser beam’s properties and must be fully understood for one to appreciate the nature of laser ophthalmic surgery. The first term to be described is light.
Light is a form of electromagnetic radiation (EMR). EMR is energy emitted and absorbed by charged particles; in other words, it is energy emitted and absorbed by matter. EMR exhibits wave-like behavior as it travels through space. As the name suggests, EMR has both electric (E-field) and magnetic (B-field) components. The E-field of EMR is responsible for all observed optical phenomena such as reflection, refraction, diffraction, and interference. In a vacuum, EMR propagates at the speed of light.2
Light may be described either as electromagnetic (EM) waves or as the flow of particles (photons). Each of these views is described in this chapter.
LIGHT AS A WAVE
Oscillations cause waves. These oscillations propagate out from the source, inducing further oscillations as the wave moves through space or a medium. There are two types of propagating waves: transverse waves and longitudinal waves.
A longitudinal wave is a wave in which the direction of oscillation is in the same plane as the direction of travel. Longitudinal waves are also referred to as compression waves. Sound waves are longitudinal waves.
A transverse wave is one in which the oscillation is perpendicular to the direction of travel. A pebble dropped into still water produces transverse waves (ripples) that move outward in two dimensions. EMR propagates as transverse waves, meaning that light propagates as a transverse wave as well. Light waves travel very much like ripples on a pond’s surface, but light waves propagate in three dimensions. Transverse waves may be polarized. The polarization of a light wave is the orientation of the E-field of the EM wave and the direction of oscillation that produced the wave. For example, an EM wave with a vertical oscillation would also have a vertically oriented E-field, and therefore has a vertical polarization.
![]() FIGURE 3-1 Electromagnetic spectrum. (Shared by Philip Ronan under the Creative Commons Attribution-Share Alike 3.0 Unported license.) IR, infrared; UV, ultraviolet. |
As the source oscillates, new waves continue to form at the frequency (ν, Greek letter nu) of the oscillation. The resulting wavelength (λ, Greek letter lambda) of the EMR is then fixed by the velocity (c) and the frequency (ν):

Note that frequency (ν) and wavelength (λ) are inversely proportional, that is, the longer the wavelength, the lower the frequency, and vice versa. EMR waves differ in their wavelength (and their equivalent frequency). The EMR spectrum is a continuum that is arbitrarily broken up into ranges called bands (Figure 3.1).
Visible light makes up only a small part of the EM spectrum. The only difference between visible light and the other parts of the EM spectrum is that, as the term suggests, the former may be detected by the human eye. Observe how the visible spectrum is oriented to the rest of the EM spectrum. Blue light has a shorter wavelength and therefore a higher frequency than the other visible wavelengths such as green, yellow, orange, and red. It is also positioned directly next to ultraviolet (UV), whereas red is positioned next to infrared (IR). Therefore, the light wave’s wavelength (and frequency) and the particles’ energy making up the light wave are related.
LIGHT AS A PARTICLE
As stated earlier, light may be described either as EM waves or as the flow of particles. The particle in question is called the photon. The photon is a discrete packet of energy (or quantum) for all forms of EMR, including light. The photon is the force carrier for the EM force, which means that while the EMR wave moves the energy through space, the photon is responsible for the actual transfer of energy or force between particles (i.e., matter).
A photon’s energy (and therefore the light wave) is quantified by the equation E = m(hν), where h is the Planck’s constant, ν is the frequency, and m is an integer, 1, 2, 3 … . More commonly, the photon equation is displayed like this:
E = hν
This equation provides a relatively complete understanding of how the wave nature of light relates to light’s particle nature. As stated earlier, frequency and wavelength are inversely proportional, whereas energy is directly proportional to frequency. Therefore, when comparing blue light to red light, it may be immediately noticed that blue has a shorter wavelength than red and therefore a higher frequency. The higher frequency of blue light indicates that blue has higher energy. It is well known that UV is harmful to tissue due to its high frequency and high energy3; blue light falls on the spectrum adjacent to UV, and red is adjacent to IR.
INTERFERENCE
The oscillation of the E-field of a light wave can be described by a sine wave. As a sine wave, the light wave has amplitude, frequency, wavelength, and phase. The phase of any sine wave is measured in degrees or radians, representing the time or distance elapsed from the source of oscillation in one cycle. The phase that two or more waves have at a point of summation will determine how they interact. When two or more waves come together at a point in space, their energy fields’ summation is referred to as interference. Interference can occur when light waves from a single source (originally in phase) are divided and recombined after traveling through separate paths with different lengths. A phase difference (δ) can result between two waves of the same wavelength (λ) because of either a difference in time of generation (Δt) or a difference in path length (Δx) traveled by waves to reach that point or a combination of both.
When two waves meet at a point in space so that they are in phase (δ = 0 or multiples of 360), they are said to undergo complete constructive interference. When two waves undergo constructive interference, their amplitudes add together, thereby amplifying the wave. When two waves are 180° out of phase at a point in space (δ = 180 or multiples of 180), they undergo complete destructive interference. Waves that undergo destructive interference cancel out one another’s amplitudes. Interference that is neither wholly constructive nor wholly destructive is referred to as partial interference.
COHERENCE
When all of the waves in a beam of light have equal wavelength and frequency (i.e., they are the same color) and all have the same phase (crests coincide with crests and troughs with troughs, i.e., δ = 0), then the light is said to be coherent. Except for lasers, most light sources do not produce highly coherent light. Most light sources are incoherent because they emit polychromatic wavelengths, all of which have different frequencies.
ATOMS AND ENERGY LEVELS
EMR is emitted and absorbed by matter. Atoms are the building blocks of all molecules and are the smallest components of matter representing a chemical element such as hydrogen or helium. An atom is composed of a nucleus of protons (positive charge) and neutrons (neutral), whereas negatively charged electrons “orbit” around the nucleus in regions of space called orbitals. To appreciate the scale, if the nucleus of an atom were the size of a grain of dust on a pitcher’s mound, the orbitals making up the remainder of the atom would fill a professional baseball stadium.
There are certain fixed orbits in which electrons orbit an atom. Each of these orbits corresponds to a certain amount of total energy, and an electron can only change from one orbit to another by giving up or receiving an amount of energy, which is the difference in energy between the two orbits. It should be noted that electrons cannot fall anywhere in between orbitals. Thus, photons of a quantal amount of energy are emitted when electrons jump from a higher energy orbit (an outer orbit) to a lower energy orbit (an inner orbit). The amount of energy is ΔE = Eouter – Einner = hν (Figure 3.2). Orbitals can also have different shapes. More complex orbital shapes have a higher energy than those that are more simple in shape. These orbitals are also known as energy levels. An energy level is the energy necessary to move an electron from a lower to a higher orbital or to a different orbital shape.
Orbitals farther from the nucleus have a much higher energy level than orbitals closer to the nucleus. The highest energy electrons—the most unstable—are found in the outermost orbitals and are called valence electrons. Valence electrons are responsible for the chemical reactivity of an element and the electrical conductivity of an element.
Once an electron is excited, it cannot maintain the excitation for long. It returns to its previously occupied lower orbital by emitting energy in the form of EMR (emission). This energy is emitted as a photon, and this is responsible for the production of light.
ABSORPTION
For emission to occur, an atom’s electrons must first be elevated to a higher energy level through absorption (Figure 3.3). The only frequencies absorbed are those that have just the right amount of energy (E = hν) to elevate electrons to the particular energy levels available in the atoms. The frequencies a substance strongly absorbs are known as resonance frequencies. The type of absorption (and scatter) depends on whether the matter with which the light interacts is rarefied or dense.
Rarefied Gas Made Up of Atoms: Atomic Gas
In a rarefied gas made up of atoms, the resonant frequencies (absorption bands) are extremely narrow. For example, sodium vapor absorbs strongly (and thus scatters strongly) at 589 nm (yellow) but allows most other wavelengths to easily get through. The sodium atom dipoles resonate at ν = c/λ = (3 × 108 m/s)/589 nm = 5.09 × 1014 Hz and reemit EMR at the same frequency (and wavelength) but in all directions, a process called resonant scatter.
Rarefied Gas Made Up of Molecules
In a rarefied gas made up of molecules, the absorption bands are broader due to interactions between atoms held together in molecules. Scatter is again greatest for wavelengths near the resonant frequencies. For example, air molecules (N2 and O2) have a resonant frequency in the UV range. Therefore, most of the scattering of visible light in the atmosphere is for blue light, which is closest to UV, and as a result, the sky appears blue.
CONDENSED MATTER: SOLIDS, LIQUIDS, AND DENSE GAS
Opaque Substances
In condensed matter, the absorption bands run together due to the interactions between close neighboring atoms and molecules (particles). These particles are so close together that when one particle absorbs EMR, it transfers some of the energy to adjacent particles through frictional forces before reradiation can occur, and the energy is converted from EMR energy to the kinetic energy of particle motion (heat). Such matter is opaque to those absorbed wavelengths. The kinetic energy causes lower frequency (ν) oscillations of the particles, so the energy is reradiated as IR light, but the original wavelength is lost.
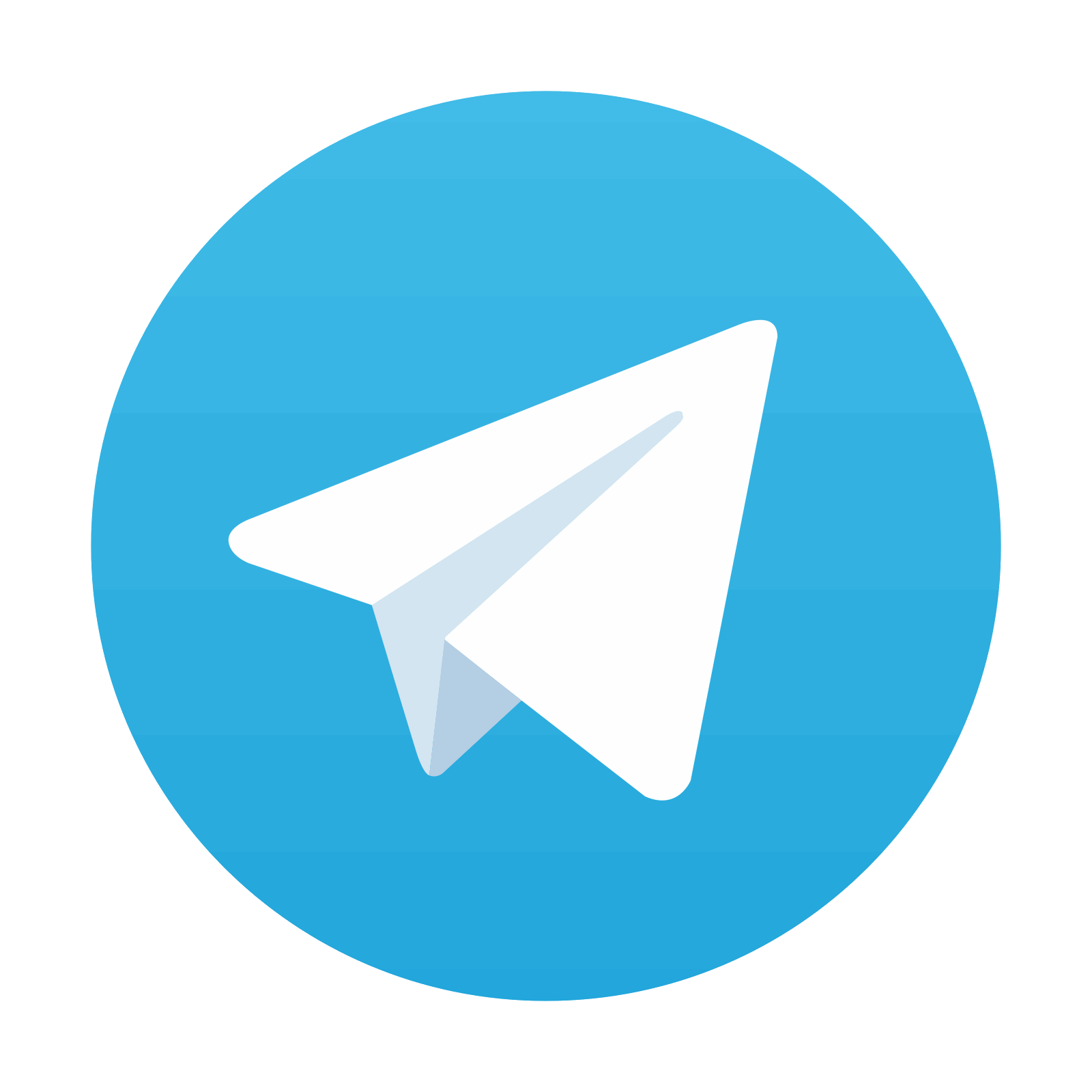
Stay updated, free articles. Join our Telegram channel

Full access? Get Clinical Tree
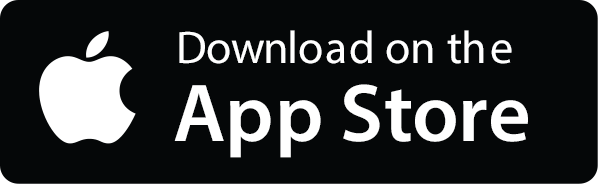
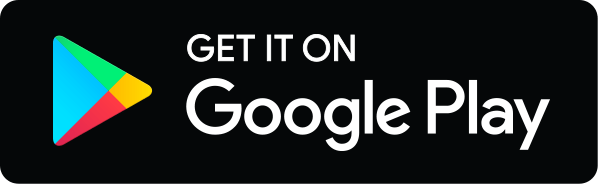