Vestibular schwannomas are benign tumors of the Schwann cells of the eighth (VIII) cranial nerve. Precision radiotherapy techniques used to manage these tumors include stereotactic radiotherapy (SRT), which can be delivered with either a conventional or hypofractionated regimen. The radio–biologic rationale and reported clinical outcomes of patients treated with SRT are reviewed.
Vestibular schwannomas (acoustic neuromas) are typically benign tumors that arise from the Schwann’s cells of the eighth (VIII) cranial nerves. Treatment options include observation, surgical resection, and radiation therapy, which can be delivered in a conformal or stereotactic manner. The choice of treatment is multifactorial and includes patient and physician preference, age, tumor size, and symptoms.
Stereotactic techniques for treating vestibular schwannoma include single fraction stereotactic radiosurgery (SRS) and fractionated stereotactic radiotherapy (SRT). Evaluation of management trends from 10 years ago predicted an explosive growth of SRS for vestibular schwannomas in the next century, and this growth has been realized. A similar growth potential also can be surmised for SRT, but there is no consensus as yet on the optimal technique, dose, or fraction size. This article describes the basic concepts of stereotactic radiotherapy and the radiobiologic rationale for this treatment and reports the clinical results.
Stereotactic radiosurgery versus radiotherapy
Stereotactic Radiosurgery
The definition of SRS is defined variably but generally is considered to be a single high dose of radiation delivered with rigid immobilization to an intracranial target to achieve a biologic result. As a rule, this treatment implies the use of a halo or frame that is affixed rigidly to the patient ‘s skull to allow for precise immobilization and to provide the reference for defining the target in space relative to a Cartesian coordinate system. The treatment can be delivered with a dedicated radiosurgery machine such as a Leksell Gamma Knife (Elekta, Norcross, Georgia) or a modified linear accelerator, of which there are various commercially available products. The invasive nature of this fixation, usually by pins that are screwed into the skull, makes this technique feasible only for single-fraction treatment. Further, the fact that a high dose is delivered in one fraction limits this treatment to small targets, usually less than 3 cm. SRS has been very effective in treating various intracranial targets, including vestibular schwannomas, meningiomas, vascular lesions, trigeminal neuralgia, and primary and metastatic brain tumors.
Stereotactic Radiotherapy
SRT is a technique that attempts to combine the biologic advantages of fractionation (ie, delivering multiple small doses of radiation) with the physical advantages of the precision immobilization seen in SRS. This technique is performed on a linear accelerator with a relocatable stereotactic frame such as with the Novalis (BrainLab Incorporated, Westchester, Illinois) or Radionics (Burlington, Massachusetts) systems or with frameless systems that use optical tracking such as Cyberknife (Accuray Incorporated, Sunnyvale, California). Much of published experience with SRT has been with the Gill-Thomas-Cosman relocatable head ring, an example of which is shown in Fig. 1 . Treatments can be delivered with multiple noncoplanar arcs or with fixed fields. Multiple noncoplanar arcs imply that a finely collimated linear accelerator delivers the radiation dose while rotating around the patient, whereas with fixed fields, the beam is fixed but delivered through multiple points that converge on the target lesion. As a rule, the precision for SRT is less than that of SRS, with a relocatable accuracy of approximately 2 mm, and often safety margins of 2 to 5 mm are added to the planning volume to account for this uncertainty.
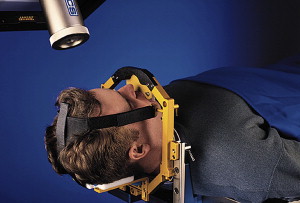
In recent years, the development of intensity-modulated radiation therapy (IMRT) and image-guided radiation therapy (IGRT) has refined SRT further. IMRT is the ability to modulate the intensity of the radiation beam through the treatment volume to allow for maximum sparing of normal tissues with improved dosimetry to the target. IGRT is the use of daily imaging of the target volume before treatment delivery to account for patient movement to assure target accuracy. One could argue that these techniques have replaced SRT in that they are imaging- and not coordinate (or frame)-based. However defined, these techniques are used widely in many radiation oncology departments and have allowed for treatments with SRT-like precision to be performed on many of the same intracranial targets as SRS. One posited advantage of SRT relative to SRS is that because of the ability to fractionate the treatment, targets larger than 3 cm/or those involving or adjacent to critical structures such as the brainstem or optic apparatus can be treated with SRT without undue risk of complications. These size and dose constraints are much more limiting in single-fraction SRS. Further, these developments are being applied to extracranial targets, including tumors in the lungs, pancreas, liver, prostate, and spine.
Radiobiologic rationale for stereotactic radiotherapy
SRS involves delivery of a single dose of radiation while SRT involves administering a more protracted and fractionated course of treatment. For both SRS and SRT, the megavoltage radiation delivered during treatment causes free radical-mediated damage to the DNA of the target. When treating fast-growing tumors such as squamous cell carcinomas of the head and neck or uterine cervix, both fraction size and overall treatment time help to determine the tumor control probability. Such fast-growing tumors can experience accelerated repopulation, whereby surviving cancer cells, during a protracted course of radiotherapy, begin to divide and multiply more rapidly. The potential for accelerated repopulation necessitates that the treatment of such fast-growing tumors be completed in a timely manner. If there are unplanned breaks during a course of radiotherapy, this may lead to a decrease in tumor control. For slow-growing tumors such as vestibular schwannomas, on the other hand, the overall treatment time has little influence on tumor control probability. The daily dose of the radiation therapy that is delivered is considered the principle factor in determining the late effects of radiation therapy. Therefore, a radiobiological concept favoring SRT over SRS for treating vestibular schwannoma would be that fractionation would not be expected to adversely impact tumor control probability, while it may reduce long-term damage to surrounding normal tissues by allowing nearby critical structures to repair sublethal radiation-induced damage.
Little is known about the precise radiobiological mechanisms that mediate the effects of ionizing radiation on vestibular schwannoma. An interesting study was done at the University of Pittsburgh in which athymic mice were transplanted with human xenografts of vestibular schwannoma tissue. This study sought to describe the mechanism of SRS-induced tumor control. Various SRS regimens ranging from 10 Gy to 40 Gy were delivered in a single fraction, and mice subsequently were sacrificed and the tissue studied at 2 weeks, 1 month, and 2 months after SRS. An average tumor volume reduction of about 16.4% was noted at 3 months in the 10 Gy arm. Histologic examination showed a higher incidence of hemosiderin deposits and vascular mural hyalinization in SRS xenografts versus controls, suggesting both a cellular and vascular effect from radiosurgery.
As will be shown in the next section, both SRS and SRT lead to high tumor control probability rates, with good preservation of hearing and limited facial nerve dysfunction. Understanding of the radiobiological mechanisms governing tumor control and normal tissue damage from radiation, however, is in its nascency. Although it is not known what the optimal dose and optimal fractionation regimen are for treating vestibular schwannoma, tumor control probability with SRS and SRT is excellent, and the risk of long-term morbidity from these treatments remains low.
Radiobiologic rationale for stereotactic radiotherapy
SRS involves delivery of a single dose of radiation while SRT involves administering a more protracted and fractionated course of treatment. For both SRS and SRT, the megavoltage radiation delivered during treatment causes free radical-mediated damage to the DNA of the target. When treating fast-growing tumors such as squamous cell carcinomas of the head and neck or uterine cervix, both fraction size and overall treatment time help to determine the tumor control probability. Such fast-growing tumors can experience accelerated repopulation, whereby surviving cancer cells, during a protracted course of radiotherapy, begin to divide and multiply more rapidly. The potential for accelerated repopulation necessitates that the treatment of such fast-growing tumors be completed in a timely manner. If there are unplanned breaks during a course of radiotherapy, this may lead to a decrease in tumor control. For slow-growing tumors such as vestibular schwannomas, on the other hand, the overall treatment time has little influence on tumor control probability. The daily dose of the radiation therapy that is delivered is considered the principle factor in determining the late effects of radiation therapy. Therefore, a radiobiological concept favoring SRT over SRS for treating vestibular schwannoma would be that fractionation would not be expected to adversely impact tumor control probability, while it may reduce long-term damage to surrounding normal tissues by allowing nearby critical structures to repair sublethal radiation-induced damage.
Little is known about the precise radiobiological mechanisms that mediate the effects of ionizing radiation on vestibular schwannoma. An interesting study was done at the University of Pittsburgh in which athymic mice were transplanted with human xenografts of vestibular schwannoma tissue. This study sought to describe the mechanism of SRS-induced tumor control. Various SRS regimens ranging from 10 Gy to 40 Gy were delivered in a single fraction, and mice subsequently were sacrificed and the tissue studied at 2 weeks, 1 month, and 2 months after SRS. An average tumor volume reduction of about 16.4% was noted at 3 months in the 10 Gy arm. Histologic examination showed a higher incidence of hemosiderin deposits and vascular mural hyalinization in SRS xenografts versus controls, suggesting both a cellular and vascular effect from radiosurgery.
As will be shown in the next section, both SRS and SRT lead to high tumor control probability rates, with good preservation of hearing and limited facial nerve dysfunction. Understanding of the radiobiological mechanisms governing tumor control and normal tissue damage from radiation, however, is in its nascency. Although it is not known what the optimal dose and optimal fractionation regimen are for treating vestibular schwannoma, tumor control probability with SRS and SRT is excellent, and the risk of long-term morbidity from these treatments remains low.
Clinical results
In reviewing the data on SRT for vestibular schwannoma, one must compare outcome measures with the mature results obtained for SRS. These measures include tumor control, hearing preservation, and the development of cranial neuropathies. Chopra and colleagues recently reported the results from the University of Pittsburgh of patients treated with Gamma Knife radiosurgery with marginal tumor doses of 12 Gy to 13 Gy. The authors reported a 10-year actuarial resection-free control of 98%, with a cranial nerve complication rate of less than 5%. Although hearing preservation is a more difficult parameter to quantify, they reported that among those patients who had testable hearing, the hearing preservation rate was 78% to keep the same Gardner-Robertson level and 97% to preserve any testable level of hearing. Similarly, Friedman and colleagues, in their series of 390 patients treated with single-fraction linear accelerator radiosurgery with a marginal dose of 12.5 Gy, described a 5-year actuarial control rate of 90%, with a cranial nerve complication rate of less than 5%. Thus, when analyzing the results of SRT, it is important that the outcomes approach those of these and other mature SRS series.
In addition, it is helpful to distinguish between SRT series that use conventional fractionation and those that use hypofractionation. Conventional fractionation SRT is based on longstanding experience in the treatment of cranial (and extracranial) tumors with nonstereotactic techniques and generally implies 5 to 6 weeks of daily treatment with total doses of between 45 Gy and 58 Gy. Conversely, hypofractioned SRT is by definition the use of larger but fewer doses of radiation delivered over a shorter period. Hypofractionation is used to gain some of the advantages of fractionation in terms of sparing normal tissues, with the convenience of a more limited treatment time. Hypofractionation schedules typically range from three to six fractions. Unfortunately, the optimal dose/fractionation schedule for this approach is unknown in terms of tumor control and complication potential. Hypofractionation is more akin to single-fraction SRS than conventional SRT and, therefore, would require more precision than conventional fractionation SRT. Conventional fractionated SRT, on the other hand, is more analogous to conventional radiation therapy techniques.
A summary of the reported series for conventionally fractionated SRT is shown in Table 1 . In the studies referenced in Table 1 treatment consisted of conventional fractionation schedules between 45 Gy and 57.6 Gy delivered over 5 to 7 weeks. These data are remarkable for being fairly consistent with local control rates of greater than 86%. Local control generally is defined as tumor stability or regression. Included in this group is the series with the longest follow-up of 80 months reported by Maire and colleagues. Maire and colleagues described an actuarial 15-year local control rate of 86%, which is quite good given the large median tumor size of 3.1 cm, but also underscores the possibility of late recurrences and the need for life-long follow-up, especially in younger patients. It is interesting that this series of patients was treated without stereotactic immobilization but only with mask fixation. In other words, the target was defined using mask fiducials on a day-to day basis as opposed to stereotactically defining the isocenter as with SRT. Thus, although stereotactic treatment has become the predominant treatment approach for vestibular schwannomas, conventional external beam treatment remains an effective modality. The remainder of the series with stereotactic immobilization show local control rates of greater than 90% but at shorter follow-up intervals.
