13 Taste and Smell in the Elderly
Introduction
It is well established that taste and smell function decline with age in a large proportion of the population.1,2 Such decrements are not without consequence. For example, in a study of 750 consecutive patients presenting to our Smell and Taste Center with complaints of chemosensory disturbances, 68% experienced decreased quality of life, 46% reported changes in appetite or body weight, and 56% noted adverse influences on daily living or psychological well-being.3 In another study of over 400 patients, many of whom were elderly, at least one hazardous event, such as failure to detect fire or leaking natural gas, was reported by 45.2% of those with anosmia, 34.1% of those with severe hyposmia, 32.8% of those with moderate hyposmia, 24.2% of those with mild hyposmia, and 19% of those with normal olfactory function.4
This chapter provides an overview of the functional and pathophysiological changes in the senses of taste and smell that are associated with aging. The goal is to provide the clinician with a basic understanding of these changes and information of value in treating and counseling older patients who present with aberrations of these senses. Although smell dysfunction can be an early sign of several age-related diseases, most notably Alzheimer disease and sporadic Parkinson disease, the reader is referred elsewhere for more detailed reviews on these topics.5,6
Basic Anatomy of the Taste and Smell Systems
The Oral Taste System
Chemicals that enter the oral cavity are sensed by taste receptors within goblet-shaped taste buds consisting of receptor, supporting, and basal cells (Fig. 13.1). Such buds, which number around 8,000 in humans, are embedded within the fungiform, foliate, and circumvallate papillae of the tongue (Fig. 13.2), as well as within the mucosa of the soft palate, uvula, epiglottis, rostral esophagus, and laryngeal surface of the epiglottis and spread caudally along the aryepiglottic folds, reaching peak density at the laryngeal side of the arytenoid tubercle. Some taste sensations, such as sweet, bitter, and savory (umami), are dependent upon G-protein-coupled receptor proteins located on receptor cell microvilli within the buds. Interestingly, such proteins are rather ubiquitous throughout the body, being found within the linings of the larynx, epiglottis, stomach, pancreas, and colon. In the gut, they influence chemical absorption, digestion, insulin release, and the metabolism of swallowed foods and beverages.7
Fig. 13.1 Idealized drawing of longitudinal section of a mammalian taste bud. Cells of types I, II, and III are elongated. These cells have different types of microvilli within the taste pit and may reach the taste pore. Type IV are basal cells and type V are marginal cells. Classically defined synapses occur only between type III cells and nerve fibers. Many of the connecting taste nerves have myelin sheaths. (Reprinted with permission from Witt M, Reutter K, Miller IJ, Jr. Morphology of the peripheral taste system. In: Doty RL, ed. Handbook of Olfaction and Gustation. New York, NY: Marcel Dekker; 2003:651–677.)
Fig. 13.2 Schematic representation of the tongue demonstrating the relative distribution of the four main classes of lingual papillae, three of which have taste buds (indentations on insets represent taste buds). Taste buds are particularly plentiful on the sides of the foliate and circumvallate papillae. Ebner glands secrete materials into the valleys between the latter papillae. The fungiform papillae can vary considerably in size and are more dense on the anterior lateral regions of the tongue.
Several mechanisms are involved in taste transduction, depending on the tastant and its associated qualitative sensations.8,9 The taste receptors involved in sweet, bitter, and savory sensations fall into distinct G-protein receptor gene families. The gene family associated with sweet and savory sensations comprises three receptor genes (TAS1R–TAS1R3), whereas the bitter receptor gene family comprises over 60 receptor genes (TAS2R1–TAS2R60), although some are not found in humans or are pseudogenes. In contrast, salty and sour sensations are not mediated by G-protein-related receptors. Salty taste, such as that produced by sodium chloride, requires dissociation of the salt into Na+ and chloride (Cl−) ions. The diffusion of the Na+ ions through specialized membrane pores on the receptor cells induces cell depolarization and transmitter release. Sour taste, such as that induced by hydrochloric acid, is similarly produced via protons (H+).
The neural projections of the peripheral human taste system are shown in Fig. 13.3. The taste buds on the anterior tongue are innervated by the chorda tympani branch of cranial nerve (CN) VII, which merges with fibers of the lingual nerve. The taste buds on the palate are also innervated by CN VII with connections through the geniculate ganglion. The taste buds on the foliate and circumvallate papillae receive their innervation from the glossopharyngeal nerve (CN IX), whereas those on the larynx and esophagus are innervated by the vagus nerve (CN X). In all cases, the afferents from the taste buds enter the brainstem and synapse within the nucleus tractus solitarius (NTS), a structure that extends from the rostrolateral medulla caudally along the ventral border of the vestibular nuclei. The fibers synapse in an orderly rostral to caudal fashion, those from CN VII being rostral to those from CN IX, which in turn are rostral to those from CN X.
Fig. 13.3 Course of peripheral taste nerves. FN, facial nerve; Ggl, geniculate ganglion; Ndgl, nodosal ganglion; Pgl, petrosal ganglion; SGP, superficial greater petrosal nerve; tm, tympanic membrane; Slgl, semilunar ganglion; SLN, superior laryngeal nerve; GLN, glossopharyngeal nerve; CT, chorda tympani. (Reprinted with permission from Welge-Luessen A. Management of Smell and Taste Disorders. New York, NY: Thieme; 2013.)
The second-order neurons within the NTS ascend ipsilaterally to the parvicellular division of the ventroposteromedial nucleus of the thalamus via the central tegmental tract.10 From this nucleus, projections are made to the primary taste cortex, a set of structures located at the junction of the anterior insula and the inner operculum. Further projections are then made to other structures, most notably the orbitofrontal cortex, in which interactions occur with neurons from the visual, somatosensory, and olfactory systems.
The Olfactory System
Unlike the taste system, the primary stimuli of this system are vapors. Odorants enter the nose by diffusion or inhalation and dissolve in the mucus that overlies the olfactory neuroepithelium. This pseudostratified columnar epithelium lines the cribriform plate, sectors of the superior septum, and both the superior and middle turbinates. Like the receptors involved in sweet, bitter, and savory taste sensations, the olfactory receptors are G-protein-coupled receptors. They are embedded in the ciliary membranes of 6 to 10 million bipolar receptor cells located in the neuroepithelium (Fig. 13.4).11 Nearly 400 different receptor proteins are expressed in humans. Interestingly, each receptor cell expresses only one type of receptor protein. Unlike the mucus within the nasal epithelium, that of the olfactory region comes from specialized Bowman glands. These secretions are replete with numerous types of enzymes that degrade xenobiotics and odorants.12 Other cell types found within this specialized epithelium are (1) supporting cells, which, among other things, insulate the olfactory receptor cells from one another; (2) microvillar cells, which likely secrete nitric oxide and play an antibacterial role; and (3) basal stem cells. The latter cells differentiate into the other cell types, replacing them when they sustain damage or death from xenobiotics, aging, or other processes.13 Unfortunately, receptor cell restoration following damage is often incomplete or nonexistent.
The axons of the olfactory receptor cells coalesce within the lamina propria into multiple bundles, termed the olfactory fila. Each filum contains fascicles ensheathed by glial cells with astrocyte- and Schwann cell mesaxon-like properties.11 The receptor cell axons course within the fila through the foramina of the cribriform plate to synapse within the olfactory bulb, an outgrowth of the forebrain made up of neurons, nerve fibers, microglia, astrocytes, and blood vessels (Fig. 13.5). Considerable neural processing occurs within this structure. The axons of the receptor cells enter into sphere-like structures, termed glomeruli, where they synapse with dendrites of the major projection neurons of the bulb, the mitral and tufted cells. The axons of the receptor cells secrete the excitatory amino acid transmitter glutamate, which activates both N-methyl-D-aspar-tate (NMDA) and non-NMDA receptors on the dendrites of the mitral, tufted, and other second-order neurons.14 Some cells intrinsic to the olfactory bulb, termed periglomerular cells, influence neural activity among glomeruli and are largely dopaminergic. These cells serve to modify the incoming signals and, when excessive stimulation occurs, suppress olfactory nerve and mitral/tufted cell activity, in effect decreasing the volume of olfactory bulb output.15 Cells expressing gamma-aminobutyric acid (GABA), located in the core of the bulb, are termed granule cells and extend processes into more peripheral bulbar layers and modulate olfactory bulb activity, in some cases as a function of bodily needs such as hunger or arousal.16 Interestingly, these cells, as well as several cells near the glomeruli, termed periglomerular cells, can be repopulated by neuroblasts that migrate from the subventricular zone of the brain through the anterior rostral migratory stream.17 There is some controversy as to the degree to which this happens in humans.18
Fig. 13.4 (a) Cross-section of the human olfactory epithelium. Four main types of cells can be discerned: bipolar receptor cells (arrows point to largely denuded cilia at dendritic knobs); c, cell body; m, microvillar cell; s, sustentacular cells; b, basal cells. bg, Bowman gland; lp, lamina propria; n, collection of axons within an ensheathing cell; d, degenerating cell; bs, basal cell undergoing mitosis. (Photo courtesy of Dr. David Moran, Longmont, Colorado.) (b) A transition zone between the human olfactory epithelium (bottom) and the respiratory epithelium (top). Arrows signify two examples of olfactory receptor cell dendrites with cilia that have been cut off. Bar = 5 mm. (Used with permission from Menco BPM, Morrison EE. Morphology of the mammalian olfactory epithelium: form, fine structure, function, and pathology. In: Doty RL, ed. Handbook of Olfaction and Gustation. New York, NY: Marcel Dekker; 2003:17–49.)
Fig. 13.5 Schematic organization of the olfactory bulb. Arabic numbers indicate the cortical structure: 1, nerve fiber layer; 2, glomerular layer; 3, external plexiform layer; 4, mitral/tufted cell layer; 5, internal plexiform layer; 6, granule cell layer. Glomerular coding: Axons of receptor cell expressing the same olfactory receptor project to only a few analogous glomeruli (e.g., “blue” olfactory receptor neurons converge with “blue” glomeruli). Efferent (bulbofugal, blue) fibers project from mitral/tufted cells to secondary olfactory structures; afferent (centrofugal, gray) fibers project either from contralateral mitral cells or ipsilateral central nuclei and synapse to glomerular or granule cells, respectively. (Reprinted with permission from Welge-Luessen A. Management of Smell and Taste Disorders. New York, NY: Thieme; 2013.)
The mitral and tufted cell axons exit the bulb via the olfactory tract to synapse in the ipsilateral temporal lobe. Major projections include those to the anterior olfactory nucleus (AON), the piriform cortex, the periamygdaloid cortex, and the rostral entorhinal cortex (Fig. 13.6). Some tertiary connections to the opposite hemisphere occur via the AON and anterior commissure.
Age-Related Changes in Taste and Smell Perception
Most patients who complain of taste loss have, in fact, altered olfactory function that markedly alters flavors. This is because flavor sensations such as chocolate, mint, pizza, apple, strawberry, and so on are dependent on stimulation of the olfactory receptors via the rear of the nasopharynx (so-called retronasal route). Blocking the nose while eating, for example, greatly decreases or eliminates flavor sensation because active movement of air into the nasopharynx from the oral cavity is significantly impeded.
This section of the chapter specifically addresses age-related deficits observed in taste and smell perception, physiological processes that contribute to such deficits, and medications associated with changes in chemosensation in the elderly.
Fig. 13.6 Simplified schematic drawing of the essential bulbofugal olfactory pathways, deriving from mitral cells of the olfactory bulb. The lateral olfactory tract carries direct connections to secondary olfactory structures (“olfactory cortex”), before tertiary olfactory structures are reached. Contralateral projections and other afferents to the olfactory bulb are not indicated. (Reprinted with permission from Welge-Luessen A. Management of Smell and Taste Disorders. New York, NY: Thieme; 2013.)
The Oral Taste System
Age-related decrements in the ability to detect low concentrations of tastants, as measured by taste thresholds, are well documented for both electrical and chemical stimuli. For example, a recent meta-analysis identified 69 studies that have reported age-related declines in taste function, with most reflecting elevated olfactory thresholds.19 Threshold deficits have been found for all of the major taste qualities and include caffeine, citric acid, hydrochloric acid, magnesium sulfate, phenyl thiocarbamide (PTC), propylthiouracil (PROP), quinine, sodium chloride, sucrose, tartaric acid, and a large number of amino acids.20–34 However, the magnitude of the effects varies from stimulus to stimulus, and, in general, the effects of age seem to be greater for bitter tastants and the least for sweet tastants.35–37 Few studies have explored when the age-related progression in dysfunction occurs. An exception is a recent study that obtained electrogustometric thresholds from 461 subjects ranging in age from 15 to 94 years.22 These investigators concluded that taste loss typically began after the age of 60 years, although in some tongue regions the deficit appeared a decade later.
It should be noted that the detection of age-related deficits depends not only on the oral regions that are assessed but also on the type of testing that is performed. In general, deficits are more marked when testing involves small regions rather than large regions of the tongue. Whole mouth swishing of tastants stimulates multiple nerves and is less sensitive to subtle deficits. An example of the age-related decline in sensitivity of smell regions of the tongue to NaCl is shown in Fig. 13.7.32
Suprathreshold perception of tastants is also altered by age. Thus, although young people experience a significant increase in the intensity of a taste stimulus as its concentration is increased, some older persons experience a more blunted perception with the increased intensity. In one study, the slope relating intensity magnitude estimates to tastant concentrations was decreased in older adults relative to younger adults by an average factor of 1.76 for bitter-tasting compounds and 2.06 for sweet-tasting compounds.38
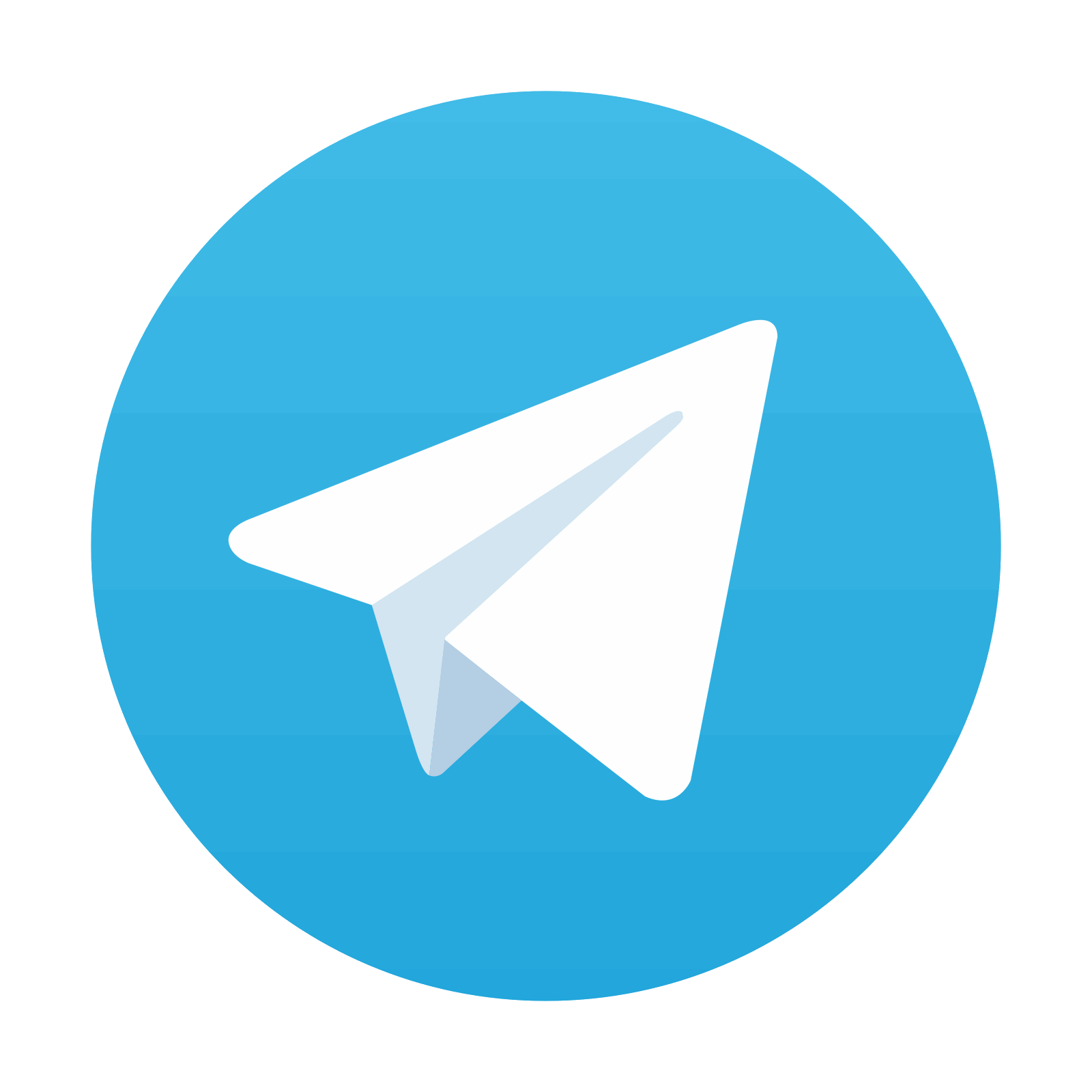
Stay updated, free articles. Join our Telegram channel

Full access? Get Clinical Tree
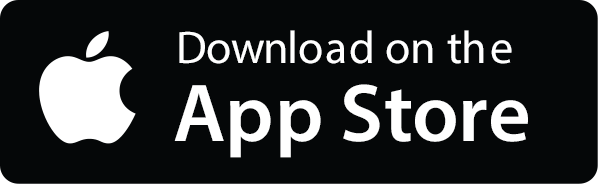
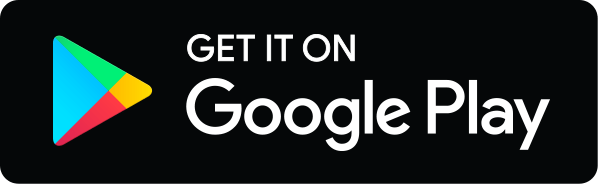