High-technology vitreous surgery requires intensive and continuous attention to the equipment and operating environment. Equipment, supplies, training, staffing, maintenance, and storage of the equipment are ultimately the surgeon’s responsibility. The requirements for effective high-consequence surgery should outweigh logistical considerations. Blaming problems on equipment, companies, nurses, technicians, administrators, or managed care is irresponsible and ineffective.
Vitreoretinal surgery has undergone many engineering advances in the four decades since it was first introduced. Most of the important developments resulted from an intuitive rather than a physics-based design approach. By contrast, medical school curriculums emphasize a biology-based approach to diagnosis and therapy. The intent of this chapter is to provide a physics and engineering framework for understanding the essential techniques and technologies used in vitreoretinal surgery today.
There are many similarities between the task domain of the pilot and of the surgeon. Pilots are required to understand the engineering principles behind the propulsion, hydraulic, pneumatic, avionics, and electrical systems, while surgeons often use tools and machines with little or no understanding of how they work. The physics of flight is very complex but is relatively well understood by both engineers and pilots. Tissue cutting, turbulence, friction, state change in materials, and fracture propagation are so complex that a precise mathematical description of the underlying physics is impossible. Nevertheless, valid assumptions about the physical principles involved and estimation of the mathematical parameters can and should be made. Vitreoretinal surgery requires a wider variety of techniques and technologies than cataract surgery, because it is used to treat a broader array of disease entities and anatomic configurations using a greater array of technologies. Knowledge of the engineering concepts involved in vitreoretinal surgery can facilitate better selection of tools and techniques as well as infusion, suction, duty cycle, and cutting rate parameters. Surgeons who understand vitreoretinal surgical systems can problem solve, troubleshoot, improvise, and perform better and faster in the operating room (OR). A surgeon should be able to set up all machines, set all parameters and modes, and connect, prime, and test all tools without the help of a nurse or technician. This knowledge reduces dependency on OR personnel and resultant anxiety if key people are not available.
OPERATING ROOM ENVIRONMENT
Scheduling
An attempt should be made to schedule vitrectomies when the surgeon and team are not too rushed or heavily burdened mentally. If this means early, late, day-off, or weekend surgery, it must be done. Some surgeons become tense if they are heavily committed for time following their surgery time. As some vitrectomies can be lengthy, especially during the learning phase, time must be available to facilitate concentration on the surgery rather than a subsequent event. A designated OR is preferable to ad hoc room utilization as it facilitates equipment accessibility, maintenance, and stocking and storage of disposables.
Instrument Storage
The approach in which instruments are assembled from various sources just prior to the case is inadequate for high-technology vitreous surgery. It is not optimal to share these specialized instruments with other surgeons and procedures. A tray that is equipped for all basic vitreous, retinal, and microsurgical methods should be prepared and stored together. The removal of dense lenses, intraocular lenses (IOLs), and large intraocular foreign bodies through the limbus requires that the appropriate instruments be present on the basic vitrectomy tray. Similarly, scleral buckling techniques may be required and probably should not dictate the use of a second tray. An identical sterile backup tray should be available at all times in the OR or adjacent storeroom.
All fragile instruments such as intraocular scissors, forceps, contact lenses, and the like must be kept sterile at all times in transparent peel-packs stored in well-labeled trays, cabinets, or boxes. These instruments are best stored in a lockable cabinet in the OR or a movable cabinet that is taken to the OR before use. They should be stored in specific places in the cabinet to permit daily inventory control and maintenance. If not stored in a single cabinet, the instruments tend to become lost and unavailable at a critical time in vitrectomy.
All disposable materials such as packs, patient drapes, microscope drapes, sutures, needles, IOLs, gases, and tubing sets should be stored in sufficient numbers in a specific place near the OR. In this way, faulty or inadvertently contaminated materials and instruments can be instantly replaced without having to be ordered from a central supply area. The many steps in a complex procedure should not be delayed while waiting for instruments or materials to be delivered.
A fast para-acetic acid (Steris) and/or gas plasma (Sterad) as well as a fast cycle steam autoclave should be immediately available to the OR personnel to reduce case turnover time as well as resterilization of inadvertently contaminated equipment if sufficient backups are not available.
Presurgical Equipment Testing
All equipment should be set up and tested prior to the administration of anesthesia. If needed vitrectomy, lensectomy, phacoemulsification, endophotocoagulation, operating microscope, or scissors equipment is not functional, the case must be postponed. Infusion fluid should be run copiously through all tubing to remove any bubbles or particulate material. Only after all equipment is tested and the surgeon is present can the team begin local or general anesthesia.
Operating Room Personnel
Preferably, a single surgical technician or nurse should be responsible along with the surgeon for all instruments as well as for the ordering, maintenance, and inventory of disposable materials. Preferably, this individual should also function in the office environment to enhance information transfer, efficiency, and patient confidence. This same individual can assist in the recording and compilation of preoperative, intraoperative, and postoperative data for outcome testing, medical records, and billing. Participation in the follow-up care provides personal evidence of the impact of surgical success and failure. This individual should then be in command of the remainder of the team and should be responsible for backup personnel in their absence. A friendly, cooperative atmosphere with a sense of humor is conducive to the team-play attitude, which is as necessary in the OR as it is for a successful sports team. This is preferable to a tense, angry, chauvinistic, find-someone-to-blame attitude unfortunately so prevalent in ORs.
Video Recording
While certain cases may have teaching value, video recording should not become a time- and concentration-consuming consideration in the OR. Centering the microscope and producing the best video opportunity should not consume costly labor dollars or a surgeon’s mental focus. At best, it is a moderately efficient way to teach and is often used for promotional purposes. 3-CCD cameras provide better color purity and image quality than 1-CCD cameras but require over three times as much light. Firewire (IEEE 1394) or DVCAM is currently the optimal connection scheme between the camera and the digital recording systems. Recording can be on hard drives or DVDs. Currently, the best image quality is obtained with AVI files, although MPEG is a reasonable alternative for DVDs as it requires less storage space. Direct-to-edit (DTE) systems are far more efficient than systems requiring capturing the video at a time subsequent to the surgical procedure. DTE permits the first review of the video using fast forward rather than normal speeds.
Tissue Cutting
An external force placed on an object will cause it to move, unless the object is constrained. A force placed on a constrained object will cause an internal reaction called stress that resists the external force. Tensile (pulling) and compressive stresses occur normal (perpendicular) to the cross-sectional plane of an object. Guy wires on a suspension bridge are subject to tensile stress while the road surface is subject to compressive stress by a traveling car. Forces that act in the plane as opposed to normal to the plane are called shear stress and can be identified as transverse or torsional (twisting). A rivet between two sliding metal plates may be subject to transverse shear. The drive train of a car has a number of torsional stresses when the throttle is rapidly depressed.
Force placed on a constrained object induces deformation in relation to the amount, duration, and speed of onset of the external force. The proportion of the amount of deformation per unit length is known as strain. Tensile and compressive stresses will cause a lengthening or shortening of an object. Transverse shear stress causes bending, and torsional shear stress causes a twisting deformation (Fig. 2.1). Relatively small amounts of stress cause small amounts of strain. After the stress is removed, the object will return to its original size and shape in a process known as elastic strain. Increasing amounts of stress will eventually cause a permanent deformation in the object that persists after the stress is removed. In material such as steel, the permanent deformation is caused by changes in the crystalline structure of the metal. Eventually, small cracks appear that interconnect, leading to rupture and failure of the metal.
Figure 2.1 Force placed on a constrained object induces deformation in relation to the amount, duration, and speed of onset of the external force.
Biological tissues, particularly in the eye, display more complicated characteristics. An externally applied force can cause deformation, with the possibility of elastic strain. With continuing force, a “flow” may occur, such as that seen in a viscous fluid. This combination of elasticity and viscous flow is termed viscoelasticity. For example, it is common for the scleral buckling effect from a sponge exoplant to appear larger 1 or 2 days later. This is caused by viscoelastic changes in the sclera.
As noted above, the remarkable complexity of tissue cutting prevents an exact physics-based understanding and mathematical representation. The author has found it useful to define several types of tissue cutting in order to develop and utilize more effective techniques and devices: elongation, shear, sharpness, inertial, vaporization, and enzyme-assisted. Ideal cutting can be defined as separating tissue into two pieces without producing any remote forces or physical or chemical effects.
Elongation simply means to apply force to tissue or a tissue interface until the tissue fails, tears, or breaks. This is analogous to the methodology used in tensile strength testing. Membrane peeling is an example of this mode of action. Epiretinal membranes (ERMs) are thought to be approximately 100 times stronger than retina, which limits the application of this method to cases with low adherence between the retina and the membrane.
Shear can be defined as moving two square-edged cutting edges past each other with the target tissue interposed. Scissors implement shear type cutting with nonparallel blades that push the tissue forward as the blades close. This concept can be referred to as an “exclusive shear.” Scissors cut at a point that advances toward the tips with blade closure. Vitreous cutters also work primarily by shear. However, in contrast to scissors, vitreous cutters cut on a line rather than a point. Vitreous cutters utilize parallel cutting edges and, therefore, do not produce the “squeeze out” force produced by scissors. Vitreous cutters may be termed “inclusive” shears. Vitreous cutters require a pressure gradient across the port to cause fluid or tissue to move into the port. The actual cutting edges are recessed from the outside diameter of the cutter by the thickness of the outer “needle” wall. Tissue larger than the diameter of the port must be elastically deformed to pass through the port.
Sharpness can be defined as high pressure per unit area created by a blade with a thin cross section. Knives work by sharpness, while scissors are never “sharp” or “dull.” It is not known if microscopic surface roughness facilitates cutting or increases undesirable tissue displacement. The tip of the microvitreoretinal (MVR) blade, although originally developed for making the sclerotomies (Developed by Steve Charles, September 1976), is occasionally used to incise intraocular tissue including ERM. Angled knives have been developed to delaminate ERMs but have not been widely adopted because of the risk of iatrogenic retinal breaks.
Inertial cutting utilizes a rapidly moving cutting element impacting against tissue at rest and is the mode of action of the phacoemulsifier. The term “emulsification,” however, is inaccurate as this refers to overcoming surface (interfacial) tension to produce small droplets of a liquid otherwise immiscible in water. It is probable that vitreous cutters do not achieve velocities sufficient for significant inertial cutting effects.
Lasers and the peak electron avalanche knife (PEAK) work by producing vaporization of tissue, which inherently produces bubbles and acoustic (remote, mechanical) effects. Colloquial terms such as “ablation” and “disruption” are misleading as they are not physics-based terms. Lasers produce power densities sufficient to vaporize tissue or water by utilizing spatial or temporal coherence. Temporal coherence means that power is delivered in a very short interval, whereas spatial coherence means the energy is delivered to a very small volume. Spatial coherence inherently produces a small volume of tissue vaporization. Therefore, tissue cutting is dependent upon a contiguous line of spots or propagation of a tear that occurs with Yittrium Aluminium, garnet (YAG) laser capsulotomy. ERMs and vitreous are very elastic as well as resistant to fracture propagation. As a result of these properties, it requires very high-power densities to produce significant tissue cutting. High-power densities produce many bubbles as well as a propagating acoustic pressure wave, which can damage tissue remote to the intended site. The erbium YAG laser requires shielding from the retina, produces bubbles, requires vitrectomy system–like fluidics, and removes tissue at a very slow rate (1). Femtosecond lasers used for LASIK flap creation and other anterior segment applications utilize very high temporal coherence and inherently broadband (white) light but are not applicable to ERM removal.
Enzyme-assisted approaches to vitreoretinal surgery such as microplasmin-assisted vitrectomy have been studied for over three decades but have not been shown to be safe and effective at this time. Enzymatic methods have been directed at liquefying the vitreous or for separation of the posterior vitreous cortex from the retina (2). Problems include the following: (a) potential for damage to the retina, lens, or zonules; (b) inflammation; (c) potential for prion, viral, or bacterial contamination from biological materials; (d) the need to inject the enzyme at least 30 minutes before surgery; and (e) complicated, costly preparation processes. Autologous plasmin enzymes are obtained from the patient’s own plasma and, thus, will not have prion or viral contamination and appear to be safe with respect to damage to ocular tissues.
Surgical Fluidics
A pressure difference across the suction port of a vitrectomy instrument is necessary to cause substances to enter the port. The term “passive egress” is incorrect because there is no physical difference between transorifice pressure (TOP, delta P) achieved by high infusion pressure and that achieved by negative pressures (vacuum) on the inside of the port. Semirigid materials such as ERM and lens nucleus must be deformed to pass through a suction port in response to a TOP gradient. Markedly low TOP will prolong the process of vitreous removal; large gradients create vitreoretinal traction and retinal breaks.
In general, a maximum suction setting of 150 mm Hg should be used for 20-gauge systems and 650 mm Hg for 25-gauge systems when removing vitreous. The lowest suction force that enables vitreous removal in a reasonable time should be used to reduce vitreoretinal traction. The safest way to clear air lock and plugged tubing is by flushing the system outside the eye and not by increasing the suction force. After the vitreous has been removed, higher suction force can be used to carefully remove adherent or previously delaminated ERMs. Suction-induced vitreoretinal traction is a key factor in the production of intraoperative retinal breaks. A baseline incidence of aphakic-like retinal detachments will exist as long as vitreous is removed using suction force. Factors other than suction force such as lattice degeneration, preexisting retinal breaks, and vitreous incarceration in the sclerotomies also cause postvitrectomy retinal detachments.
Infusion Fluidics
Pars plana vitrectomy requires both infusion and aspiration; there are many parallels between infusion and aspiration fluidics because they are influenced by the same physical principles. Resistance to fluid flow is determined by the internal diameter of a lumen, length of tubing, and flow restrictions in the cannula. Fluidic resistance is proportional to the fourth power of the diameter (Hagen-Poiseuille equation) and linearly related to the length. The impact of diameter is significant because of the fourth-power relationship and clinically relevant because of the transition from 20-gauge (0.89 mm) to 23-gauge (0.75 mm) or 25-gauge (0.5 mm) technology in recent years.
The resistance of the infusion cannula is more than that produced by 84 inches of connected tubing. Ohm’s law (voltage = current × resistance [E = IR]) is mathematically equivalent to Ohm’s law for fluid flow (pressure gradient = flow × resistance). Fluidic resistance is an advantage in the aspiration system. By contrast, fluidic resistance is a disadvantage in the infusion system because it produces a pressure drop between the infusion pressure and the intraocular pressure (IOP), which must be compensated for. The pressure drop occurs only during flow and increases with higher flow rates (Fig. 2.2). It is typically 20 mm Hg or more during core vitrectomy. Conversely, the infusion pressure is equal to the IOP when there is no flow, that is, during membrane peeling, scissors segmentation or delamination, and endophotocoagulation. The highest flow rate and subsequent pressure drop occurs with the 20-gauge fragmenter, excessively low cutting rates, and nontapered extrusion cannulas.
Figure 2.2 Intraoperative IOP is determined by infusion pressure that is reduced by resistance through infusion system while flow is occurring minus vacuum level at console reduced by resistance in suction system including cutter port intermittent closing.
Effects of Low IOP
Excessively low IOP during vitrectomy is common in part because of undue concern about the effects of higher pressure. Low IOP causes pupillary constriction for unknown reasons; miosis often results in a need to perform potentially complication-producing techniques such as using iris retractors and injecting intraocular epinephrine. Low IOP permits deformation of the corneal dome from the force of a handheld irrigating contact lens; the author prefers an IOP of 45 mm Hg unless there is low arterial perfusion pressure as occurs in children or in some instances with general anesthesia. The most significant complication of low IOP is bleeding from resected vessels, most commonly in diabetic traction retinal detachment cases or those with elevated neovascularization. Suprachoroidal hemorrhage during vitrectomy is virtually always iatrogenic, caused by inadvertent suprachoroidal infusion with secondary shearing of the short posterior ciliary vessels. Hypotony is 100% preventable during vitrectomy, which is not the case with cataract surgery because of the need to open the eye for IOL implantation. Suprachoroidal infusion is preventable by careful attention to surgical technique during infusion cannula placement and rapid recognition of displacement of the infusion cannula into the suprachoroidal space.
Effects of High IOP
Excessively high IOP during vitrectomy can lead to the occlusion of the central retinal artery and corneal edema. Corneal edema is more likely to occur when the endothelial cell count is low as a result of previous intraocular surgery, trauma, or Fuchs’ dystrophy. Corneal edema occurs almost instantaneously when the IOP is excessive but takes substantially longer to resolve when the IOP is normalized. Fortunately, corneal edema is an early warning sign of excessive IOP before the retinal vessels come into view.
Once the retinal vessels are in view, the retinal vessels can be monitored for interrupted flow.
Intentionally elevated IOP is a useful technique to control bleeding when segmenting or delaminating vascularized ERMs, resecting scar tissue, or removing a subretinal choroidal neovascular membrane. Rapid foot pedal control is essential when using transient high IOP to control bleeding.
Safety Precautions for Infusion Cannula Placement
Wound construction is discussed extensively in another tutorial but several safety points are important to emphasize. The infusion cannula must be inserted in a relatively firm eye so that it penetrates completely through the choroid and nonpigmented pars plana epithelium, as opposed to just the sclera. Care must be taken to choose a location away from a preexisting choroidal or suprachoroidal hemorrhage or known abnormality of the pars plana (trauma scar, persistent hyperplastic primary vitreous (PHPV)). The cannula should be visualized with the operating microscope or an indirect ophthalmoscope, not an unaided eye prior to use to avoid subretinal or suprachoroidal infusion from failure to visualize transparent tissue over the cannula tip. The infusion cannula tubing must be adhesively fastened to the drape with a significant service loop to prevent cannula dislocation from ocular rotation to see the periphery. The cannula must be placed close to the 3 o’clock or 9 o’clock position so that it will not be displaced by the lower lid or speculum during ocular rotation.
Infusion System Technologies
All systems available prior to the development of the Accurus (Alcon, Inc., Ft. Worth, Texas) used gravity-based infusion systems. Gravity-based systems have three significant disadvantages: There is no digital readout of infusion pressure, and surgeons cannot mentally convert inches (or centimeters) of water to millimeters of mercury; IV poles cannot be controlled by the surgeon; and motorized IV poles are slower than the VGFI (vented gas-forced infusion) and the more advanced Alcon Constellation Vision System pressurized infusion/aspiration system. The older gas-forced infusion is better than gravity-based systems because gas-forced infusion readily produces a direct digital readout. The VGFI implemented on the Accurus was even better because it allowed rapid decrease as well as increase of infusion pressure via surgeon foot pedal command. The Constellation Vision System has servo-controlled IOP compensation (not infusion pressure). The Constellation Vision System calibrates the resistance of the infusion system during push-priming, measures actual flow using a proprietary sensing system, calculates fluid Ohm’s law in real time, and adjusts infusion pressure to produce the selected IOP with ±2 mm Hg.
Aspiration Fluidics and Vitreous Cutting
Pars plana vitrectomy requires both infusion and aspiration; there are many parallels between infusion and aspiration fluidics because they are influenced by the same physical principles. Resistance to fluid flow is determined by the internal diameter of a lumen or port, length of tubing, cannula or tool, and the cutter port opening and closing, cyclically obstructing the port. Fluidic resistance is proportional to the fourth power of the diameter (Hagen-Poiseuille equation) and linearly related to the length. The impact of diameter is very significant because of the fourth-power relationship and clinically relevant because of the transition from 20-gauge (0.89 mm) to 23-gauge (0.75 mm) or 25-gauge (0.5 mm) technology in recent years. The resistance of the cutter inner needle and the infusion port limit the flow far more than the 84 inches of connected tubing. Ohm’s law, voltage = current × resistance (E = IR), is mathematically equivalent to Ohm’s law for fluid flow; pressure gradient = flow × resistance.
Port-based flow limiting is a term coined by the principal author that encompasses both the flow limiting resulting from smaller diameter cutters and that caused by higher cutting rates and biased closed duty cycles. Higher cutting rates cyclically interrupt the flow through the port, thereby increasing fluidic resistance (Fig. 2.3). High cutting rates, and therefore higher fluidic resistance at the port, are beneficial for all cases and all tasks because they increase fluidic stability, which, in turn, decreases pulsatile vitreoretinal traction on both detached (retinal motion) and attached retina and therefore iatrogenic retinal breaks. The principal author refers to the amount of fluid that passes through the port during an open-close cycle as “pulse flow.” High cutting rates produce many small-volume pulses with much less remote (far field) effects, that is, pulsatile vitreoretinal traction, than those that occur using lower cutting rates. Small pulse flow means that the vitreous does not have time to produce remote effects because of the Force = Mass × Acceleration (F = MA) relationship. Higher cutting rates do not cut collagen fibers better; this is because the velocity of the cutter does not increase with higher cutting rates on pneumatic cutters. In addition, port-based flow limiting decreases surge, and therefore iatrogenic retinal breaks, after sudden elastic deformation of the dense ERM or scar tissue through the port. High cutting rates in addition to producing desirable port-based flow limiting reduce the travel of uncut vitreous collagen fibers through the port. 25 gauge provides more resistance than 23 gauge because fluidic resistance is proportional to the fourth power of the diameter. Surgeons often incorrectly believe that 25-gauge vitrectomy is “inefficient” or produces insufficient flow rates when, in fact, it is safer because of less pulsatile vitreoretinal traction.
Figure 2.3 High cutting rates cause port-based flow limiting, which increases fluidic stability.
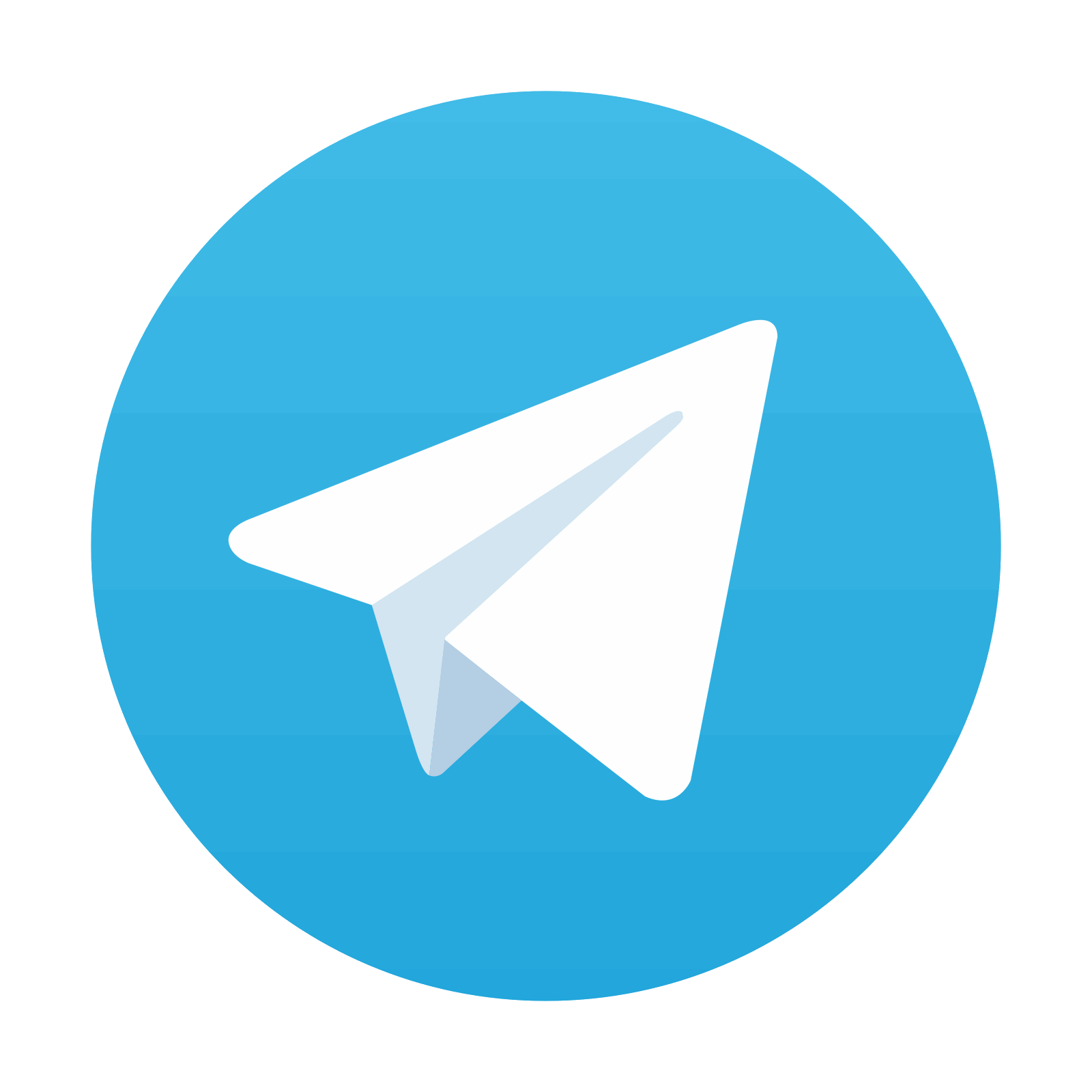
Stay updated, free articles. Join our Telegram channel

Full access? Get Clinical Tree
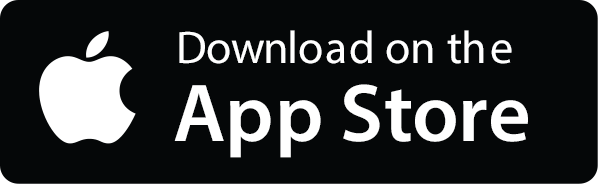
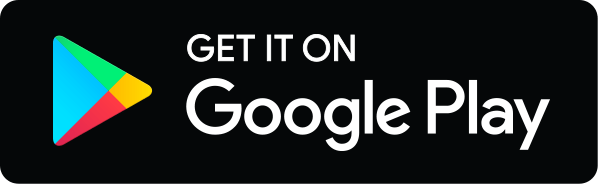