Meningiomas are the most common nonglial brain tumors. They tend to be slow growing and benign and can reach substantial sizes before becoming symptomatic. Complete surgical resection of intracranial meningiomas remains the treatment of choice. Location of a meningioma within the cranial vault may make complete surgical resection unlikely; tumors arising from the dura of the skull base are particularly challenging. Advances in radiation therapy, including stereotactic techniques, can expand the options for treatment available in these situations. They may be used as adjuncts to surgery or as alternative modalities in the treatment of these complex tumors.
Meningiomas are the most common of the nonglial brain tumors. They account for approximately 20% of primary brain tumors. They tend to be slow growing, tend to be benign, and often can reach substantial sizes before becoming symptomatic. Complete surgical resection of intracranial meningiomas, including the dura of origin and infiltrated bone, remains the treatment of choice when able to be accomplished safely. Extent of resection has been shown to be a predictor of recurrence. Additional prognostic factors for recurrence after surgical resection include the World Health Organization (WHO) pathologic grade and the tumor cell proliferation rate. Location of a meningioma within the cranial vault may make a complete surgical resection unlikely, and tumors arising from the dura of the skull base are particularly challenging in this regard. Advances in radiation therapy, including stereotactic techniques, expand the options for treatment available in these situations. They may be used as adjuncts to surgery or as alternative modalities in the treatment of these complex tumors. The therapy for these patients can be individualized depending on several factors, including clinical presentation, patient comorbidities, tumor progression, tumor location, and involvement of adjacent neurovascular structures.
Skull base meningiomas
One of the most difficult areas in which to achieve complete resection is the basal skull. This region includes tumors that arise from the dura of the clivus and petrous bone; dura of the cavernous sinus and tentorium; sphenoid bone, including sella turcica; and the olfactory groove; and the optic nerve sheath. Approximately 20% of intracranial meningiomas reside at these regions of the skull base. In these locations they tend to present clinically with symptoms due to the proximity of adjacent vascular and cranial nerve structures or due to mass effect on intracranial contents.
Treatments of meningiomas of the skull base often have to be tailored to the local environment in which the tumor arises and the surrounding structures that find themselves involved in tumor. Because of the location of the tumor, treatment options must be weighed against the sensitivity of normal adjacent structures and by the morbidity associated with the treatment approaches required and the natural history of the tumor itself. Complexities in the clinical decision making regarding treatment for skull base meningiomas center around the risk for morbidity and from intervention and expectant management. These tumors tend to be benign and slow growing although they can be aggressive clinically and histologically.
The natural history and the tolerance of surrounding neural tissues must be considered when treatments are discussed. Bindal and colleagues addressed the issue of natural history of skull base meningiomas. They reviewed 40 patients who were diagnosed with skull base meningiomas based on radiographic studies and who were followed without treatment over a mean of nearly 7 years. Twenty-seven percent progressed neurologically, all with worsening cranial nerve deficit. No new strokes or brainstem symptoms developed. On serial follow-up imaging studies, 18% showed some degree of growth. The 2-, 5-, and 10-year radiologic evaluations showed their tumors were progression free on serial imaging at rates of 97%, 80%, and 42%, respectively. Forty percent of their patients eventually underwent some form of radiosurgical or surgical treatment. These observations need to be considered when recommendations for treatment are made. They also need to be considered when trying to assess the results of various treatment modalities. Still, with the threefold advances of imaging capabilities, microsurgical techniques, and focused radiation delivery systems, the options to individualize therapy, taking into account tumor- and patient-specific variables, have never been as great.
Surgery for skull base meningiomas
Microsurgery for meningiomas of the skull base remains a viable and in many cases an optimal treatment of this condition. The abilities of a surgeon to affect complete removal, obtain tissue diagnosis, and affect an immediate decompression of compromised neural structures with minimal new neurologic sequelae are important points in the decision-making process. Yet the ability to accomplish those goals surgically must be assessed on a case-by-case basis. Every tumor and every patient has unique characteristics that affect a decision for surgery.
Microsurgery of the skull base has been shown effective, yet there are risks for adjacent structures and there are recurrences. Current microsurgical techniques have advanced the abilities of surgeons to perform aggressive resections in the skull base area with minimal morbidity and mortality and prolongation of progression-free survivals. Complete surgical resection in all cases of skull base meningiomas with minimal morbidity remains, however, elusive. In addition, several reports evaluating pathologic specimens of skull base meningiomas have demonstrated that tumor infiltration can be seen into neurovascular structures adjacent to tumor, suggesting that there are limits to which microsurgery can achieve complete resection. De Jesus and colleagues, in the analysis of their series of 119 patients who had cavernous sinus meningiomas at a mean of 2.5 years follow-up, identified a recurrence rate of 10% in patients initially believed to have complete resection and a progression rate of 15% in patients who had initial subtotal resection. DeMonte and colleagues observed, in their 41 patients followed over a mean 45 months, 7% mortality, 7% morbidity, 10% new cranial nerve deficit, and 10% recurrence rate for patients who had complete resection. O’Sullivan and colleagues reviewed 89 patients, over a mean of 2 years, who had cavernous meningiomas of varying histologic grade. They reported a 12% incidence of new cranial nerve deficit with surgery. They were able to attain a complete resection in 8 patients who had WHO grade I tumors who had no recurrence during the follow-up period. The progression rate for the patients who had subtotal resection was 6%.
Surgery for resection of skull base meningiomas of the petroclival region involves a particularly difficult area. Natarajan and colleagues reported on the long-term results of 150 patients evaluated at a mean of 102 months after microsurgery for skull base meningiomas in this area. They reported new neurologic deficits in 20% of patients. Gross total resection was accomplished in 40% of patients. Recurrence developed in 4% of patients who had complete resection and 5% of patients who had incomplete resection. They reviewed the literature on surgery in this area and reported a range of total or gross total resection rates of between 40% and 79% with recurrence/progression rates reported as 0 to 36%. Follow-up times for the other reports are comparatively shorter, however (14–73 months).
Surgery for skull base meningiomas
Microsurgery for meningiomas of the skull base remains a viable and in many cases an optimal treatment of this condition. The abilities of a surgeon to affect complete removal, obtain tissue diagnosis, and affect an immediate decompression of compromised neural structures with minimal new neurologic sequelae are important points in the decision-making process. Yet the ability to accomplish those goals surgically must be assessed on a case-by-case basis. Every tumor and every patient has unique characteristics that affect a decision for surgery.
Microsurgery of the skull base has been shown effective, yet there are risks for adjacent structures and there are recurrences. Current microsurgical techniques have advanced the abilities of surgeons to perform aggressive resections in the skull base area with minimal morbidity and mortality and prolongation of progression-free survivals. Complete surgical resection in all cases of skull base meningiomas with minimal morbidity remains, however, elusive. In addition, several reports evaluating pathologic specimens of skull base meningiomas have demonstrated that tumor infiltration can be seen into neurovascular structures adjacent to tumor, suggesting that there are limits to which microsurgery can achieve complete resection. De Jesus and colleagues, in the analysis of their series of 119 patients who had cavernous sinus meningiomas at a mean of 2.5 years follow-up, identified a recurrence rate of 10% in patients initially believed to have complete resection and a progression rate of 15% in patients who had initial subtotal resection. DeMonte and colleagues observed, in their 41 patients followed over a mean 45 months, 7% mortality, 7% morbidity, 10% new cranial nerve deficit, and 10% recurrence rate for patients who had complete resection. O’Sullivan and colleagues reviewed 89 patients, over a mean of 2 years, who had cavernous meningiomas of varying histologic grade. They reported a 12% incidence of new cranial nerve deficit with surgery. They were able to attain a complete resection in 8 patients who had WHO grade I tumors who had no recurrence during the follow-up period. The progression rate for the patients who had subtotal resection was 6%.
Surgery for resection of skull base meningiomas of the petroclival region involves a particularly difficult area. Natarajan and colleagues reported on the long-term results of 150 patients evaluated at a mean of 102 months after microsurgery for skull base meningiomas in this area. They reported new neurologic deficits in 20% of patients. Gross total resection was accomplished in 40% of patients. Recurrence developed in 4% of patients who had complete resection and 5% of patients who had incomplete resection. They reviewed the literature on surgery in this area and reported a range of total or gross total resection rates of between 40% and 79% with recurrence/progression rates reported as 0 to 36%. Follow-up times for the other reports are comparatively shorter, however (14–73 months).
Radiation therapy for skull base meningioma
Historically, radiation therapy has been shown a reasonable adjunct to surgical resection, providing enhanced local control of tumor growth for benign meningiomas. Barbaro and colleagues in 1987 reviewed their series, which included all types and grades of meningiomas, and showed an improvement in progression-free survival at 6.5 years with external-beam fractionated radiotherapy after subtotal resection when compared with subtotal resection alone (68% versus 40%).
In the ensuing years, advancements in imaging and improvements in radiation delivery methods have enhanced abilities to conform the dose of radiation ever more precisely to tumor, have allowed for more intense tumor dosing, and have allowed for reduction in radiation to nontumor structures. These concepts have been particularly advantageous for tumors that arise at the skull base. The proximity of important radiosensitive neurovascular structures, brainstem, and pituitary gland to tumor margins requires that radiation to skull base lesions be delivered in a way that provides therapeutic benefit while maintaining enhanced margins of safety for adjacent tissues. Further improvements in conformality have been achieved with the introduction of focused radiation techniques, including stereotactic radiosurgery (SRS) and stereotactic radiotherapy (SRT); each may be delivered by gamma photons, x-ray photons, or charged particle beam therapy.
Stereotactic therapy is the term used to identify treatment modalities that use high-dose radiation, delivered from multiple beam locations, to precise targets registered within a defined 3-D space. Stereotactic localization uses high-quality imaging to identify tumor, tumor margins, critical neurovascular structures, and identifiable fiducial points, which then are digitally processed to register a patient and anatomy into a defined 3-D space. Stereotactic therapy includes a mechanism of radiation accuracy assurance during treatment. Repetitive image localization during treatment or fixation of a patient and target throughout the treatment are used as methods to confirm the accuracy of the radiation dose delivered.
Radiation Sources
The radiation sources for SRS or SRT that are used most commonly in clinical practice are the gamma photons generated from the decay of cobalt-60 or the x-ray photons generated from a linear accelerator (linac). Cobalt-60 decays with the release of two gamma photons. The isotope is housed in such a way as to allow the release of only those photons emitted in a predetermined direction. A series of cobalt sources are arranged to deliver multiple gamma photons to a single convergent point. Targeting then is accomplished by adjusting a patient’s position so as to place the tumor into the point of convergence. The isotope has a half-life of 5.28 years. The sources typically are replaced every 5 to 8 years because the treatment times increase as the sources age.
Linear Accelerators
Linear accelerators can generate x-ray photons by the collision of accelerated electrons and a target metal. The resulting photon beam exits the generator following a linear path. The beam then can be directed by moving the accelerator into the proper position. Targeting is accomplished by positioning the accelerator to allow the beam to pass through the target site from multiple entrance trajectories. These radiation beams, gamma radiation and x irradiation, are characterized by having high enough energy to penetrate the cranial tissues allowing for treatment of the intracranial contents. Additionally, they deposit radiation over the entire length of the beam, including both target tissue and nontarget tissue proximal and distal to the target. By arranging multiple portals for the delivery of these radiation beams and having them all converge on the exact same target point, the target can accumulate significant radiation exposure while the dose delivered to nontarget tissues is minimized. A steep gradient of radiation dosage is generated with a substantial falloff of the dose measured at distances adjacent to and distal to the target. In addition to varying the location of beam delivery, current delivery systems allow gamma photon beams and x-ray photon beams to be varied in their intensity along each particular delivery path, providing variable dosing per exposure and greater opportunities for dose contouring. These techniques add to the ability to minimize the dose to nontarget tissues while maintaining the planned target dose accumulation.
Particle Therapy
Particle therapy refers generally to beams of heavier charged particles used as radiation sources. These particles include protons (hydrogen nuclei), helium nuclei, and carbon nuclei. Using a cyclotron, these beam sources are generated by the stripping of the underlying atoms of their electrons and accelerating the charged nuclei to a variable exiting energy level. The particle beam then is released in the intended direction of the target. As with photon-based therapies, particle therapies vary the direction and the intensity of the beam entering the patient to improve conformality of the dose to the tumor. Because the particles have mass and charge, they can be focused magnetically. Most importantly, particle beams release their energy essentially completely at a single interaction point at a predetermined depth within the target tissue. This characteristic is known as the Bragg peak effect. By varying the intensity of the beam as it leaves the cyclotron, the distance the particle beam travels in tissue before discharging its energy can be varied. Because so little energy is delivered beyond the length of the Bragg peak, the radiation exposure is decreased to the surrounding nontarget tissues. This allows for increased conformality of the radiation dose and provides a theoretically improved radiobiologic profile.
Focused radiation and fractionation
SRS, then, is a radiation delivery method that uses multiple radiation beams focused in a 3-D manner within a defined target space (stereotactically) to a single target. Typically, the term, radiosurgery , refers to treatment performed in a single fraction, although up to five fractions may be considered radiosurgery according to convention. The radiation may be generated from fixed sources, such as the gamma knife unit. In this unit, 201 fixed, cobalt-60, gamma ray–emitting sources housed in cast iron are preset in a spherical array focused to the unit’s isocenter. A series of tungsten helmet collimators are used to further focus the individual sources. Patients are immobilized in a rigid titanium stereotactic frame applied to the skull in four-point pin fixation. The pins secure the frame with tension applied to the outer table of the skull. A fiducial box is attached to the frame, and the patient then undergoes stereotactic MRI or CT scanning or both for localization of the target and adjacent structures. Other relevant images (positron emission tomography, functional MRI, or diffusion tensor imaging) may be merged to the stereotactic images as necessary. Once targets are identified and digitized and a treatment plan optimized, the patient is attached via the rigid frame to the gamma knife unit in such a manner as to place the patient’s target in the machine’s isocenter. The table then moves the patient into the focal point of the sources and the radiation for that isocenter is delivered. Depending on the complexity of the plan, the patient may require several isocenter exposures to complete the treatment plan. Once the treatment plan is completed, the head frame is removed.
The radiation output for SRS also may be generated as x rays from a nonfixed linac. In this instance electrons are accelerated and then collide with a metal target generating a beam of x-ray photons. The photon source then is rotated in multiple arcs around the patient lying on the treatment table, positioning the x-ray beam in multiple orientations focused to a fixed isocenter. This method generates a spherical distribution at the isocenter. Tertiary collimators can be added to the beam to adjust the dose at each position, allowing for a nonuniform, or shaped, isocenter. For 3-D localization, the patient may be immobilized by a stereotactic rigid frame applied to the skull, implanted with attached fiducials, or registered with a frameless system in which repeated x-ray imaging confirms patient position throughout the treatment. A high-resolution, stereotactic MRI or CT is performed with an attached fiducial reference frame. The images are used for target localization and treatment planning. Depending on the complexity of the tumor and the plan, there may be a single isocenter used to treat the tumor or multiple isocenters. In the treatment paradigms that use rigid fixation, the radiation dose delivered can be contoured to the margin of the tumor itself. No additional treatment beyond the margin is needed.
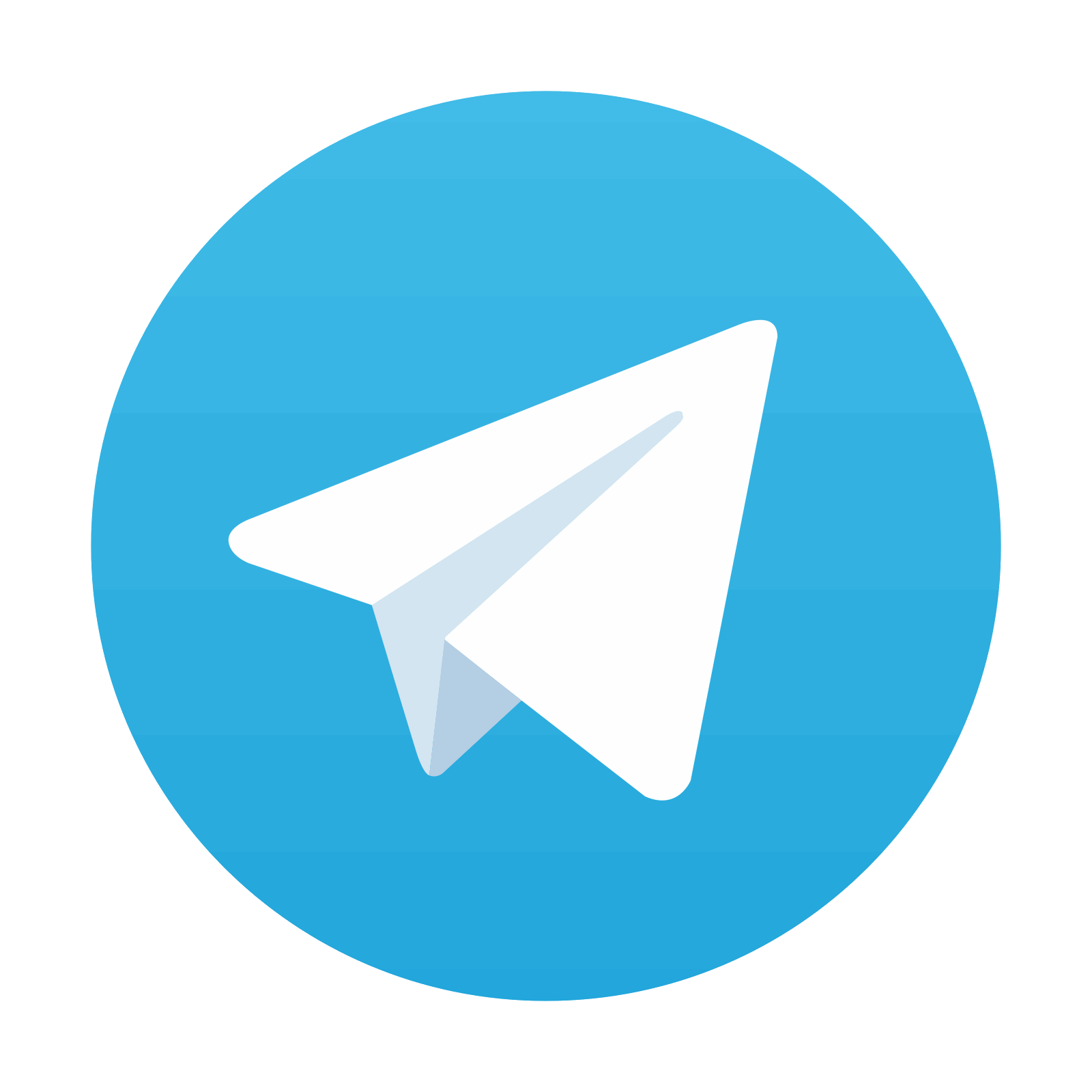
Stay updated, free articles. Join our Telegram channel

Full access? Get Clinical Tree
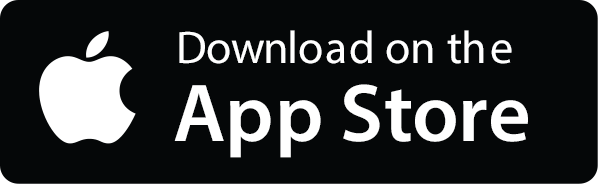
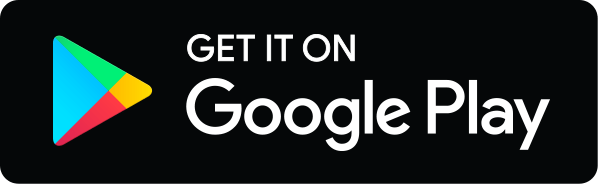