(1)
Department of Ophthalmology, School of Medicine, Gazi University, Besevler, Ankara, 06500, Turkey
(2)
Department of Ophthalmology, Columbia University College of Physicians and Surgeons, Edward S. Harkness Eye Institute, Columbia University Medical Center, 635 West 165th Street, Box 38, New York, 10032, NY, USA
Retinal Arterial Occlusions
Central Retinal Artery Occlusion
Central retinal artery occlusion leads to a sudden blockage of blood supply to inner retina, resulting in severe vision loss. It is an ophthalmic emergency and widely accepted to be the ocular analogue of acute ischemic cerebral stroke. The risk factors are similar to cerebral stroke and ischemic heart disease [1].
The incidence of acute central retinal artery occlusion is 0.85/100,000 per year. It accounts for 1.13 cases in 10,000 ophthalmological outpatient visits and 57 % of all acute retinal artery obstructions [2, 3].
Although central retinal artery occlusion clinically resembles ischemic cerebral stroke, there are several etio-pathogenic differences. Nearly 80 % of cerebral strokes are ischemic in nature and occur due to the stagnation of blood flow within a cerebral artery secondary to a thrombus or embolus. The remaining 20 % are hemorrhagic strokes and as the name implies develops secondary to a cerebral hemorrhage. Analysis of thrombi retrieved from cerebral arteries of patients with acute ischemic stroke revealed that most thrombi were composed of fibrin and platelets. This observation provides the basis for thrombolytic treatment in ischemic stroke [4].
On the other hand, 74 % of the emboli that cause central retinal artery occlusion are made of cholesterol (Hollenhorst plaques) and only 15.5 % of them are fibrin-platelet in nature. The remaining 10.5 % are calcific emboli [5]. Hence, only a small percentage of retinal arterial emboli may respond to thrombolytic treatment.
In spite of its embolic etiology, visible emboli can only be seen in 20–40 % of eyes with central retinal artery occlusion [6, 7]. This may be due to the fact that the embolus usually blocks the central retinal artery where it pierces the dural sheath of the optic nerve. The lumen of the artery is at its narrowest at this site, however, it cannot be seen clinically [8]. Fragmentation and distal migration may also cause emboli to not be clinically visible [6].
Transient occlusion of central retinal artery has also been described. Such transient events have been attributed to the dislodging of emboli, vasospasm of the central retinal artery, or transient compromise of the blood flow due to nocturnal hypotension [9–11].
Clinically central retinal artery occlusion can be subgrouped into four different entities [12]:
- (i)
Non-arteritic permanent central retinal artery occlusion
- (ii)
Non-arteritic transient central retinal artery occlusion
- (iii)
Non-arteritic central retinal artery occlusion with cilioretinal sparing
- (iv)
Arteritic central retinal artery occlusion
There is a “presumed” critical period after the occlusion of the central retinal artery beyond which permanent visual loss occurs. If blockage is removed within this period and blood supply is restored, full visual recovery is possible. Classical knowledge obtained from experiments in old atherosclerotic hypertensive rhesus monkeys indicates that no detectable damage occurs in the retina within the first 97 min after clamping central retinal artery. However, retinal damage becomes massive and irreversible after 240 min [13].
Partial recovery of central vision beyond the described experimental critical period has been reported in patients with non-arteritic permanent central retinal artery occlusion [12, 14, 15]. A simple explanation can be incomplete closure of central retinal artery in such cases [16]. However, discrepancies between the animal model and human disease can also be explained by the failure of central artery clamping to mimic all aspects of central retinal artery occlusion in patients. A recent case series revealed that immediate vitrectomy with or without manual dislodging of the embolus may improve all patients’ central visual acuity by more than three Snellen lines and result in 20/40 or better visual acuity in 44 % of the patients. Rapid alleviation of the cytotoxic retinal edema and increased oxygenation of the retinal penumbra were proposed to explain the visual recovery [17]. This study demonstrates that in patients with central retinal artery occlusion, retinal cell death does not solely occur as a result of acute ischemia but also due to secondary events that follow the ischemic episode, such as cytotoxic cell edema. Inner retinal edema develops in all cases with total occlusion with disorganization of inner retinal layers in 77 % of the patients (Fig. 1). The amount of inner retinal edema is a major factor determining the functional outcome of central retinal artery occlusion and plays a more important role than the time to presentation [18]. This indicates that apart from acute ischemia, there are several secondary events that play a role in inner retinal neuronal loss. In order to comprehend the role of vitrectomy, response of retinal neurons to ischemia has to be reviewed. Once the oxygen saturation starts to drop, inner retinal neurons shut down their excessive metabolic activities to adapt to hypoxic environment. They initiate anaerobic metabolism, increase synthesis of neuroprotective proteins and chaperones, and halt several ATP-consuming processes including the visual cycle. This vegetative state can allow retinal neurons to survive in the hypoxic milieu. Maintenance of viability even in a vegetative state also requires a low but continuous flow of oxygen that is required to maintain basic metabolic needs. Diffusion of oxygen through intact choroid is the most possible source for this limited but vital amount of oxygen. A compensatory increase in choroidal blood flow has been observed in central retinal artery occlusion. The biological effect of oxygen diffusion from the choroid is clinically evident with the intact peripheral visual fields in central retinal artery occlusion where oxygen from the choroid can easily diffuse through the thin peripheral retina to supply adequate oxygen for retinal cell survival. However, the retina is thickest at the perifovea and thus does not allow diffused choroidal oxygen to reach levels as high as in the periphery. Also, the relatively crowded arrangement of retinal neurons aggravates compressional cell death once cytotoxic edema develops. For these reasons, visual field defects manifest most commonly as central scotoma in central retinal artery occlusion. If left untreated, prolonged hypoxia eventually shuts down the energy-dependent ionic pumps and leads to sodium and water leakage into the cell. Hydrops of the retinal neurons finally causes cell rupture and death. Restoration of retinal perfusion before cytotoxic cell edema leads to irreversible neuronal loss can preserve the central vision. Thus the surgical treatment of the central retinal artery occlusion should aim to restore retinal oxygenation either by reestablishing retinal blood flow or by removing the vitreous and elevating preretinal oxygen tension with convectional oxygen currents. Increased oxygenation will initiate full metabolic activity, resolve intracellular edema, and help remaining retinal neurons regain their function. Side benefits of vitrectomy may include removal of inflammatory and pro-apoptotic chemokines, and decreased N-methyl-D-aspartate (NMDA) induced cell death with intravitreal injection of triamcinolone acetonide.


Fig. 1
Forty-seven-year-old patient with central retinal artery occlusion. The patient’s visual acuity is at light perception level. (a) Fundus exam revels typical whitening of the retina and cherry-red spot. (b) A HD-OCT scan reveals hyperreflectivity of the inner retina due to the edema and opacification of the inner retinal layers and cessation of the axoplasmic transport
Several other surgical approaches have been attempted to restore the blood flow in central retinal artery occlusion after the failure of medical treatments [19, 20]. The surgical goal has traditionally been to destroy, remove, or dislodge the visible embolus. Thus, traditional surgical approaches aim to address only 20–40 % of the non-arteritic central retinal artery occlusions where the embolus is visible [6, 7].
These traditional surgical approaches are:
- (i)
Local intra-arterial fibrinolysis
- (ii)
Neodymium:yttrium-aluminum-garnet (Nd:YAG) embolysis and embolectomy
- (iii)
Surgical embolectomy
- (iv)
Surgical cannulation of the central retinal artery
Local intra-arterial fibrinolysis involves local infusion of a fibrinolytic agent, such as urokinase or recombinant tissue plasminogen activator (rt-PA), at the site of occlusion via catheterization of the ophthalmic artery. Although several retrospective reports claimed functional outcomes better than natural history, the recent report of the European Assessment Group for Lysis in the Eye (EAGLE) proved otherwise [21]. In this prospective and randomized multicenter study, patients between the ages of 18 and 75 years who had acute central retinal artery occlusion of less than 20 h onset with a presenting visual acuity of less than 0.32 were randomly assigned to receive either intra-arterial 50 mg recombinant tissue plasminogen activator or a conservative therapy including massage of the eye, topical beta-blocker, acetazolamide, aspirin, heparin, and isovolemic hemodilution. The primary end point was best corrected visual acuity at 1 month. The study was halted after the first interim analysis because similar outcomes were seen in both groups, with an increased risk of complications in the local intra-arterial fibrinolysis group, such as stroke, transient ischemic attacks, aphasia, and hemiparesis [22]. The conclusion of the study was to not recommend local intra-arterial fibrinolysis in treatment of acute central retinal artery occlusion.
Opremcak and Benner were the first to describe the use of Nd:YAG laser to break the intraluminal embolus and dislodge it distally to restore the blood flow in branch retinal artery occlusion [23]. Their technique was subsequently modified by Reynard and Hanscom, to make a small arteriotomy through which the embolus leaves the arterial lumen into the vitreous [24].
Later Opremcak and colleagues reported a case series of ten eyes with central retinal artery occlusion treated with Nd:YAG laser and named the technique “transluminal Nd:YAG embolysis and embolectomy,” depending on whether the embolus was fragmented or moved to the vitreous, respectively [25]. The median energy employed was 1 mJ, with an average energy per pulse at 2.4 mJ (range, 0.3–9 mJ). Restoration of retinal blood flow was noted in all eyes; however, intraoperative complications as vitreous or subhyaloid hemorrhage were noted in five (50 %) patients, which mostly required pars plana vitrectomy. Visual acuity improved with an average of 4.8 Snellen lines gained including the eyes that underwent vitrectomy. However, this technique was not extensively embraced due to failure to reproduce similar results, limited effectiveness, and high complication rates [26].
After the report of successful surgical removal of a branch retinal artery embolus [27], there have been attempts of surgical embolectomy for central retinal artery occlusion [26, 28]. With this technique, following standard three-port pars plana vitrectomy with removal of posterior hyaloid, the artery is reached and dissected with a microvitreoretinal blade or bent needle tip, and the plaque is dislodged into the vitreous cavity spontaneously or removed with a vitreoretinal forceps. In case of arterial bleeding, hemostasis is obtained by increasing the intraocular pressure. Although bleeding was a common complication, authors noted that occasionally vasospasm closed the incision. This procedure was also performed with transconjunctival sutureless small-gauge pars plana vitrectomy [28]. However, none of the operated eyes improved beyond counting fingers [26, 28]. Currently, a prospective multicenter study has been recruiting patients to determine whether surgical embolectomy results in better functional outcome compared with natural history [29].
Tang and Topping reported a single case treated with surgical cannulation of the central retinal artery [30]. After vitrectomy, they penetrated the central bifurcation of the central retinal artery with a microvitreoretinal blade and cannulated the central retinal artery for a length of 3.5 mm with a 50-gauge nickel titanium flexible stylet. They moved the stylet forward and backward with circular motions and observed emergence of a small clot. An improvement in visual acuity from counting fingers to 20/25 at 4 months was noted.
In a recent study, the effectiveness and safety of vitrectomy with and without manual dislodging of the embolus was reported [17]. In this case series of 18 eyes with non-arteritic central retinal artery occlusion, immediate 25-gauge pars plana vitrectomy with posterior hyaloid separation and manual dislodging of the embolus was performed within 36 ± 25 h (range: 6–72 h) of the onset of the symptoms. Intraoperative restoration of the retinal blood flow along with rapid disappearance of the macular edema was observed in all patients. Visual acuity improved more than three lines in all eyes. At 2 weeks, eight patients (44.4 %) were able to see 20/40 or better, whereas visual acuity remained below 20/200 in another eight patients (44.4 %). No significant visual acuity changes were observed after the second postoperative week for up to 12 months. Results of this study indicate that immediate vitrectomy can result in partial restoration of foveal vision in central retinal artery occlusion.
Branch Retinal Artery Occlusion
Branch retinal artery occlusion results in less severe loss of vision compared to central retinal artery occlusion. An embolic event with underlying atherosclerosis is almost always the cause apart from arteritic cilioretinal artery occlusion. Central and branch retinal artery occlusions share the same risk factors. Branch retinal artery occlusion represents 38 % of all acute retinal artery obstructions [2].
Actually, the so-called branch retinal arteries are arterioles; hence, the widely used term of “branch retinal artery occlusion” is a misnomer. Their diameter close to the optic disc is about 100 μm, which is typical for an arteriole. In addition, unlike arteries, they possess neither an internal elastic lamina nor a continuous muscular coat. Therefore, they are not affected by giant cell arteritis [31].
Branch retinal artery occlusion has been divided into three subclasses:
- (i)
Permanent branch retinal artery occlusion
- (ii)
Transient branch retinal artery occlusion
- (iii)
Cilioretinal artery occlusion
- (a)
Non-arteritic cilioretinal artery occlusion alone
- (b)
Non-arteritic cilioretinal artery occlusion with retinal vein occlusion
- (c)
Arteritic cilioretinal artery occlusion
- (a)
Visible emboli in branch retinal arteries can be observed much more often in branch retinal artery occlusion than in patients with central retinal artery occlusion [32, 33].
The visual prognosis in branch retinal artery occlusion is generally favorable, with final visual acuity of 20/40 or better in 80–90 % of eyes [34, 35]. Some degree of macular perfusion through contralateral temporal branch can prolong the critical period for retinal survival and increase the chance for spontaneous visual recovery in branch retinal artery occlusion [26, 36]. Aggressive therapy is required for branch retinal artery occlusion cases involving the fovea [31].
Two surgical treatment modalities for branch retinal artery occlusion have been described:
- (i)
Surgical embolectomy
- (ii)
Nd:YAG embolysis and embolectomy
Surgical removal and intravascular fragmentation of an embolus in a branch retinal arteriole was first described by Peyman and Gremillion [27]. Despite the surgical procedure being done 60 h after the onset of the occlusion, visual acuity of their patient improved from counting fingers to 20/200. The gain in visual acuity was also observed in a following case series where six patients underwent surgical embolectomy within 20.5 h (range 4–33 h) of the occlusion [26]. However, these results may not be any better than the natural course of branch retinal artery occlusion [36].
Opremcak and Benner described Nd:YAG laser embolysis in nine patients [23, 25]. Three patients (33 %) experienced dense vitreous hemorrhage that required vitrectomy to clear the blood. Overall, VA improved an average of 4.67 Snellen lines [25]. The functional outcome of this study is also no different than the natural course of the branch retinal artery occlusion. Moreover, serious complications such as subretinal hemorrhage, retinal tears, choroidal neovascularization, and epiretinal proliferation can occur during or after Nd:YAG laser embolysis [37].
Retinal Venous Occlusions
Central and branch retinal vein occlusions are common causes of vision loss in the elderly and the second most common retinal vascular disease after diabetic retinopathy. Their prevalence is 1–2 % in people older than 40 years of age, with branch retinal vein occlusion being four times as common as central retinal vein occlusion [38–40].
Retinal vein occlusions are classified into two major subclasses:
- (i)
Central retinal vein occlusion
- (a)
Ischemic
- (b)
Nonischemic
- (a)
- (ii)
Branch retinal vein occlusion
- (a)
Ischemic
- (b)
Nonischemic
- (a)
Hemicentral retinal vein occlusion is a rare and a unique condition where one of the two trunks of the central retinal vein is occluded [41]. Its clinical features resemble those of a central retinal vein occlusion. Macular branch vein occlusion is another subset of branch retinal vein occlusion, where a small branch that drains a part of the macula is affected. Macular branch vein occlusion shows different characteristics than branch retinal vein occlusion. It is not associated with subsequent retinal neovascularization; complications such as macular edema and hemorrhages are less common, resolve earlier [42], and respond to vitrectomy better [43].
All retinal vein occlusion have thrombotic etiologies. The pathogenesis of retinal vein occlusion has been described to follow the principles of thrombogenesis, involving hemodynamic changes (stasis, turbulence) and endothelial injury [44]. The vein is believed to become narrowed at an arteriovenous crossing point due to compression from the neighboring atherosclerotic artery, where both vessels share the same adventitial sheath. The blood flow in the vein becomes turbulent at the constriction, which inevitably causes endothelial cell damage and death, exposing subendothelial matrix and leading to formation of a clot [45]. Although both branch and central retinal vein occlusions are thrombotic processes, the impact of local and systemic factors in predisposing to these conditions is quite different. For example, increased blood pressure, hyperopia, and atherosclerosis are more common in branch retinal vein occlusion patients, whereas high intraocular pressure seems to play a more important role in the development of central retinal vein occlusion [46]. Such differences also exist in the natural history and therapeutic response of both pathologies [47].
A variety of hematologic risk factors for general venous thrombosis have been observed to occur sporadically in retinal vein occlusions. It was concluded that they do not play a major importance in the pathophysiology of retinal vein occlusion [48, 49].
Retinal vein occlusion results in delay in retinal blood flow through the occluded segment, which in turn leads to macular ischemia and/or edema, two major causes of loss of visual acuity. Increased hydrostatic pressure, ischemia-induced vascular endothelial growth factor upregulation, and inflammation are the major causes of cystoid macular edema in retinal vein occlusion [50, 51]. Moderate to severe macular edema is a part of the clinical picture in 87 % of the central retina vein occlusions (Fig. 2) [52], 51 % of the branch retinal vein occlusions (Fig. 3), and 29 % of the macular branch vein occlusions. It resolves in 51 % of the cases in 2 years [42, 52]. Short duration of macular edema is known to be associated with favorable visual outcome in retinal vein occlusions [53]. Results from various studies have shown that macular edema can be controlled with intraocular injections of anti-vascular endothelial growth factor agents and/or steroid implants [54–56]. Despite good initial response, several intravitreal injections are needed to prevent the recurrence of the macular edema. Pharmacologic therapy also falls short of addressing other comorbidities, such as macular ischemia. Although functional results of several intravitreal anti-vascular endothelial growth factor trials for vein occlusions are favorable, application of these results to daily clinical life may be misleading since in many trials, eyes with characteristics of ischemia such as poor visual acuity and afferent pupil defect were excluded [57, 58].



Fig. 2
Seventy-three-year-old male patient with central retinal vein occlusion. (a) Posterior segment exam reveals tortuous veins and petaloid appearance of cystoid macular edema. (b) Horizontal OCT scan crossing the foveola shows “volcano-style” cystoid macular edema. Note that some of the Muller cell columns have already broken up, allowing lateral spread of the intraretinal fluid indicating the chronicity of the edema

Fig. 3
Seventy-one-year-old male with left inferotemporal branch retinal vein occlusion. (a) Occlusion of the inferotemporal retinal vein at the first bifurcation resulted in intraretinal hemorrhages and edema extending toward fovea. (b) A cross-sectional HD-OCT reveals acute massive intraretinal fluid buildup at the fovea. Note that posterior hyaloid remained attached at the apex of the cystoid macular edema
Central Retinal Vein Occlusion
The prevalence of CRVO is estimated to be 0.80 per 1000 persons (95 % CI: 0.61–0.99) [40]. The surgical approaches described for its treatment can be classified as follows:
- (i)
Induction of chorioretinal venous anastomosis with intense laser burns
- (ii)
Surgical establishment of chorioretinal venous anastomosis
- (iii)
Pars plana vitrectomy
- (iv)
Radial optic neurotomy (RON) (also called optic nerve sheath decompression)
- (v)
Retinal vein cannulation and direct injection of recombinant tissue plasminogen activator (also called retinal endovascular lysis)
McAllister and Constable described the technique of laser-induced chorioretinal venous anastomosis for the treatment of nonischemic central retinal vein occlusion, in which a focal laser burn near a tributary vein is performed to rupture the vein wall toward the underlying Bruch’s membrane. The aim of this procedure was to produce a functional anastomosis between the occluded vein and choroidal circulation [59, 60]. A chorioretinal anastomosis was achieved in about a third of eyes with nonischemic central retinal vein occlusion. However, no functional anastomosis was achieved in eyes with ischemic central retinal vein occlusion [61]. Difficulty of the technique and low rate of success, coupled with high rate of adverse events such as chorioretinal neovascularization at the treatment site, fibrous tissue proliferation, distal vein closure, macular traction, and vitreous hemorrhage, impeded approval of the technique [62–64]. Also some criticism was raised claiming that the technique conferred no additional benefits when compared to the natural course of nonischemic central retinal vein occlusion [63].
Later, Peyman and colleagues described the surgical method to induce chorioretinal anastomosis [65]. Following a standard three-port pars plana vitrectomy and separation of the posterior hyaloid, they placed multiple slit-like incisions through the Bruch’s membrane around major veins in four quadrants. In order to promote chorioretinal vascularization, they inserted Mersilene sutures over these incisions. In their series of five eyes with ischemic central retinal vein occlusion, 10 of 16 anastomoses remained patent. Visual acuity improved in three eyes (60 %) and one eye achieved 20/50 vision. Significant complications included optic atrophy (60 %), occlusion of the retinal veins (40 %), vitreous hemorrhage (80 %), macular traction due to significant epiretinal membrane formation (40 %), and fibrous glial proliferation at chorioretinal incision sites. Absence of iris or angle neovascularization was considered to be a beneficial effect of the surgery.
Kado and colleagues observed increased incidence of posterior vitreous detachment (PVD) in eyes followed with central retinal vein occlusion. They attributed this to the plasma leak into the vitreous from the occluded vessels. High incidence of persistent macular edema in cases with attached posterior hyaloid suggested the role of vitreomacular adhesion in the development of macular edema and pars plana vitrectomy as a surgical remedy to relieve the traction [66]. Potential benefits of vitrectomy also included removal of the cytokines that increase vascular permeability such as vascular endothelial growth factor and an increase in retinal oxygenation [67, 68]. Efficacy of pars plana vitrectomy in central retinal vein occlusion has been reported in many case series. Better visual results have been obtained in eyes with nonischemic central retinal vein occlusion treated earlier [69–75]. Surgical trauma and ischemia-related inner retinal atrophy remained as the two major factors for the failure of visual gain after resolution of the macular edema in these vitrectomized eyes [76].
A false assumption has been considering internal limiting membrane as a barrier for diffusion of intraretinal fluid into vitreous [51]. This idea led to a few attempts to decompress the swollen retina with internal limiting membrane peeling during pars plana vitrectomy [77–79]. As expected, no benefit was seen in peeling the internal limiting membrane, and even a detrimental effect on visual acuity was noted [76, 80–82].
Another surgical approach used to treat central retinal vein occlusion was radial optic neurotomy. This approach was based on the assumption that optic nerve is compressed at the level of lamina cribrosa due to a tight and rigid scleral ring. A radial cut to nasal parapapillary scleral ring was suggested as a remedy to relieve the compression on optic nerve [83]. Despite improvement in visual acuity in some eyes [84–87], this technique was criticized for lacking anatomic basis. Contradicting studies reported the site of occlusion of the vein to be at varying distances posterior to the lamina cribrosa in the optic nerve, rather than at lamina cribrosa as suggested. In histopathological studies lamina cribrosa was found to be a firm, compact, rigid band of collagen tissue instead of an elastic structure that could be decompressed with a radial incision. Also, a common fibrous tissue capsule wrapping the central retinal artery and vein throughout their course at the center of the optic nerve was found to be a more likely structure to compress the central retinal vein rather than the scleral ring outside optic nerve [47, 88–90]. Radial optic neurotomy surgery also carries some serious complications, such as severe immediate intravitreal hemorrhage, choroidovitreal neovascularization, visual field defects, retinal detachment originating from the incision site, neovascular glaucoma, phthisis bulbi, central retinal artery laceration, and optic nerve atrophy [85, 86]. Observing comparable benefits without a radial parapapillary cut indicates that pars plana vitrectomy and subsequent physiological changes, such as increased oxygenation, removal of inflammatory and angiogenic mediators, or relief of traction provide the reported benefits [84].
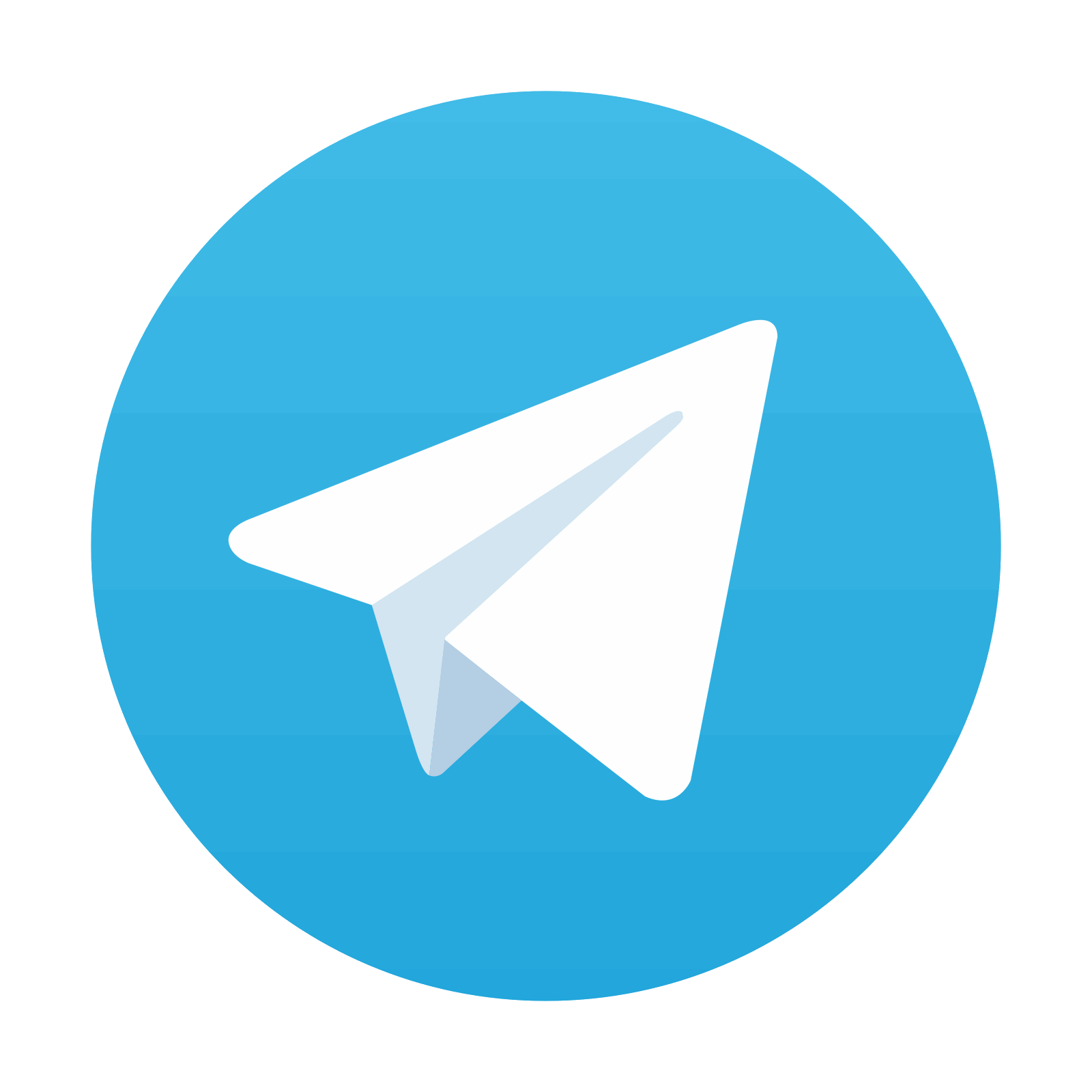
Stay updated, free articles. Join our Telegram channel

Full access? Get Clinical Tree
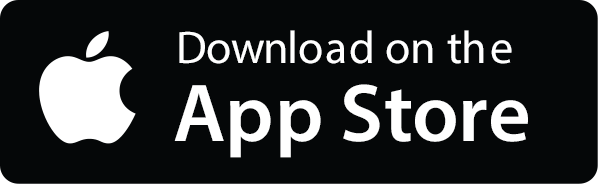
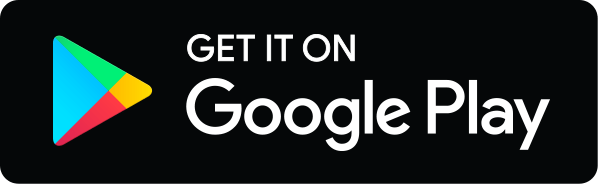