IA Trabecular Outflow The trabecular outflow pathway is the eye’s main site of aqueous humor (AH) drainage. It is responsible for 60 to 95% of AH outflow depending on the age of the patient and the health of the eye. The remaining drainage occurs through the uveoscleral pathway. The trabecular outflow pathway is located at the iridocorneal angle, and it is composed of the trabecular meshwork (TM), endothelial lining of Schlemm’s canal, collector channels, and aqueous veins (Fig. 2.1). The trabecular outflow pathway is a key site of intraocular pressure (IOP) regulation and a critical pathway in the pathogenesis of primary open-angle glaucoma (POAG). As AH exits the anterior chamber through the trabecular outflow pathway, it first traverses the three layers of the TM. The TM is located in a semicircular groove on the inner aspect of the sclera known as the scleral sulcus. The TM attaches anteriorly to the peripheral cornea at Schwalbe’s line and inserts posteriorly into the scleral spur.1 Attachment of the TM together with the ciliary muscle to the scleral spur is important in regulating the trabecular outflow pathway, as this arrangement allows ciliary muscle contraction to widen the TM. Structurally, the TM is a sponge-like network of connective tissue composed of trabecular beams, which contain a core of collagen and elastin fibers. Lining the trabecular beams are TM endothelial cells. The cells on the outer layers of the TM are phagocytic in nature and are believed to help remove cellular debris from the AH.1 The TM serves two main functions in the eye. First, it filters the AH, removing any cellular or pigment debris that can disrupt drainage of AH through the trabecular outflow pathway. Second, it acts as a path of resistance for the regulation of outflow facility and ultimately IOP. Histologically, the TM can be separated into three regions that differ in structure: the uveal meshwork, the corneoscleral meshwork, and the juxtacanalicular tissue (JCT). The uveal meshwork is the innermost region of the trabecular meshwork. It originates at the anterior aspect of the ciliary body and inserts at the peripheral cornea. The uveal meshwork is composed of one to three layers of trabecular beams and has relatively large intertrabecular spaces, which provide minimal resistance to AH outflow.2 The corneoscleral meshwork forms the middle region of the TM and extends from the scleral spur to Schwalbe’s line. This region is composed of eight to 15 layers of trabecular beams, which are thicker than the uveal meshwork, resulting in smaller intertrabecular spaces that confer a greater resistance to AH outflow.2 The JCT is the thinnest (2–20 µm) and outermost portion of the TM and is located directly adjacent to the endothelial lining of Schlemm’s canal. It has a unique architecture compared with that of the other regions of the TM, in that it is not composed of trabecular beams. The JCT is a dynamic and complex region made up of loose connective tissue supported by an incomplete basement membrane with TM cells surrounded by a fibrillar extracellular matrix (ECM).1,3 Compared with the other regions of the TM, it has relatively low porosity, and it functions as the main point of resistance for the TM outflow pathway. Within the ECM of the JCT, there are nonstructural elements called matricellular proteins, such as secreted protein acidic and rich in cysteine (SPARC) and thrombospondin-1 (TSP-1). Matricellular proteins are secreted by cells of the ECM and aid in cellular communication with the ECM.4–6 A prominent structural change in the TM of patients with POAG is an increase in the fibrillar content in the ECM of the JCT.7 Due to the increased deposition of ECM in patients with POAG and the importance that ECM turnover plays in managing outflow facility, matricellular proteins have become an important focus for determining the pathogenesis of POAG. After passing through the TM, AH drains into Schlemm’s canal. Schlemm’s canal is a collecting channel deep to the TM in the scleral sulcus. The inner wall of Schlemm’s canal is formed by a layer of endothelial cells. As AH flows by transcellular transport into the inner wall endothelium, giant vacuoles develop and bulge into the lumen of the canal8,9 (Fig. 2.2). The giant vacuoles in Schlemm’s canal are hypothesized to be involved in regulating the resistance of AH outflow. The outer wall of Schlemm’s canal has a single layer of endothelium and contains outlet channels that drain directly into the episcleral veins. The flow of AH through the TM outflow pathway is a pressure-dependent process. At steady-state IOP, the combination of flow of AH across the trabecular and uveoscleral outflow pathway is at the same rate as its production from the ciliary body.9 In pathological processes, such as aging and POAG, the steady-state IOP can shift. The increase in IOP in these two examples is due to an increase in resistance across the TM outflow pathway.10,11 Three major mechanisms govern outflow resistance across the TM outflow pathway. The transcellular route is the flow of AH as it leaves the JCT and enters vacuoles in the inner-wall endothelial cells of Schlemm’s canal. The paracellular route is the flow of AH passing between the inner-wall endothelial cells of Schlemm’s canal. The third mechanism of resistance is the ECM turnover that occurs in the JCT region12 (Fig. 2.3). Because of the electrical charge of many of the structural components, the JCT ECM provides resistance to water and ions. Flow of AH through the TM is a dynamic process that requires regulation of outflow resistance to maintain a stable IOP. Homeostatic regulation of outflow resistance is believed to be triggered by cellular monitoring of changes in stretch or distortion of the TM. Cellular signals then alter ECM turnover in the TM to shift its balance toward higher or lower resistance.9,13 Matricellular proteins, such as SPARC, may be key intermediates in the regulation of ECM turnover as they are highly expressed following cellular stress and are involved in ECM regulation in other tissues. Alteration in the regulation of ECM turnover is believed to be key in the pathogenesis of POAG. Patients with POAG characteristically display increased deposition of ECM in the JCT region of the TM.7 Multiple studies have shown that patients with POAG have elevated levels of transforming growth factor-β2 (TGF-β2), which increases ECM synthesis in the TM.14,15 The trabecular outflow pathway receives contractile forces from the ciliary body and scleral spur. Tendons of the ciliary muscle extend anteriorly and attach to the scleral spur; they are continuous with the ECM of the TM. As a result, contraction of the ciliary muscle posteriorly displaces the scleral spur and widens the trabecular meshwork, which enlarges the intertrabecular pores and decreases outflow resistance (Fig. 2.4). The cells of the scleral spur also have a contractile phenotype and receive parasympathetic innervation.16,17 Given the attachment of the TM to the scleral spur, a change in scleral spur cell tone would likely affect outflow resistance. Furthermore, there is evidence to suggest a contractile phenotype for TM cells as well, and studies have shown that TM cells can modify outflow resistance by changing cellular tone and altering the contractile nature of the JCT–Schlemm’s canal region.18,19 Fig. 2.3 Three mechanisms of resistance to AH outflow in the trabecular meshwork outflow pathway: 1, extracellular matrix turnover occurs in the juxtacanalicular tissue region. 2, the transcellular route is the flow of AH as it leaves the JCT and enters vacuoles in the inner wall endothelial cells of Schlemm’s canal. The vacuoles provide resistance to outflow. 3, the paracellular route is the flow of AH passing between the inner wall endothelial cells of Schlemm’s canal.
2 Structure and Mechanisms of Trabecular Outflow
Trabecular Meshwork
Uveal Meshwork
Corneoscleral Meshwork
Juxtacanalicular Tissue
Schlemm’s Canal
Physiology of Trabecular Meshwork Outflow
Extracellular Matrix Turnover
Contractile Influence on Trabecular Outflow
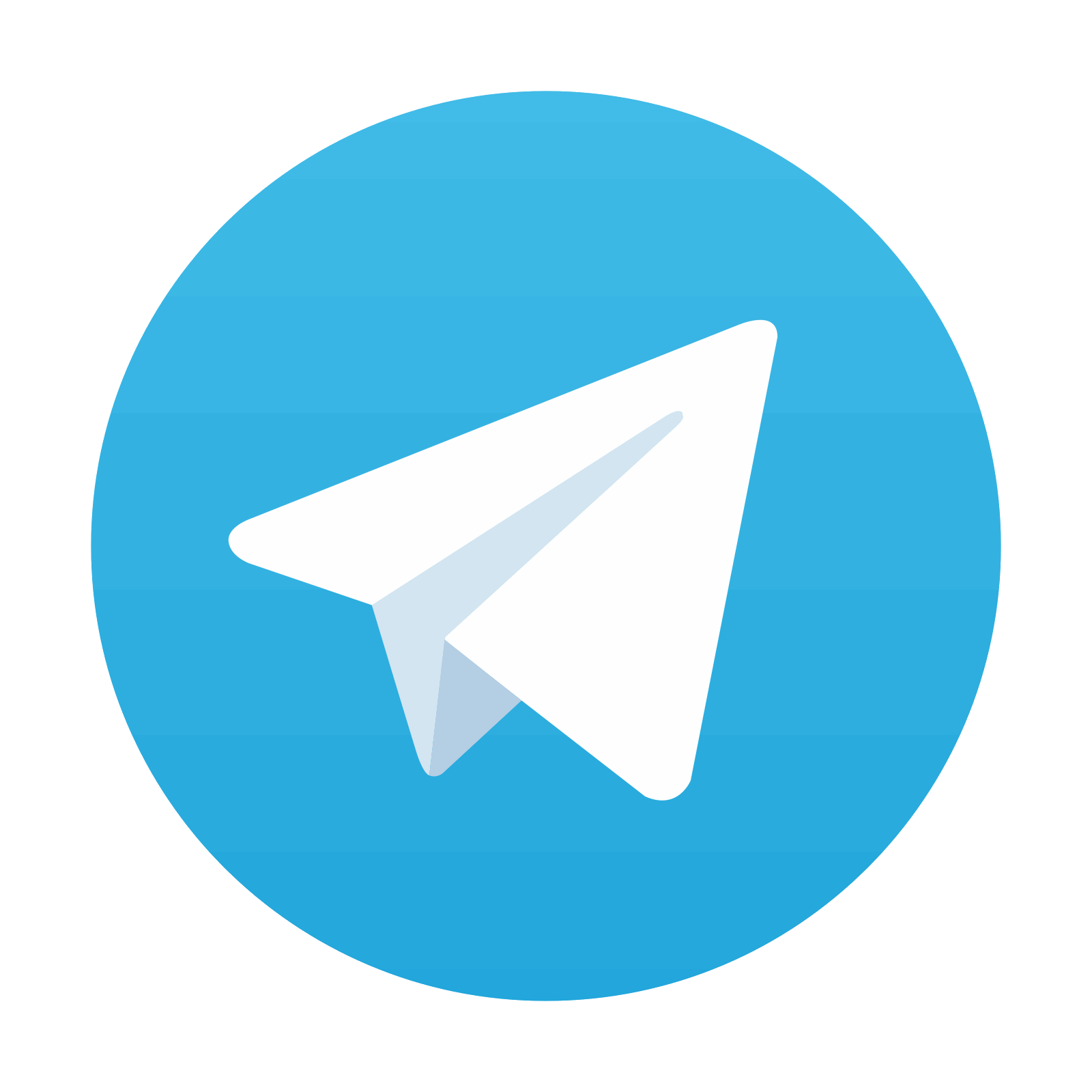
Stay updated, free articles. Join our Telegram channel

Full access? Get Clinical Tree
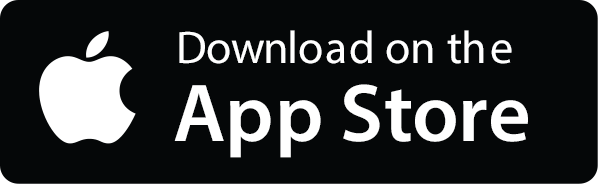
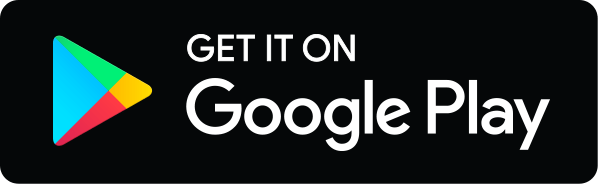