CLINICAL TESTS OF CENTRAL VISUAL FUNCTION
Visual acuity testing
Contrast sensitivity testing
Brightness sense testing
Photo-stress testing
Amsler grid testing
Color vision testing
Stereopsis testing
Other tests of central vision
VISUAL FIELD TESTING
The visual field: size and shape
Perimetry techniques
Interpreting perimetry results
PHYSIOLOGICAL AND ELECTROPHYSIOLOGICAL RESPONSES
Relative afferent pupillary defect
Electrophysiological tests
KEY POINTS
As discussed in Chapter 1, the clinical examination evaluates the structure (eg, eye examination, neuroimaging) and the function of the visual apparatus. The structural aspects of the examination are relatively objective, but as we shall see in this chapter, evaluating function introduces a significant subjective component.
In order to understand, treat, and follow disorders of the visual system, it is vital to measure how well a patient sees. Although seemingly simple, this task is far more difficult than one might think. Vision is a sensory experience, and sensory examinations are inherently difficult to perform and interpret. Most tests of visual function are subjective, since the examiner depends on the patient’s description of what he or she perceives in response to a test stimulus. For this reason, visual function testing suffers from the same shortcomings as all other sensory tests—testing is subjective and depends on the willingness and ability of the patient to respond. Important exceptions to this general rule include clinical tests that employ physiological responses (the relative afferent pupillary defect [RAPD] test and optokinetic nystagmus [OKN] test) and electrophysiological tests (full-field and multifocal electroretinogram [ERG] and visually evoked potential [VEP] tests). Functional neuroimaging, as discussed in Chapter 1, could also be theoretically included in this group of objective tests of visual function.
The subjective nature of visual function testing requires that clinical tests of visual function be interpreted by the examiner: The meaning of the test result depends on the limitations of the study, reliability of the patient’s input, and correlation with the objective aspects of the examination. The results of visual function testing can be confusing in patients who cannot or will not cooperate fully, or when the subjective and objective components of the examination do not fully agree, as with nonorganic visual loss (discussed in detail in Chapter 6).
The complexity of the visual experience also makes it difficult to objectively quantify. Vision cannot be fully characterized by a number such as “20/30,” as it encompasses an expansive panorama of colors, contrasts, shadows, and motion. Even the most sophisticated clinical tests provide only an estimate of a limited aspect of visual function, and the data may not correlate directly with a patient’s experience in the real world. To obtain a meaningful assessment of a patient’s visual function, a number of different tests may be required. Choosing the appropriate tests requires an accurate and directed history (as discussed in Chapter 1) and an understanding of the types (and limitations) of clinical tests of visual function.
The important features of clinical tests that measure central vision and the visual fields, as well as physiological and electrophysiological responses that can be used to assess visual function, are explored in this chapter. Emphasis is placed on the three tests that form the basis of all afferent vision assessments, often called the “vital signs of vision”: visual acuity, visual field, and relative afferent pupillary defect (RAPD) testing.
The visual axis of the eye is coincident with the foveloa. The foveola is the center of the fovea, a specialized area of the retina that corresponds to central vision. The unique architecture and neural circuitry of the fovea permit high resolution and sensitivity, which are vital for important visual tasks such as reading and recognizing faces. Patients are generally symptomatic from even the most minor disturbances in their central vision.
Visual acuity tests are composed of lines of characters that become progressively smaller, presented as a printed wall chart or near card, or by a projector system or computer. The most common format, the Snellen visual acuity test, uses uppercase letters as the test characters (optotypes) (Figure 2–1A). Other optotypes are available that do not depend on alphabet recognition, such as the line pictures that are used to test young children, or Landolt rings (shaped like the letter “c”) and “tumbling Es,” in which patients are asked to simply identify the orientation of the character (Figure 2–2). Unlike the Snellen visual acuity chart, LogMAR acuity tests present the same number of optotypes for each acuity line. This uniformity is necessary for statistical modeling in research studies (Figure 2–3).
Figure 2–2.
Other common optotypes used in testing visual acuity.
A variety of optotypes are available to test young children or illiterate adults. The subject may simply name the object or point to a matching picture on a control card that has large images of the symbols: Allen figures (1), Lea symbols (2), HOTV optotypes (3).
Other optotypes require the subject to say or point to show the orientation of the optotype, or to orient a symbol card he or she is holding to match the symbol on the chart: Landolt rings (4), “tumbling Es” (5).

Figure 2–3.
LogMAR visual acuity tests.
Snellen acuity testing has a number of shortcomings, the most obvious being that the various lines do not have the same number of test letters, ranging from one 20/400 letter to eight 20/20 letters. This causes nonuniformity of perception (crowding phenomenon) and makes statistical comparisons of changes in acuity cumbersome. LogMAR charts, such as the ETDRS (Early Treatment Diabetic Retinopathy Study) acuity chart pictured, have the same number of optotypes on each line, forming an inverted pyramid. The size of the optotype changes linearly, so visual acuities can be reported as the log of the angle of discrimination (a statistically friendly number). LogMAR acuity charts are used primarily in clinical research studies. This visual acuity chart uses Sloan letters, a letter optotype that is analogous to the Landolt C rings.

In almost every case, the physician is primarily interested in the patient’s best corrected visual acuity, so the test is performed with the patient wearing his or her glasses.
Visual acuity testing is typically performed at 20 feet. At this distance, accommodation is relaxed and unlikely to interfere with measuring the patient’s true distance acuity (or determining their refraction). However, near cards are far more practical for the bedside examination (Figure 2–4), as long as the examiner allows for presbyopia (typically in patients older than 45 years) by having them wear their bifocals or reading glasses.
Figure 2–4.
Using a near card.
There are a variety of near cards (with a selection of optotypes) available for testing visual acuity at the bedside. Important considerations include (1) patients need to wear their best correction for reading (bifocals or reading glasses); (2) testing distance should be uniform, typically 14 inches; (3) lighting should be adequate; (4) the medical record should reflect use of the near card and glasses worn.

With Snellen acuity testing, the smallest line in which the patient can identify correctly more than one-half of the characters is designated as the Snellen visual acuity. A “+” modifier shows that a few characters on the next smallest line were also correctly named; a “−” modifier identifies the number of characters missed on the designated acuity line (eg, 20/30 − 2, 20/60 + 3) (Figure 2–5).
Figure 2–5.
Notation of visual acuity.
“VA” is the standard notation for visual acuity (1). The visual acuity of the right eye is written on top (2) (often designated OD or RE), and the left eye on the bottom (OS or LE). A “+” or “−” is added to the Snellen acuity (3) to note that the subject read a few letters on the following line (+), or to show the number of letters that could not be read on the designated acuity line (-). A “d” (or no designation) means that the standard 20-foot test distance was observed (4). An “n” shows that a near card (with Snellen-equivalent notation) was used to test the visual acuity (such as at the bedside). Patients who wear glasses should wear them when visual acuity is being tested (designated as “cc” for “with correction”) (5). If no glasses are worn, the designation “sc” is used.

The Snellen acuity notation is expressed as a fraction, with the numerator identifying the standard testing distance, typically 20 feet. Characters on the 20/20 line subtend an angular size of 5 minutes of an arc. To recognize a 20/20 character, the subject must be able to resolve components of the letter that measure 1 minute of arc (Figure 2–1B). The denominator in the Snellen notation designates the relative size of the test character. The 20/200 E is 10 times larger than the 20/20 letters on the chart. The denominator can also be thought of as the distance at which letters on a given line would subtend 5 minutes of arc. For example, a 20/100 letter at 100 feet has the same angular size as a 20/20 letter at 20 feet: Both measure 5 minutes of an arc. A practical way of understanding Snellen notation is as follows: A patient with 20/100 visual acuity (at 20 feet) can only distinguish what a normal-sighted person could still read from 100 feet.
The standard metric testing distance for Snellen visual acuity is 6 meters. Thus, the metric equivalent of 20/20 acuity is 6/6. Conversion between acuities obtained in feet or meters can be performed by treating the notation as a simple fraction: 20/40 = 6/12, 20/200 = 6/60.
Some patients may have such poor vision that they cannot see even the largest characters on the acuity chart at the standard testing distance. For these patients, the testing distance can be shortened, or methods other than Snellen acuity can be used to define the visual acuity (Box 2–1).
BOX 2–1. MEASURING AND RECORDING POOR VISUAL ACUITY
Shortening the test distance. Patients who cannot see even the largest Snellen letter can be tested by shortening the testing distance, either by bringing the patient closer to the chart or moving the acuity chart closer to the patient. At 10 feet, the test letters are twice the angular size than at 20 feet. As the numerator denotes the testing distance, a patient who could only see the 20/200 letters at 5 feet would be designated as 5/200. Because the Snellen notation can be treated as a simple fraction, this visual acuity would be equivalent to 20/800.
Tumbling E card. Rather than moving the chart or the patient, a 20/200 “tumbling E” card allows the examiner to test at any distance. The examiner can hold the card as close as required to the patient and vary its orientation. For example, if the patient can consistently state (or point at) which direction the 20/200 E is pointing at 5 feet, the visual acuity would be recorded as 5/200.
Counting fingers (CF). An alternative for patients whose visual acuity is “off the chart” is to record the distance at which the patient can accurately count the examiner’s fingers, for example, CF 5′, CF 2′. The size and spacing of an examiner’s outstretched fingers is roughly the same size as the 20/200 E. Thus, CF 5′ can be thought of as being (approximately) equivalent to 5/200.
Tests for very poor vision. For patients who cannot see well enough to count fingers, the ability to see hand movement (HM) is recorded. Patients with severe visual loss may have only light perception (LP), which can be further subdivided as “LP with projection,” “LP without projection,” or no light perception (NLP).
Visual acuity testing is performed one eye at a time. Care must be taken to fully occlude the other eye. Patients may inadvertently peek around handheld occluders with their better eye. This situation typically occurs when spectacles prevent close apposition of the occluder to the eye, or when patients insist on moving their head around to see better. In an effort to please, children frequently peek with their better eye. The patient’s (or examiner’s) hand may be used to cover an eye. The palm of the hand, not the fingers, should be used to insure occlusion. In patients wearing glasses, a folded tissue can be slipped behind the lens and positioned for reliable occlusion if a handheld occluder is not available or sufficient.
The measured visual acuity is severely affected by refractive errors. Thus, the visual acuity test is not a good indicator of the health of the visual pathways unless all refractive errors are accounted for. Although “pinhole acuity” can give a rough idea of true visual acuity, whenever possible, a full refraction should be obtained to determine the best corrected visual acuity on every patient with a complaint of visual loss (Box 2–2).
BOX 2–2. PINHOLE ACUITY
We appropriately emphasize the necessity of determining the patient’s true best-corrected visual acuity. Decreased visual acuity that can be corrected with the phoropter suggests the need for eyeglasses, rather than an extensive evaluation. Conversely, vision that cannot be fully corrected with lenses suggests pathology. Pinhole acuity provides a reasonable substitute when a phoropter and refraction are not available, as may be the case in a neurology clinic. The pinhole is an optical trick that essentially serves as a universal lens, much like a pinhole camera. Visual acuity measured with a pinhole is a reasonable approximation of visual acuity at distance when a simple refractive error is the only problem. Since the pinhole eliminates optical aberrations from corneal and lenticular astigmatism, it can provide better acuity than glasses in a condition with irregular astigmatism such as keratoconus. Patients with monocular diplopia from optical media (eg, cataract, ocular surface disease) will note that their diplopia disappears with a pinhole.
Patients should have their visual acuity tested one eye at a time (first without the pinhole) in their distance glasses, if they have them. If the visual acuity is not good, then test with the pinhole (have patients keep their glasses on if they have them). It sometimes takes patients a few seconds to find a hole to look through. Significant improvement in the acuity means that the subnormal visual acuity is likely a refractive (glasses) issue, rather than pathological. However, be aware that some disorders can cause refractive changes secondarily, such as a myopic shift with cataract, or a hyperopic shift with papilledema or orbital mass.
Much can be learned by simply listening to patients as they attempt to read a visual acuity chart. Patients with homonymous hemianopic visual field defects may consistently fail to see the first or last letters of a line. Patients with marked constriction of the visual field, such as with retinitis pigmentosa, might have great difficulty even locating the eye chart in front of them, but may then read the smallest lines once they are “lined up.”
The widespread use of Snellen visual acuity testing allows a common basis to compare visual acuities obtained almost anywhere. Unfortunately, the comparisons will be rough estimates at best, because there are so many uncon-trollable variables inherent in this test method: different instruments for presenting the chart, variations in illumination of the chart, inconsistent light-adaptation of the patient, and varying examiner techniques.
Visual experiences in the real world often consist of vague and subtle contrast differences, not at all like the high-contrast visual acuity letters tested in the physician’s office (Figure 2–6). Contrast sensitivity testing requires the subject to try to discern the presence (or orientation) of “fuzzy bars.” The test is composed of alternating bright and dark lines, where luminance varies sinusoidally (Figure 2–7A, B). By varying the spacing of the bars (spatial frequency) and amplitude of the sinusoidal wave (contrast), the data collected determine a contrast sensitivity curve (Figure 2–7C), rather than a single value like the Snellen visual acuity. As with visual acuity testing, a number of modalities exist for presenting contrast stimuli including wall charts, projector systems, near cards, and computer-generated tests. The Pelli-Robson Contrast Sensitivity Chart is a popular variation that combines classic optotypes with decreasing contrast (Figure 2–8).
Figure 2–7.
Contrast sensitivity testing.
(A) This version of a contrast sensitivity test (CSV-1000 VectorVision, Inc., Dayton, OH) uses an illuminated distance chart. This format is a “forced-choice” test, because the patient is asked to identify which of two circles contains the contrast sensitivity pattern. The gratings are in four groups of increasing spatial frequency from top to bottom. Within each group, the contrast level decreases from left to right. (B) The gratings have a sinusoidal luminance, with the spatial frequency corresponding to the cycle of the sine wave, and contrast corresponding to the amplitude. The red curve in this diagram has the highest contrast. The dashed blue curve is lower in contrast, but both have the same spatial frequency and average luminance. (C) The results of the test are plotted, with spatial frequency on the horizontal axis and contrast on the vertical axis. Normal age-matched values are provided as shaded areas. This subject has an abnormal “notch” at an intermediate frequency, resulting from a previous episode of optic neuritis.

In some cases, patients with visual complaints have relatively normal visual acuities, but abnormal contrast sensitivity curves (Box 2–3). For example, patients with multiple sclerosis may do relatively well at the high and low frequency ends of the spectrum, but may be missing a “notch” in the mid-range. Chronic papilledema and glaucoma may affect the low-frequency end of the curve, leaving the high spatial frequencies relatively unscathed. Cataract and refractive errors affect the high-frequency end far more severely than the rest of the curve.
BOX 2–3. PARALLEL RETINOGENICULATE PATHWAYS
There are many types of ganglion cells identified in the retina, each type coding for different kinds of information, and some projecting to destinations other than the lateral geniculate nucleus (LGN). Mammalian visual systems demonstrate (at least) two distinct projections of axons from the retina ganglion cells to the LGN, with distinct projections from the LGN to the visual cortex. These parallel pathways are most evident anatomically in the LGN, where axons from specific groups of ganglion cells synapse with specific populations of neurons in the LGN (see Figure 5–13). These anatomically distinct LGN neurons are thought to subserve different components of visual perception and define distinct “channels” of visual information processing: Small parvocellular neurons (P cells) transmit color and fine discrimination information; larger magnocellular neurons (M cells) transmit information about motion, stereopsis, and low spatial frequency contrast sensitivity. The extent to which some disorders of the visual system preferentially affect one or the other of these parallel systems can be of diagnostic help. Most physicians are more familiar with tests that predominantly evaluate the parvocellular system than with the more specialized clinical tests that examine the magnocellular system. Other than contrast sensitivity testing, M-system tests are not routinely used by most physicians, but are of value in evaluating neuro-ophthalmic disorders.
P-system tests
Visual acuity testing
Color discrimination
Contrast sensitivity testing (high spatial frequencies)
M-system tests
Motion discrimination tests
Critical flicker fusion testing
Tests of stereopsis
Contrast sensitivity testing (low spatial frequencies)
Like most tests of central vision, the contrast sensitivity test is rarely diagnostic by itself; rather, it offers additional information regarding the nature of visual loss. Measuring contrast sensitivity is particularly useful as part of a battery of optic nerve function tests when clinical decisions rest on subtle progression of disease (eg, optic nerve sheath meningioma, compressive orbital Graves disease, or chronic papilledema).
Patients with optic nerve disorders may describe their vision as dim, rather than blurry. For example, a patient who has recovered from optic neuritis may note a dramatic difference in brightness between the normal and affected eyes, even when the visual acuity and visual field in each eye are relatively normal. Such patients may volunteer that the light of the slitlamp or indirect ophthalmoscope is more tolerable in their affected eye.
The simplest brightness test consists of shining a bright light in each eye in turn and asking the patient to comment on the perceived brightness. Many physicians have the patient subjectively grade the brightness in the affected eye, comparing it to the normal eye at 100%. The brightness difference between the two eyes may also be measured by using neutral density filters of varying density over the normal eye, in an attempt to subjectively match the two eyes. Brightness sense testing is generally useful only in patients with monocular disorders, because a normal eye is needed for comparison.
Not uncommonly, it may be difficult to determine whether central visual loss is due to pathology in the retina (macula) or the optic nerve. In the diseased macula, photoreceptors take longer to recover from a very bright light (photo-stress) than in the healthy retina. Photo-stress recovery time is not altered by optic nerve disease. The photo-stress test is performed by first identifying the smallest line on the Snellen acuity chart that the patient can read under normal testing conditions with each eye. Each eye in turn is then exposed to a bright light (such as the indirect ophthalmoscope beam) for 10 seconds to bleach the macula. The time it takes for the patient to recover the ability to read the designated Snellen acuity line is measured for each eye. Prolonged photo-stress recovery times suggest macular rather than optic nerve disease. Similar to brightness sense testing, photo-stress testing is mainly beneficial in patients with monocular complaints (who have a normal eye for comparison).
The Amsler grid is a square containing small grid lines with a central target (Figure 2–9). This test is most useful in identifying the metamorphopsia that frequently accompanies macular disease, both as a diagnostic tool and for patient self-monitoring at home. Patients with maculopathies may report that the lines are bent, twisted, or misshapen.
Figure 2–9.
The Amsler grid test.
(A) The Amsler grid is held at reading distance (14 inches or 40 cm) and one eye is tested at a time, with best near correction (glasses used for reading). The patient is instructed to look directly at the central dot and is asked, “Can you see all four corners of the grid? Are any of the lines blurry, wavy, distorted, bent, gray, or missing?” (B) Most Amsler grid scoring sheets are printed as a tablet, so the patient (with the help of the examiner) can designate abnormal portions of the grid by drawing or writing comments directly on the grid, which can then be entered into the patient’s chart (or scanned into electronic medical records). (C) This Amsler grid is from a patient with metamorphopsia due to an epiretinal membrane. This is the same patient depicted in Figure 6–7.

The Amsler grid is also a useful test of the central visual field. When held at 33 cm, the grid covers approximately a 10° radius of the visual field from fixation (each box is ∼1°). Central visual field defects may make it difficult for patients to see the central dot, or they may note that some of the squares or lines are missing or are lighter than others.
Amsler grids are available in many forms: printed pads, wallet cards, online versions (recommended for the computer “home page” of patients with macular degeneration), and as smartphone apps. Variations include white lines with a black background or as a graded decrease of the contrast of the grid lines.
Color vision abnormalities may be congenital or acquired. Congenital color defects are usually caused by chromosomal abnormalities in coding for one of the three cone pigments. Defects are named by designating a prefix to describe which pigment is defective: protan = red, deuteron = green, tritan = blue. A suffix denotes whether there is complete absence (-opia), or merely a deficiency (-anomaly). For example, a complete lack of red pigment cones would be protanopia, whereas a deficiency of green cones would be deuteranomaly.
The presence of all three cone pigments in normal concentration is required to distinguish the entire spectrum of the color wheel. Most congenital color deficiencies are the result of chromosomal abnormalities coding for the red and green pigments on the X chromosome, and are thus manifest more commonly in men (8% of men and boys in the general population). Congenital deficiencies tend to cause “pure” red/green defects with predictable color-matching deficits.
Acquired color vision deficits may be caused by optic nerve or retinal disease (and rarely cortical disease). Optic nerve disorders reportedly tend to affect red/green color discrimination, whereas macular disorders create more blue/yellow confusion (Kollner rule). However, unlike congenital color defects, acquired disorders tend to affect the cone pathways indiscriminately. Acquired color defects are therefore more widespread in their effects on the spectrum of the color wheel, and the Kollner rule is only a rough guideline at best.
Most clinical color vision tests are specifically designed to detect and classify congenital defects, but are helpful in acquired disorders as well.
The most exhaustive (and exhausting for the patient) clinical test of color vision is the Farns-worth-Munsell 100 hue test (FM-100). This is a just-noticeable-difference task, which means that even normal subjects are expected to find the test difficult and to make errors. The test involves placing 85 small tiles with subtle differences in color in rank order along the color spectrum (Figure 2–10). The FM-100 is only occasionally used in the clinical setting because it is so time-consuming and tedious. The Farnsworth D-15 is a color-ranking test similar to the FM-100, but is much shorter (only 15 tiles) and can give information similar to that of the FM-100 (Figure 2–11).
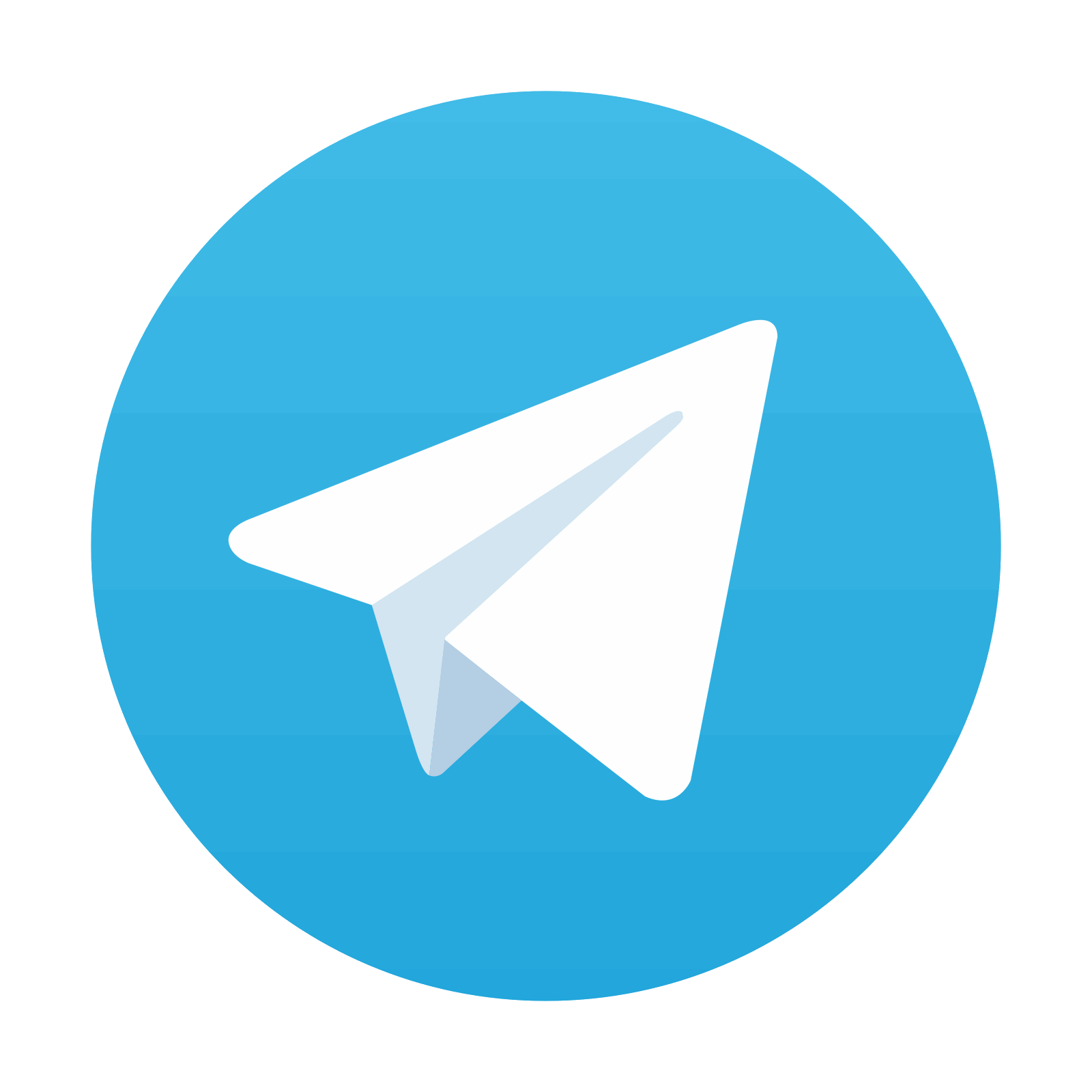
Stay updated, free articles. Join our Telegram channel

Full access? Get Clinical Tree
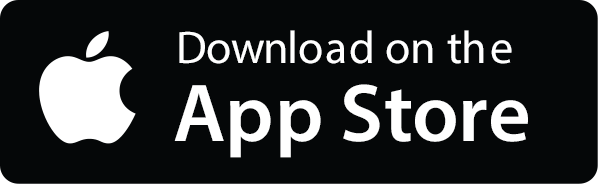
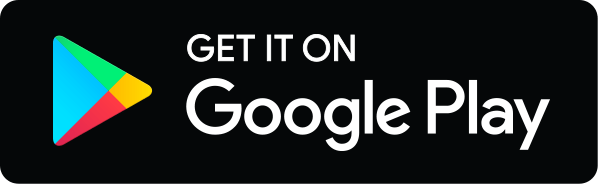