Some of the most enjoyable activities of daily living involve eating and drinking. These include meals (where eating and drinking are the purpose of the activity), special events such as receptions (where eating and drinking enhance the celebration), and relaxation activities such as going to the movies (where eating popcorn and drinking soda are an integral part of the experience for some people). Figure 16–1 is a cartoon that depicts the anticipation of a good meal and the social context in which it is enjoyed.
The ease of eating and drinking is deceptive. These are complicated activities that require intricately coordinated actions of the lips, mandible, tongue, velum, pharynx, larynx, esophagus, and other structures. Because eating and drinking engage many of the same structures and much of the same airway as those used for speaking and breathing, it is not uncommon for there to be competition between these activities or for tradeoffs to occur when trying to do them simultaneously. For example, chewing must stop to be able to speak clearly and breathing must stop to be able to swallow safely.
The entire act of placing liquid or solid substance in the oral cavity, moving it backward to the pharynx, propelling it into the esophagus, and allowing it to make its way to the stomach is called deglutition. Although the word swallowing is sometimes used as a synonym for deglutition, swallowing actually includes only certain phases of deglutition. Nevertheless, to simplify the explanations that follow, the term swallowing is used in place of deglutition and is meant to include all phases of deglutition.
Figure 16–1. Cartoon depicting the anticipation of a good meal and the social context in which it is enjoyed.
Figure 16–2 shows the structures that participate in swallowing. These structures extend from the lips to the stomach. Most of these same structures also participate in speech production; notable exceptions are the esophagus and stomach.
Breathing, Laryngeal, Velopharyngeal-Nasal, and Pharyngeal-Oral Structures
Structures within the breathing, laryngeal, velopharyngeal-nasal, and pharyngeal-oral subsystems participate in swallowing. These include the chest wall, vocal folds, ventricular folds, epiglottis, pharynx (laryngopharynx, oropharynx, nasopharynx), velum, tongue, mandible, and lips. Their anatomy is described in Chapters 2, 3, 4, and 5, and their functions during swallowing are described below. The anatomy of the esophagus and stomach is not covered in the other chapters and warrants attention here.
Figure 16–2. Structures of the swallowing apparatus. These include structures that participate in speech production (see Chapters 2, 3, 4, and 5 for detailed descriptions), as well as structures that do not (esophagus, stomach, and intestines and their associated sphincters).
The esophagus is a flexible tube, about 20 to 25 cm long in adults, which extends from the lower part of the pharynx to the stomach. The esophagus begins below the base of the larynx and runs behind the trachea, pulmonary apparatus, and heart. It courses through the diaphragm (see Figure 2–6) and enters the abdominal cavity, where it connects to the stomach. The esophagus is usually in a flattened state, but can stretch to accommodate substances passing through it. The cervical (upper) esophagus consists of striated (voluntary) muscle, whereas the thoracic (middle) esophagus comprises a mixture of striated and smooth (involuntary) muscle in its upper region and purely smooth muscle in its lower region. The abdominal (lower) esophagus is composed of only smooth muscle. The esophagus is lined with a thick layer of mucosa, beneath which lies connective tissue and glands that secrete mucus to aid in the movement of substances through it.
The esophagus is bounded at its upper end by the upper esophageal sphincter (sometimes called the pharyngoesophageal segment, or PE segment) and at its lower end by the lower esophageal sphincter. These sphincters mark the entrance and exit of the esophagus and are operationally defined as zones of high pressure, rather than as precise anatomical entities. It is uncertain which structures are responsible for creating these high-pressure zones. Nevertheless, indirect evidence suggests that the cricopharyngeus muscles of the pharynx (considered by some to be part of the inferior constrictor muscles) are the primary contributors to the contraction–relaxation pattern of the upper esophageal sphincter (Hila, Castell, & Castell, 2001).
The upper end of the esophagus is positioned among several of the pharyngeal and laryngeal structures discussed in Chapters 3, 4, and 5. These structures are depicted in two different views in Figure 16–3. Figure 16–3A depicts the laryngeal area and the top of the esophagus (in its closed state) as viewed from above. Of particular interest in the context of swallowing are the pyriform sinuses and the epiglottic valleculae. The pyriform sinuses are cavities that are located near the back of the larynx and lateral to the aryepiglottic folds. The epiglottic valleculae (one vallecula on each side) are depressions located toward the front of the larynx on the lingual (tongue) side of the epiglottis and just behind the root of the tongue. Figure 16–3B shows the pharyngeal, laryngeal, and upper esophageal areas as viewed from the back, with the pharyngeal muscles intact (left side) and with those muscles removed and the pharynx open at the back (right side). The cricopharyngeus muscle (lower part of the inferior constrictor muscle) is shown to surround the region of the upper esophagus. Also shown in Figure 16–3B is the relationship of the esophagus to one pyriform sinus and one epiglottic vallecula, as well as the epiglottis, tongue, and velum.
The stomach is a large, saclike structure made up of smooth muscle, mucosa, and other tissue. It is on the left side of the abdominal cavity, against the undersurface of the diaphragm (see Figure 16–2). The stomach connects to the esophagus via the lower esophageal sphincter and to the small intestine via the pyloric sphincter. After a typical meal, the stomach holds about a liter of solid and/or liquid substance, although it can stretch to hold much more if necessary. Gastric juices in the stomach break up ingested substances so that they can be absorbed into the body through the stomach lining.
America Regains the Mustard Yellow Belt
Holy stomach full! Japan’s Takeru Kobayashi was the six-time hot-dog-eating world champion and ate 63 hot dogs and buns (6 more than his personal best) on July 4, 2007, at Coney Island, New York. With more than 50,000 people in attendance and in the glare of national television cameras, Kobayashi lost his crown to Joey Chestnut of San Jose, California. Sanctioned by Major League Eating, the world governing body of all stomach-centric sport, Nathan’s Famous International Fourth of July Hot Dog Eating Championship has been held each year on the 4th of July since 1916. In 2007, Chestnut put down an astounding 66 hot dogs and buns to set a new world’s record. On July 4, 2018, with ten wins already under his belt, Chestnut once again exceeded his personal best by ingesting 74 dogs and buns in just 10 minutes. And, yes, there is a women’s competition too. The women’s 2018 winner was Miki Sudo, who ingested 37 hot dogs to take home her fifth Nathan’s title in a row.
Figure 16–3. Two views of laryngeal and pharyngeal structures. The top figure (A) depicts the laryngeal area viewed from above. In particular, note the location of the pyriform sinuses (toward the back), esophagus (behind the larynx), and the epiglottic valleculae (toward the front, near the tongue root). The bottom figure (B) is a view from the back. Its left side shows the superior, middle, and inferior (thyropharyngeus and cricopharyngeus divisions) constrictor muscles intact. Its right side shows these muscles removed to reveal the pharyngeal and laryngeal regions (again, note the location of the pyriform sinus).
FORCES AND MOVEMENTS OF SWALLOWING
Although many of the structures that participate in swallowing are the same as those that are used for speaking, the forces and movements for the two activities are very different. In general, the forces are greater and many of the movements are slower during swallowing than during speech production.
To set the stage for understanding the forces and movements of swallowing, it is useful to begin by considering certain pressures associated with the resting state of the swallowing apparatus. These pressures, pointed out in Figure 16–4, are shown with the swallowing apparatus at rest at the end of a tidal expiration. As expected, the pressure in the oral cavity, which is coupled to the outside via the velopharyngeal-nasal airway, is zero (equal to atmospheric pressure) at rest. The other pressures are not zero and their values as shown in Figure 16–4 are approximations; some of them can range substantially.
The pressure within the esophagus is below atmospheric pressure (approximately −5 centimeters of water [cmH2O]). This pressure is negative because of pleural linkage—the link between a pulmonary apparatus that “wants” to contract and a chest wall that “wants” to expand (recall the spring analogy in Figure 2–4 representing the linked pulmonary apparatus and chest wall). This creates a negative pressure between the pleura (called pleural pressure; see Chapter 2), and that negative pressure is transmitted across the dividing wall to the esophagus.
Figure 16–4. Relevant pressures associated with the resting state of the swallowing apparatus. Oral pressure is zero (atmospheric), esophageal pressure is slightly negative, and stomach (gastric) pressure is slightly positive. Both the upper and lower esophageal sphincters exert high pressure that can range considerably in magnitude. It is especially important that the lower esophageal sphincter pressure remain higher than the pressure within the stomach (gastric pressure) so that the contents of the stomach do not reflux.
The pressure in the stomach (gastric pressure) is slightly above atmospheric pressure (approximately 5 cmH2O). This positive pressure is, in part, the result of the muscle tone exerted by the wall of the stomach. This pressure is also attributed to the hydrostatic properties of the abdomen.
In contrast to these relatively low esophageal and gastric pressures, the pressures within the upper and lower esophageal sphincters are high. These high pressures are attributable to the high tissue forces exerted by the sphincters. Although a typical range is 40 to 80 cmH2O in the resting state, their absolute magnitudes depend on the measurement approach used as well as a variety of physiological factors (Goyal & Cobb, 1981; Linden, Hogosta, & Norlander, 2007). Because the upper and lower esophageal sphincters exert such high pressures, these regions function like forcefully closed valves while at rest. In particular, it is important that the pressure in the lower esophageal sphincter be substantially higher than the pressure in the stomach. Otherwise, substances from the stomach may reflux (flow back) into the esophagus.
The act of swallowing is driven by both passive and active forces. Passive force comes from many sources, including: (a) the natural recoil of connective tissues (ligaments and membranes), cartilages, and bones, (b) the surface tension between structures in apposition, (c) the pull of gravity, and (d) aeromechanical factors. Active force results from the activation of breathing, laryngeal, velopharyngeal-nasal, and pharyngeal-oral muscles in various combinations. Their contributions to active force are described in Chapters 2, 3, 4, and 5, and are discussed here as they relate to swallowing, as are the forces exerted by the esophagus.
Forces and movements of swallowing can be described as they pertain to four phases of swallowing. These phases, illustrated in Figure 16–5, are the oral preparatory phase, oral transport phase (sometimes called the oral propulsive phase or oral transit phase), pharyngeal phase, and esophageal phase. They are used to describe the movement of a bolus (shown in green in Figure 16–5) through the oral, pharyngeal, and esophageal regions of the swallowing apparatus. Bolus is the word used to refer to the volume of liquid or the mass of solid substance being swallowed. The physiological events associated with each of these phases are described in the text below and summarized in Table 16–1.
The oral preparatory phase is depicted in the first panel of Figure 16–5. This phase begins as a solid or liquid substance makes contact with the structures of the anterior oral vestibule. The mandible has already lowered and the lips have abducted in anticipation of the swallow (Shune, Moon, & Goodman, 2016). What happens next largely depends on the nature of the substance to be swallowed.
Figure 16–5. Depiction of the oral preparatory, oral transport, pharyngeal, and esophageal phases of swallowing. The actions associated with each phase are summarized in Table 16–1.
If the substance is liquid, the mandible elevates and the lips adduct, forming an anterior seal to contain the bolus. The bolus is contained in the anterior region of the oral cavity by actions of the tongue and other structures, and held there momentarily (usually on the order of 1 second). The anterior tongue depresses and the sides of the tongue elevate to form a cup for the bolus. The bolus may be cupped in one of two ways, depending on the person. Some people hold the bolus with the tongue tip elevated and contacting the back surface of the maxillary incisors, and other people hold the bolus on the floor of the oral cavity in front of the tongue (dubbed “dipper” and “tipper” type swallows, respectively; Dodds et al., 1989). The back of the tongue elevates to make contact with the velum to form a back wall that separates the oral from the pharyngeal cavity and helps ensure that no substance can slip by and into the pulmonary airways. The velopharynx is open so that breathing can continue. Nevertheless, many people stop breathing momentarily at this point in the swallow (this is called the apneic interval) or even before the glass or straw reaches the lips (Martin, Logemann, Shaker, & Dodds, 1994; Martin-Harris, Brodsky, Price, Michel, & Walters, 2003; Martin-Harris, Michel, & Castell, 2005b). This apneic interval serves to reduce the risk of aspiration (defined as invasion of substances below the vocal folds).
These initial events are quite different when the substance to be swallowed is solid rather than liquid, primarily because solid substances need to be masticated (chewed) into smaller pieces and mixed with saliva before being transported toward the esophagus. Saliva is an important ingredient in this process because it moistens the solid substance to facilitate its transport; saliva also introduces enzymes that begin to break down the substance for digestion. Actions of the mandible (and teeth), lips, tongue, and cheeks grind and manipulate the solid substance into a cohesive bolus and position it on the surface of the anterior tongue. The lips may adduct (though this is not necessary) while the mandible moves to grind the bolus. During chewing, the mandible moves up and down, forward and backward, and side to side. This is in contrast to speech production, during which the mandible moves primarily up and down. The velum makes contact with the back part of the tongue to seal off the oral from the pharyngeal cavity and prevent the bolus from moving into the pharynx and larynx. The velopharynx is open during preparation of the bolus, and breathing may either continue or be interrupted by apnea (McFarland & Lund, 1995; Palmer & Hiiemae, 2003). The duration of the oral preparatory phase for solid substances may last from as short as 3 seconds, when chewing a soft cookie, to as long as 20 seconds, when chewing a tough piece of steak.
“Mmm, mmm, good!” Does this make you think of steamy, chunky soup? Or, better yet, freshly baked cookies just out of the oven? Maybe your imagination is so good that your mouth actually starts to water, which brings us to the point of this sidetrack: saliva. Saliva is produced by salivary glands and is critical to our ability to swallow and digest. Most saliva is swallowed alone (these are called “dry swallows”). During eating, saliva mixes with the food to moisten it for easier transport through oral, pharyngeal, and esophageal parts of the digestive tract and introduces enzymes that begin the digestive process. Do you have any idea how much saliva we produce? The answer is an amazing 1 to 2 liters of saliva every 24 hours! Although saliva production is continuous, its volume and content vary rhythmically; that is, saliva production has a circadian rhythm. Much less saliva is produced during sleep than during wakefulness. That’s good. Best to save that saliva for the cookie-eating waking hours.
At the end of the oral preparatory phase, the substance in the oral cavity is ready for consumption. Usually it is immediately transported back toward the pharynx (oral transport phase). There are choices at this point, however, including that the substance can be: (a) savored for a while by continued manipulation, (b) squirreled in the cheeks, or (c) expelled. The expulsion option is used when performing a sham feeding test to study the actions of the stomach in anticipation of receiving food.
The oral transport phase, also called the oral propulsion phase or oral transit phase, is shown in the second panel of Figure 16–5. From the ready position (either the “dipper” or “tipper” position for liquids), the bolus is transported back through the oral cavity. This is done by using the tongue tip to squeeze the bolus against the hard palate; then progressively more posterior regions of the tongue elevate and squeeze the bolus against the palate, moving the bolus back toward the pharynx. The tongue is an especially effective structure for moving and clearing the bolus because it behaves like a muscular hydrostat and can move and change shape in an almost infinite number of ways (see Chapter 5). The force needed to propel the bolus varies with bolus viscosity (the resistance offered by a fluid to flowing). The lips usually press together firmly (although this is not necessary) and the cheeks are pulled inward slightly to keep the bolus positioned over the tongue. At the same time, the velum begins to elevate and the upper esophageal sphincter is relaxing. The oral transport phase is short, lasting less than a second (Cook et al., 1994; Tracy et al., 1989).
The pharyngeal phase of the swallow is “triggered” once the bolus passes the anterior faucial pillars; however, the exact location of the trigger varies, depending on the bolus type and the age of the individual. During this phase, depicted in the third panel of Figure 16–5, several events occur rapidly and nearly simultaneously to move the bolus quickly through the pharynx while protecting the airway. This phase is under “automatic” neural control, so that once triggered, it proceeds as a relatively fixed set of events that cannot be altered voluntarily (except in the magnitude and duration of the pressures generated). These events occur within about half a second (Cook et al., 1994; Tracy et al., 1989) and include velopharyngeal closure, elevation of the hyoid bone and larynx, laryngeal closure, pharyngeal constriction, and opening of the upper esophageal sphincter, as described below.
During the pharyngeal phase, the velopharynx closes like a flap-sphincter valve by elevation of the velum and constriction of the pharyngeal walls. This closure is forceful (more forceful than for speech production) so as to prohibit substances from passing through the nasopharynx into the nose.
The hyoid bone and larynx move upward and forward as a result of contraction of extrinsic tongue muscles, with major contributions from the geniohyoid muscles to upward movement and the mylohyoid muscles to forward movement (Pearson, Langmore, & Zumwalt, 2011). (Recall from Chapters 3 and 5 that several extrinsic muscles of the tongue attach to the hyoid bone.) As the hyoid bone is pulled upward and forward, the larynx is pulled along with it by its muscular and nonmuscular connections to the hyoid bone. In fact, this group of structures is often called the hyolaryngeal complex because of these anatomical connections and the tendency for them to move as a unit. Elevation of the larynx also causes the pharynx to shorten.
Closure of the larynx for swallowing has been described as a folding of the laryngeal apparatus (Fink & Demarest, 1978) that forms a seal to the entrance of the trachea to protect the pulmonary airways. Closure occurs at multiple levels, which include the vocal folds, the ventricular folds, and the aryepiglottic folds and epiglottis. The arytenoid cartilages move medially and then tilt forward to touch the epiglottis and both the vocal folds and ventricular folds adduct firmly. The epiglottis is forced down over the laryngeal aditus like a trap door and serves as a first line of defense against substances entering the larynx and pulmonary airways. Both passive and active forces appear to be responsible for downward movement of the epiglottis during swallowing (Ekberg & Sigurjonsson, 1982; Fink & Demarest, 1978; VanDaele, Perlman, & Cassell, 1995). The passive force derives from backward movement of the tongue and upward and forward movement of the hyoid bone and larynx, which mechanically deflect the epiglottis backward and downward. Upward and forward movement of the larynx simultaneously contributes to airway protection by tucking the larynx against the root of the tongue and deflecting the trachea away from the digestive pathway. The active force is somewhat less certain (Fink, Martin, & Rohrmann, 1979; Ramsey, Watson, Gramiak, & Weinberg, 1955; VanDaele et al., 1995), but is argued to derive from contraction of the aryepiglottic muscles (and possibly from vertically ascending lateral fibers of the thyroarytenoid muscles), which purportedly pull the epiglottis downward to complete the seal of the laryngeal aditus (Ekberg & Sigurjonsson, 1982).
As the tongue propels the bolus into the pharynx, the pharynx undergoes segmental contraction (from top to bottom). The tongue root moves backward and the pharyngeal walls constrict to squeeze the bolus toward the esophagus. The bolus often divides at the epiglottis as it passes through the left and right epiglottic valleculae (lateral channels between the root of the tongue and the epiglottis) and into the left and right pyriform sinuses (recesses bounded by the pharynx and larynx), or it flows down one side or through the midline of the covered laryngeal aditus (Dua, Ren, Bardan, Xie, & Shaker, 1997; Logemann, Kahrilas, Kobara, & Vakil, 1989).
As all of these events are playing out, the upper esophageal sphincter is opening to allow the bolus to pass into the esophagus. Two sets of actions appear to contribute to its opening: (a) stretching of the upper esophageal sphincter by forward and upward movement of the hyolaryngeal complex (likely accomplished by activity of the mylohyoid, geniohyoid, and anterior belly of the digastric muscles), and (b) relaxing of the cricopharyngeus muscles (Omari et al., 2016).
The bolus is propelled through the pharynx to the esophagus during the pharyngeal phase by a combination of mechanical (structural) forces and aeromechanical forces. The mechanical forces consist of the tongue pushing the bolus back into the pharynx and the pharynx contracting segmentally against the tongue root, as just described. The aeromechanical forces are in the form of regional pressure changes that help to move the bolus along. Specifically, backward movement of the tongue and constriction of the pharyngeal walls serve to narrow the airway and reduce the airway volume, thereby causing the pressure to rise in that region. At the same time, elevation of the larynx and dilation of the upper esophageal sphincter lowers the pressure below the bolus. The pressure differential (higher pressure behind the bolus than in front of it) helps to drive the bolus toward its destination.
It is also relevant to mention that the pharyngeal phase of swallowing can be stimulated by pooling of saliva in the pharynx and can be initiated in the absence of oral preparatory and oral transport phases (Logemann, 1998). These swallows occur regularly throughout the day and night and are called nonbolus swallows, dry swallows, or saliva swallows.
The esophageal phase, the initial part of which is illustrated in the last panel of Figure 16–5, begins when the bolus enters the upper esophageal sphincter and ends when it passes into the stomach through the lower esophageal sphincter. This phase may last anywhere from 8 to 20 seconds (Dodds, Hogan, Reid, Stewart, & Arndorfer, 1973). At the same time the upper esophageal sphincter opens to allow the bolus to pass into the esophagus, the lower esophageal sphincter relaxes. The bolus is propelled through the esophagus by peristaltic actions (alternating waves of contraction and relaxation) of the esophageal walls. Peristaltic contraction raises pressure behind the bolus and relaxation lowers pressure in front of the bolus, creating the pressure differential needed to propel it toward the stomach. The nature of the peristaltic action varies somewhat depending on the nature of the bolus (liquid or solid), body position (relation of esophagus and bolus to gravity), and other factors. When a substance is left behind following the primary peristalsis, it is cleared by subsequent peristaltic action (called secondary peristalsis). Although the esophagus usually transports substances toward the stomach, it can also transport substances or gas away from the stomach (as in the case of vomiting or burping).
Although the phases of swallowing are described above as though they are discrete and occur one after the other, in fact they overlap substantially. When a person is eating a solid substance, for example, preparation of part of the bolus in the oral cavity may continue while another part of the bolus moves into the pharyngeal area, as illustrated in Figure 16–6. This partial bolus may remain in the epiglottic valleculae as long as 10 seconds before it merges with the rest of the bolus and the pharyngeal transport phase of the swallow is triggered (Hiiemae & Palmer, 1999).
Overlap of phases is also apparent if swallowing is viewed in relation to the actions of individual structures, rather than in relation to the status of the bolus. Whereas the traditional description of swallowing (used in this chapter) focuses on preparation and transport of the bolus to define the phases of swallowing, there are schema that try to segment physiological events along somewhat different conceptual lines and to categorize them across different levels of observation (Martin-Harris et al., 2005b). This view of the swallowing process focuses on coordination of temporal events across structures. Figure 16–7 is an example of this type of conceptualization. Starting at the left side of the figure, the lips are abducted and the mandible and tongue tip are depressed to allow the bolus to enter the oral vestibule. Moving rightward from there, many structures (not an exhaustive list) take action to move the bolus back toward the esophagus and to protect the airways. Note that this figure presents a general representation of the sequence and relative time of the onsets of various events; actual measures of these events reveal substantial variability within and across people (Molfenter & Steele, 2012). Schema such as these that are based on cross-structure analyses reveal the overlapping elements of swallowing behavior and interactions among its components and hold promise for developing a better understanding of the swallowing process.
Figure 16–6. An illustration of eating, in which part of the bolus continues to be chewed while another part moves into the epiglottic valleculae, where it may remain for many seconds. This is an example of why the conceptualization of swallowing as comprising discrete phases can be problematic.
Protection of the pulmonary airways during swallowing depends, in large part, on the coordination of breathing and swallowing. Without such coordination, inspiration might occur at the same time a substance is being transported through the pharynx and that substance might be “sucked” through the larynx into the pulmonary airways (aspiration). This is avoided by closing the larynx (at multiple levels, as described above), an action that arrests breathing for a brief period during the swallow.
One Tug and Two Consequences
Breathing and swallowing cooperate in healthy individuals to prevent unwanted substances from entering the pulmonary airways. This cooperation can be more difficult with certain diseases. Chronic obstructive pulmonary disease (COPD) is one of these. When advanced, COPD expands the pulmonary apparatus, and the diaphragm rides low and flat because air is trapped in the alveoli and airways. The abnormal positioning of the diaphragm has two potential consequences for swallowing. One is that a downward tug is placed on the larynx that tends to abduct the vocal folds and diminish their ability to protect the pulmonary airways. A second possible consequence is that the same downward tug lowers the laryngeal housing and tethers it from below. This means that the larynx may have difficulty moving up during a swallow because it has farther to go and because it must work against the downward pull of the diaphragm. It’s no wonder that many people with advanced COPD also have problems with their swallowing.
Figure 16–7. Schematic representation of the initiation of actions of several structures during a swallow. This is not meant to represent a fixed sequence or timing of actions (because they can differ across swallows and individuals, depending on many variables), but, rather, to illustrate that swallowing is a continuous physiological process that cannot be divided into a strict set of phases. Figure designed in collaboration with Rosemary Lester-Smith, PhD, CCC-SLP and based on Langmore (2001).
The risk of aspiration appears to be further reduced by timing the swallow to occur during the expiratory phase of the breathing cycle. During single swallows, the most common pattern is expiration-swallow-expiration; that is, expiration begins, the swallow occurs (accompanied by apnea), and then expiration continues (Martin et al., 1994; Martin-Harris, 2006; Nishino, Yonezawa, & Honda, 1985; Perlman, Ettema, & Barkmeier, 2000; Selley, Flack, Ellis, & Brooks, 1989; Smith, Wolkove, Colacone, & Kreisman, 1989). This pattern, illustrated in Figure 16–8, is the predominant one for swallowing over a broad range of bolus volumes and consistencies and under a variety of serving conditions, such as presenting a liquid bolus with a syringe, drinking water from a cup or straw, or eating a solid substance (Preiksaitis & Mills, 1996; Wheeler-Hegland, Huber, Pitts, & Sapienza, 2009). This appears to be a protective mechanism for potentially “blowing” any foreign substance away from the pulmonary airways. Swallowing during expiration is associated with a reduced risk of aspiration in people with various impairments (Steele & Cichero, 2014) and is the basis for part of a training protocol used to improve breathing-swallowing coordination for clients at risk for aspiration (Martin-Harris, Garand, & McFarland, 2017). Nevertheless, it is interesting to note that, even in healthy people, not every swallow is followed by expiration, and that some healthy individuals occasionally inspire immediately after a swallow. This is particularly prevalent in people over age 65 years (Martin-Harris et al., 2005a). It is also possible for healthy individuals to swallow voluntarily during the inspiratory phase of the breathing cycle (Ulysal, Kizilay, Ünal, Güngor, & Ertekin, 2013).
Figure 16–8. Typical breathing pattern during a single swallow, featuring a period of apnea (cessation of breathing). This pattern is described as expiration–swallow (accompanied by apnea)–expiration.
Although the apneic interval during swallowing typically lasts about 1 second, it can range from less than a second to several seconds (Klaun & Perlman, 1999; Martin et al., 1994; Martin-Harris et al., 2003, 2005a; Palmer & Hiiemae, 2003; Perlman et al., 2000; Preiksaitis & Mills, 1996). In some people, the duration of the apneic interval is influenced by variables such as bolus volume (Preiksaitis, Mayrand, Robins, & Diamant, 1992). Nevertheless, most of the variability in apnea duration can be attributed to variability in the onset of apnea relative to the eating or drinking event. For example, one person may stop breathing as the food or drink is approaching the mouth, whereas another person may continue to breathe until immediately before the larynx begins to elevate for the pharyngeal transport phase of the swallow (Martin et al., 1994).
The apnea associated with swallowing can cause dyspnea (breathing discomfort) and a subsequent increase in ventilation, even in healthy people (Lederle, Hoit, & Barkmeier-Kraemer, 2012) and can be particularly uncomfortable and challenging in people with pulmonary disease (Hoit, Lansing, Dean, Yarkosky, & Lederle, 2011). When healthy people experience high respiratory drive (such as might occur during exercise or at high elevations), they tend to shorten the apneic interval during swallowing (Hårdemark Cedborg et al., 2010; in this study, high respiratory drive was created by breathing gas with a greater-than-usual amount of carbon dioxide). Shortening apnea in this way likely helps to minimize dyspnea. Another way that people apparently minimize dyspnea is to breathe frequently during a series of swallows, such as when drinking a glass of water without stopping (Gürgor et al., 2013; Lederle et al., 2012).
Swallowing occurs at lung volumes that are almost always larger than the resting expiratory level (that is, the end-expiratory lung volume associated with resting tidal breathing), usually on the order of 10% to 20% larger (Lederle et al., 2012; McFarland et al., 2016; Wheeler-Hegland et al., 2009; Wheeler-Hegland, Huber, Pitts, & Davenport, 2011). This lung volume range is one in which the passive (recoil) pressure of the breathing apparatus is positive, on the order of 5 to 10 cmH2O (see relaxation characteristic in Figure 2–18), and the tracheal pressures associated with swallowing generally fall in this recoil pressure range (Gross et al., 2012). The fact that swallowing occurs at lung volumes that are larger than the resting size of the breathing apparatus, but still within the midrange of the vital capacity, appears to have several advantages. To begin, because swallows are produced at lung volumes where the alveolar pressure is positive, post-swallow expirations are easily driven by the recoil pressure of the breathing apparatus. Also, because swallows are produced at lung volumes that are only moderately large, there is no need to exert inspiratory muscular pressure to brake excessive positive recoil pressure that prevails at large lung volumes. Finally, by avoiding larger-than-necessary lung volumes, the abductory force exerted on the vocal folds by the descent of the diaphragm is minimized (i.e., “tracheal tug,” see Chapter 3). This optimal lung volume range has been incorporated as a component of a training protocol for clients with abnormal breathing-swallowing patterns who are at risk for aspiration (Martin-Harris et al., 2017).
People with chronic obstructive pulmonary disease (COPD) have other problems besides the expanded pulmonary apparatus and flattened diaphragm described in the previous sidetrack. One particularly troublesome problem is dyspnea (breathing discomfort), a condition that causes people with COPD to avoid activities that compete with their already strong drive to breathe. Perhaps surprisingly, eating is one of those activities. Most healthy people have no idea that they hold their breath when they swallow. But for people with severe COPD, it’s quite a different story. They are often acutely aware of the competition that goes on between eating and breathing. The need to breath hold during the swallow causes “air hunger” and makes eating and drinking unpleasant chores rather than pleasurable pastimes. Do everything you can to avoid COPD and your life will be happier. Have you quit smoking yet?
The neural control of swallowing is complex and not completely understood. Nevertheless, studies of humans and animals have offered important insights into how swallowing is controlled by the nervous system. Some of the salient features of that control are discussed below as they relate to the participation of the peripheral and central nervous systems.
Role of the Peripheral Nervous System
Nearly all the structures involved in swallowing are the same as those involved in speech production (the most notable exceptions being the esophagus and stomach). Those structures that participate in both swallowing and speech production are innervated by the spinal nerves and cranial nerves described in previous chapters, as summarized in Table 16–2. As can be seen in the table, half of the cranial nerves (6 of 12) and most of the spinal nerves (22 of 31) are potential participants in swallowing (and speech production). The cranial nerves are involved in swallowing through their innervation of the lips, mandible, tongue, velum, pharynx, and larynx, whereas the spinal nerves are primarily involved in breathing and its cessation as they relate to swallowing.
Peripheral innervation of the esophagus differs along its length. The upper (cervical) region is made up of striated muscle, the type of muscle found in other structures of the swallowing apparatus (lips, mandible, tongue, velum, pharynx, larynx, and breathing structures). The cervical region, which includes the upper esophageal sphincter, is innervated by the recurrent branch of the vagus nerve (cranial nerve X), the same branch that innervates most of the intrinsic muscles of the larynx. Thus, the same peripheral nerve is responsible for the simultaneous actions of closing the larynx and opening the upper esophageal sphincter. This means that there is a strong neural link between actions that serve to protect the airway and actions that allow substances to pass into the esophagus. This strong link has obvious advantages for the coordination of the normal swallow, but also has the disadvantage that damage to the recurrent branch of the vagus nerve can have serious consequences for both voice production and swallowing (Corbin-Lewis & Liss, 2015).
In lower regions of the esophagus, where smooth muscle intermingles with striated muscle (thoracic esophagus) and where smooth muscle is the only type of muscle present (abdominal esophagus), a different form of neural control operates. This control comes from the autonomic nervous system, which is generally considered to be under automatic (as opposed to voluntary) control. The autonomic nervous system has two parts, the parasympathetic and sympathetic subdivisions. The parasympathetic subdivision is important for maintaining gastrointestinal motility so that a swallowed substance moves through the esophagus easily and quickly. In contrast, the sympathetic subdivision, best known for its importance in fight-or-flight responses to stressful situations, tends to inhibit gastrointestinal motility. This is one reason why gastrointestinal problems are associated with physical and emotional stress. Many of the nerve fibers of the autonomic nervous system travel with the vagus nerve.
Table 16–2. Summary of Motor and Sensory Nerve Supply to the Breathing Apparatus, Laryngeal Apparatus, Velopharyngeal-Nasal Apparatus, and Pharyngeal-Oral Apparatus
Note. Spinal nerves are designated by their segmental origins (C = cervical, T = thoracic, L = lumbar). Cranial nerves are V (trigeminal), VII (facial), IX (glossopharyngeal), X (vagus), XI (accessory), and XII (hypoglossal). This information is also available in Tables 2–2, 3–1, 4–1, and 5–2.
aIncludes intrinsic, extrinsic, and supplementary laryngeal muscles
b Sensory innervation of extrinsic and supplementary laryngeal muscles includes other cranial nerves, such as V and VII.
Role of the Central Nervous System
Although swallowing and speech production are executed using many of the same peripheral nerves, central nervous system control of these two activities is quite different. This means that a given structure, such as the tongue, is under one form of neural control during swallowing and under another form of neural control during speech production. Because of this, it is possible to have central nervous system damage that impairs the function of a structure for speech production but not swallowing, and vice versa. There are two major regions within the central nervous system that are responsible for the control of swallowing. One is in the brainstem and the other is in cortical and subcortical areas.
The brainstem center is located primarily in the medulla, the part of the brainstem that is contiguous with the uppermost part of the spinal cord. Two main groups of brainstem neurons participate in swallowing: one group that appears to be primarily responsible for triggering the swallow and shaping its temporal pattern and another group that appears to allocate neural drive to the various motor nerves that participate in swallowing (Jean, 2001). This brainstem center has primary control over the more automatic phases of swallowing (pharyngeal and esophageal phases).
Many cortical and subcortical regions contribute to the generation and shaping of swallowing behaviors. The most consistent findings point to contributions of the primary motor and sensory areas of the cortex, anterior cingulate cortex, and insular cortex, with probable contributions from basal ganglia, thalamus, and cerebellum (Humbert & Robbins, 2007). Activity in these areas has a strong influence over the control and modulation of the more voluntary phases of swallowing (oral preparatory phase, including mastication, and oral transport phase). However, studies of people with cortical damage from strokes indicate that the cortex may also exert influence over what have traditionally been thought of as the automatic phases (pharyngeal and esophageal phases) of swallowing (Martin & Sessle, 1993).
Afferent input is critical to the generation of a normal swallow. The sources of afferent input are many and include, but are not limited to, information related to: (a) muscle length and rate of length change, (b) muscle tension, (c) joint position and movement, (d) surface and deep pressures, (e) surface deformation, (f) temperature, (g) taste, and (h) noxious stimuli. Afferent activity is generated by receptors in the swallowing apparatus and sent to the brainstem, where such activity may trigger the motor output required to elicit the pharyngeal phase of the swallow or it may modulate the motor output to accommodate a larger-than-expected bolus. Afferent activity may also be sent on to subcortical regions (such as the thalamus) or cortical regions (such as the sensorimotor cortex) where it may be consciously perceived. Often the perception is a pleasant experience, such as savoring the flavor and texture of ice cream, or it may be unpleasant (see sidetrack “Sphenopalatine Ganglioneuralgia” and Figure 16–9).
Sphenopalatine Ganglioneuralgia
Boy, that sounds like something you wouldn’t want to meet in the dark. But it comes from something really good. As a child (or even as an adult) you may have said the phrase, “I scream, you scream, we all scream for ice cream.” Scream has a meaning of anticipation in this context, but it can also have a meaning of hurting. You know the feeling. You take a bite of ice cream and momentarily hold it against the roof of your mouth before you swallow it. Then suddenly you get an intense, stabbing pain in your forehead. What’s up? The pain is caused as your hard palate warms up after you made it cold. Cold causes vasocon-striction (reduction in blood vessel diameter) in the region, which is followed by rapid vasodilation (increase in blood vessel diameter). It’s the rapid vasodilation that hurts and gets your attention. The technical term for this pain is sphenopalatine ganglioneuralgia. The common term (and the one more easily pronounced) is “brain freeze.” Fortunately, the pain lasts only a few seconds. Be thankful. There’s all that ice cream still waiting to be eaten.
Figure 16–9. Sphenopalatine ganglioneuralgia (or so-called brain freeze) caused by placing something cold against the roof of the mouth. Image provided courtesy of the University of Cincinnati. Reproduced with permission.
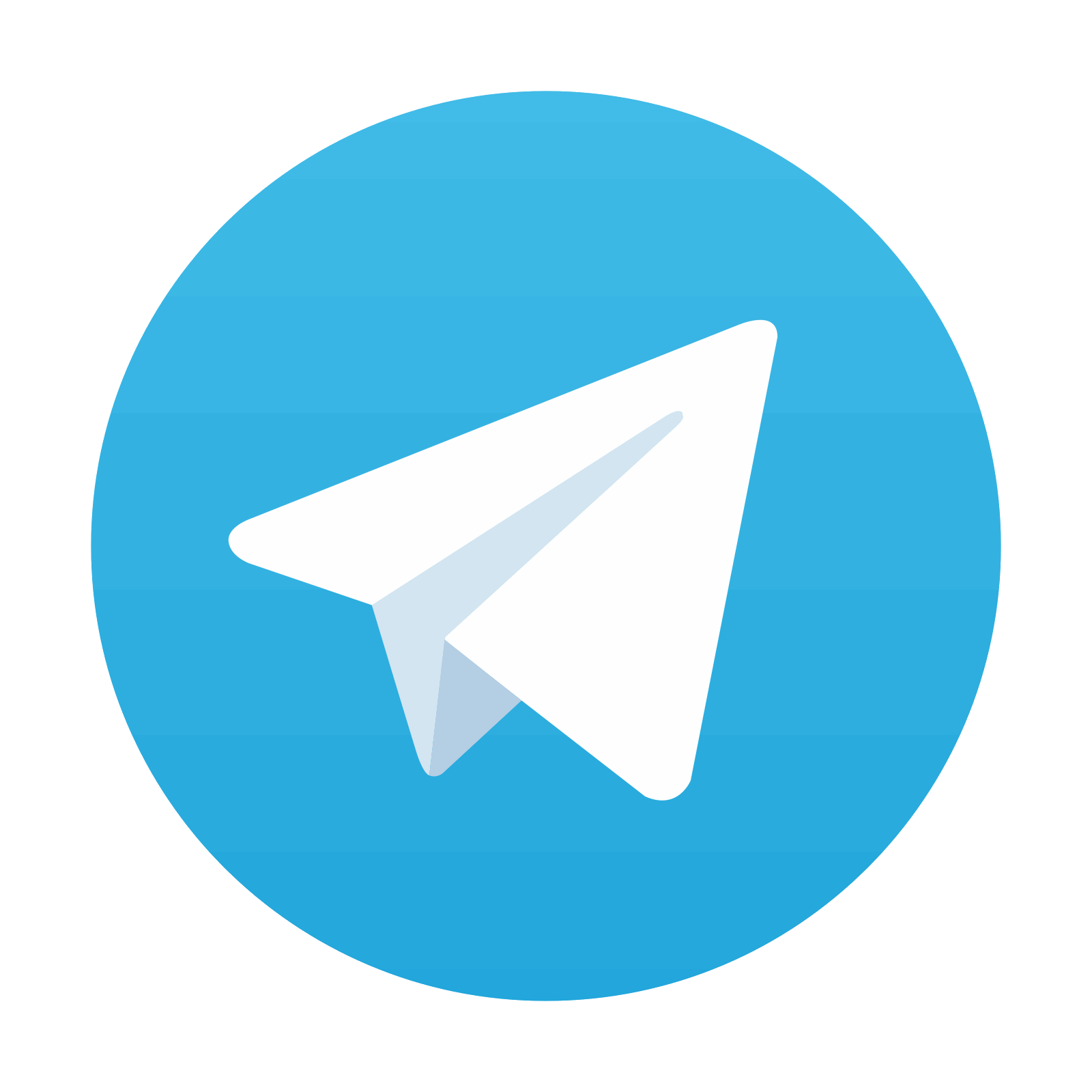
Stay updated, free articles. Join our Telegram channel

Full access? Get Clinical Tree
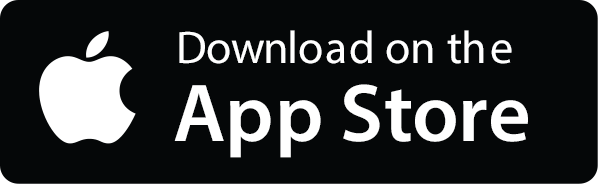
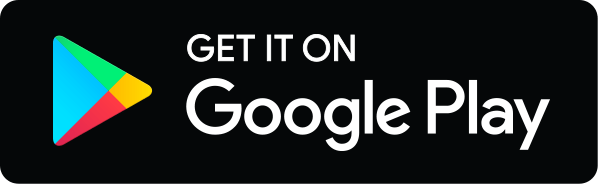