Cause
Clinical features
Diagnostic test
Impaired intestinal calcium absorption
Low dietary intake
Avoidance of dairy products/ obtained via history
24 h urine calcium assessment
Lactose intolerance
Abdominal pain, bloating, flatulence, diarrhea, bulky, frothy, and watery stools
Known history of lactose intolerance
Lactose tolerance test
Lactose Breath Hydrogen tests
? genetic testing
Decreased intestinal absorption
Celiac disease
Diarrhea, weight loss, associated autoimmune diseases such as Crohn’s disease, T1DM
Transglutaminase antibodies
Endomysial Ab
Intestinal Biopsy
Evaluate for iron and B12 deficiencies
Pancreatic steatorrhea
Mild GI symptoms
Stool studies for fat
Evaluate for iron and B12 deficiencies
Vitamin D deficiency
Sunlight deprivation
Lack of sun exposure especially high-risk groups (elderly, nursing home residents) religious beliefs
Use of sunscreens
25OHD
Intestinal Vitamin D malabsorption
Liver disease
Hepatic function
Loss of calcium related to the following disease
Bone
History of bisphosphonate use in:
Paget’s disease
Osteoporosis
Bone metastasis
NA
Lactation
Obtained via history/recent history of weaning
NA
Kidney
History of kidney stones, loop diuretics, family history of kidney stones
24 h urine studies for calcium, sodium, phosphorus, oxalate, citrate
Soft tissue damage
Traumatic muscle damage, ICU stay, extensive burns
CK levels
Impaired PTH secretion
Renal failure
Pruritus, anuria, uremic symptoms, anemia
Creatinine, phosphorus, blood urea nitrogen
Pseudohypoparathyroidism
Albright phenotype
Family history
Tetany
Genetic testing
In the case of SHPT due to renal disease, additional history of renal insufficiency, serum creatinine, phosphorus, BUN, assessment of urine output, and comorbidities is essential. The measurement of PTH is required and is typically high in the presence of a normal to mildly low serum calcium concentration. Due to the chronicity of the disease, serum albumin should be routinely measured to obtain a corrected calcium level. Elevated serum calcium excludes SHPT of renal origin.
Guidelines for the Management of SHPT
Treatment of SHPT is aimed at minimizing all-cause morbidity and mortality, abnormal mineral metabolism, and bone disease as well as preventing extra-skeletal calcium deposits including vascular calcification.
In 2009, the Kidney Disease: Improving Global Outcome (KDIGO) developed practice guidelines which provided recommendations for the evaluation and management of chronic kidney disease-mineral and bone disorder (CKD-MBD) . The KDIGO does not recommend specific targets for PTH based on the stage of renal disease. They recommend using intact PTH as an assay. There is a greater momentum towards early detection and treatment of SHPT. In CKD stage III {GFR 30–59 mL/min/1.73 m2] PTH should be monitored every 12 months along with annual calcium and phosphorus assessments. Therapy should be initiated if the PTH rises steadily or persistently remains above the upper limit of normal. In CKD stage IV [GFR 15–29 mL/min/1.73 m2], PTH measurements are recommended every 3 months. In CKD V not yet on dialysis, PTH should be assessed every 3 months while calcium and phosphorus levels are assessed monthly. The goal is to maintain calcium and phosphorus levels within the normal range.
In 2003, the Kidney Disease Outcome Quality Initiative KDOQI specified target goals for PTH, calcium, and phosphorus. For patients with CKD stage V [below 15 mL/min/1.73 m2], the KDOQI guidelines recommended a target intact PTH (first-generation immunometric assay) of between 150 and 300 pg/mL, calcium phosphorus product of less than 55, serum calcium in the lower half of the normal range [8.4–9.5 mg/dL] and serum phosphorus between 3.5 and 5.5 mg/dL. The standard treatments used in order to achieve these targets were limited by drug side effects and thus these goals remained largely unmet [9].
The newer KDIGO CKD-MBD guidelines have used more of an evidence-based approach and have individualized treatment and represent the most current clinical management guidelines. Target PTH levels should be based on the levels of renal dysfunction to avoid high bone turnover and maintain near normal levels of alkaline phosphatase. This goal can be achieved by keeping PTH near the upper limit of normal in CKD stages 3–4 and up to nine times normal in the dialysis population [10]. However, a uniform classification system in this area is lacking.
Current KDIGO guidelines do not recommend routine measurements of bone mineral density in CKD, although suggest that serum PTH or bone-specific alkaline phosphatase levels should be assessed to predict bone turnover [10].
Bone biopsy is the gold standard procedure to assess CKD-MBD but is invasive and not widely available. Vascular calcification can be assessed by lateral abdominal radiograph, echocardiogram, or computed tomography (CT) scanning.
Treatment of SHPT
The goal of treatment is to lower PTH while maintaining normal calcium and phosphorus levels. This is often times very challenging due to the complex interrelationship of hormones, bone health, diet, and mineral balances within the body. It is to be kept in mind that the treatment options discussed below improve biochemical parameters and bone histology although the evidence on the impact on patient-related outcomes is lacking.
The use of standard treatment measures such as calcium supplementation, phosphate binders, and vitamin D analogs are limited by the development of hypercalcemia, hyperphosphatemia, increased Ca × P product with resultant increase in vascular calcifications.
Calcium Supplements
Calcium supplements are only moderately effective in controlling PTH levels in those with SHPT on dialysis or early stages of renal failure. Calcium carbonate alone versus oral or IV calcitriol did not show a significant decrease in the levels of PTH [11, 12]. High doses of calcium supplementation are limited by calcifications that occur once the Ca X P product exceeds 55. Calcium supplements are limited to 1–2 g per day due to this concern.
Restriction of Dietary Phosphorus and Phosphate Binders
The mainstay of treatment is the correction of hyperphosphatemia and prevention of a positive phosphate balance. This in turn limits the development of hyperparathyroidism and its related effects.
Phosphate control is still an unmet need in CKD. Serum phosphorus is an independent risk factor for mortality in ESRD [13, 14] In mild CKD , restriction of dietary phosphate reduces PTH levels [15]. Phosphate restriction can be achieved via eliminating food preservatives and other additives. In order to maintain a balance between protein and phosphorus levels, foods with high biological value such as eggs and meats are preferred. Phosphorus intake should be limited to 900 mg/day.
In addition to dietary interventions, phosphate binders are used to control serum phosphate levels. As the disease advances, dietary restriction alone is insufficient to reduce hyperphosphatemia and the resultant SHPT. The currently available phosphate binders bind about 250 mg/day of phosphate [16]. With use of a single phosphate binder, about 30–50 % of ESRD patients remain hyperphosphatemic. Combination therapy with two different binders, increases the phosphate binding capacity and maintains serum phosphorus levels within acceptable ranges. Both calcium and non-calcium-based phosphate binders have been used in the treatment of SHPT. Aluminum is no longer used due to its serious toxicities. The use of calcium-based binders increase the risk of hypercalcemia, calciphylaxis, and vascular calcification [17]. The calcium free phosphate binders such as, sevelamer and lanthanum carbonate, decrease serum phosphate levels without causing hypercalcemia but they are not potent in lowering PTH levels [18]. Ferric citrate is an iron-based oral phosphate binder that effectively lowers serum phosphorus levels and has been shown to have safety profile similar to sevelamer and calcium acetate in hemodialysis patient [19]. Niacin reduces phosphate absorption by blocking the active sodium-phosphate co-transporters in the small intestines and has shown promising results [20]. Calcium containing binders are less costly and readily available and can be used in patients with CKD who have hypocalcemia. Non calcium containing phosphate binders are preferred in individuals with CKD and normal or high calcium levels.
Calcium as well as non-calcium-containing phosphate binders were shown to comparably lower FGF23 levels [21]. Overall, both calcium and non-calcium phosphate binders are shown to be effective in lowering phosphorus levels but the impact of these agents on all-cause mortality and cardiovascular mortality in CKD is unclear [22]. In a recently published meta-analysis, non-calcium-containing phosphate binders were associated with lower all-cause mortality [23]. Available phosphate binders can increase calcifications in coronary artery and abdominal aorta [24]. Dietary phosphate restriction, with or without calcium carbonate treatment, resulted in progression of vascular calcifications , although this effect was not seen in those treated with sevelamer [25]. However, data supporting improved clinical outcomes by limiting the progression of vascular calcifications is lacking.
Vitamin D and VDR Agonist
Adequate levels of vitamin D are required for intestinal absorption of calcium. In the presence of low vitamin D, intestinal absorption of calcium is reduced which results in elevated PTH levels and subsequently parathyroid gland hyperplasia [26]. In CKD stages 3–4, calcium and phosphorus levels are usually in the physiologic range [27]. The majority of patients with CKD have low vitamin D [28]. The KDOQI guidelines recommend correction with vitamin D especially in stages 3 and 4 as these low levels may trigger the development of hyperparathyroidism. Supplementation of vitamin D with either ergocalciferol or cholecalciferol increases the level of 25HD and 1,25 (OH)D2 and may suppress but not necessarily normalize PTH in stages 3–4. In Stage 5, supplementation with vitamin D is generally ineffective in suppressing PTH levels.
The deficiency of endogenous calcitriol production is often treated with biologically active VDR agonist such as calcitriol, paricalcitol, alfacalcidol (not approved for use in the United States), and doxercalciferol. These active sterols increase the absorption of calcium and phosphorus from the intestines and in turn decrease the synthesis of PTH in a dose dependent manner regardless of the stage of CKD. Dialysis patients have impaired uptake and metabolism of 25HD. Calcitriol not only replete the levels of 1,25-D but also increases the uptake of 25HD [29]. However, These drugs are limited by the development of hypercalcemia and hyperphosphatemia, especially at higher doses and have a narrow therapeutic index [30]. The currently available synthetic analogs reduce PTH to a similar extent although paricalcitol achieves this reduction sooner than the other drugs [31]. In addition, paricalcitol showed significant and sustained control of PTH, with fewer episodes of hypercalcemia [32].
Intravenous calcitriol has been used since the late 1980s as an alternative therapy to either oral calcitriol or parathyroidectomy in adult dialysis patients with SHPT. Long term treatment showed reductions in PTH as well as alkaline phosphatase [33]. In addition, lowering PTH was also shown to be cardioprotective [34]. Widespread use of intravenous calcitriol has resulted in fewer parathyroidectomies [35].
Low vitamin D and 1,25 (OH)D2 levels correlate with increased cardiovascular disease and deaths, while the use of VDR agonist therapy may be cardioprotective. There are few prospective studies evaluating the effects of VDR agonist on survival. Previous meta-analysis showed Vitamin D supplementation was beneficial in lowering cardiovascular and all-cause mortality in patients with CKD [36]. Paricalcitriol has a greater survival advantage over calcitriol [36]. Contrary to the above, current evidence on Vitamin D supplementation does not support a benefit in survival or cardiovascular mortality in patients with CKD [37].
Continuous use of a VDR agonist results in lowering PTH, preserving bone mass, and lowering markers of bone remodeling such as bone specific alkaline phosphatase and osteocalcin [38, 39]. There is a lack of data on the effectiveness of the interrupted use of VDR agonist. Continuous therapy is recommended in order to maintain PTH suppression.
Therapy and goals for optimal PTH suppression need to be individualized. Occurrence of hypercalcemia due to VDR agonist may suggest the development of adynamic bone, a form of renal osteodystrophy characterized by a low bone turnover state [40]. VDR agonists can be used to achieve PTH suppression while ensuring that hypercalcemia and hyperphosphatemia do not occur.
Calcium-Sensing Receptor Agonist: Cinacalcet
The calcium-sensing receptor (CaSR) is a G protein-coupled receptor located on the parathyroid chief cell membrane and represents the pivotal mechanism regulating PTH secretion. This receptor was initially cloned from bovine parathyroid cells and described by Brown et al. [41]. Activation of this receptor by an increase in extracellular calcium, the endogenous ligand, decreases PTH secretion [42]. The lack of a drug capable of directly altering PTH secretion without affecting serum calcium levels made the CaSR a high priority molecular target [43].
Ligands that simulate or potentiate the effects of extracellular calcium at the CaSR have been termed calcimimetics. There are two mechanistically distinct types. Type I calcimimetics are inorganic and organic polycations that act as agonists. Type II calcimimetics are l-amino acids and phenylalkamines that function as allosteric activators [44]. These type II drugs interact with membrane-spanning portions of the CaSR and induce conformational change in the receptor. This conformational change lowers the threshold for CaSR activation by plasma calcium, thereby reducing PTH secretion without a change in the serum calcium level [45].
The first-generation calcimimetic drug candidate was NPS R-568, a phenylalkamine type II compound. NPS R-568 was shown to selectively activate the parathyroid CaSR and inhibit PTH secretion both in vitro and in vivo [46]. However this compound demonstrated a variable pharmacokinetic and molecular profile [47]. This prompted the development of cinacalcet HCl, or (αR)-(−)-α-methyl-N-[3-[3-[trifluoromethylphenyl]propyl]-1-napthalenemethanamine hydrochloride, a second-generation analog of NPS R-568 that possesses the same safety and efficacy with improved bioavailability.
Cinacalcet (Sensipar, Amgen, Thousand Oaks, CA) has been approved by the FDA for use in the US since 2004. Its indications include (1) treatment of SHPT in adult patients on dialysis for chronic kidney disease, (2) hypercalcemia in patients with parathyroid carcinoma, and (3) severe hypercalcemia in patients with primary HPT.
In SHPT, cinacalcet treatment results in a decrease in FGF23 levels and the effect on FGF23 is independent of the changes in PTH levels [48]. Orally administered cinacalcet has been shown to reduce PTH and this effect can be maintained long term [49]. In CKD patients not on dialysis, cinacalcet decreases the levels of PTH but can cause hypocalcemia, hyperphosphatemia, and hypercalciuria. Therefore close monitoring of these laboratory values is required [50, 51].
Long-term administration of cinacalcet is associated with reduced progression of abdominal aortic calcification and uremic arteriolopathy [52]. Appropriate calcium and phosphorus levels may be achieved and together these changes reduce the rates of cardiovascular events and mortality in patients on hemodialysis [52, 53]. Cinacalcet did not reduce the risk of death or cardiovascular morbidity in those individuals on hemodialysis with moderate to severe hyperparathyroidism [54]. Nevertheless, addition of cinacalcet to standard therapy in adults with EGFR < 15 % (Stage 5) disease who were on dialysis, helped prevent surgical parathyroidectomy .
Cinacalcet resulted in a higher incidence of hypocalcemia. The data is robust for individuals with EGFR < 15 but sparse for those with EGFR between 15 and 60 ml/min/1.73 m2 and kidney transplant recipients [55]. Cinacalcet does not reduce the rate of clinical fractures in patients on hemodialysis [56].
Combination Therapy
Low-dose cinacalcet plus calcitriol is more effective than calcitriol alone for the treatment of moderate and severe SHPT in chronic dialysis patients. Combination therapy resulted in fewer episodes of hyperphosphatemia and hypercalcemia [57]. When combined with VDR agonist, cinacalcet has been shown to reduce the parathyroid gland volume [58].
Primary Hyperparathyroidism
A growing body of evidence exists to support the efficacy of cinacalcet in lowering plasma PTH and serum calcium levels in patients with PHPT [62, 63]. A phase 3 multi-center trial demonstrated that cinacalcet resulted in a significant plasma PTH reduction and serum calcium normalization compared to placebo [64]. Cinacalcet is effective and durable for multiple years for PHPT patients with varying degrees of disease severity [65]. In patients with PHPT and nephrolithiasis, the addition of cinacalcet to standard therapy and dietary measures led to a significant reduction in both the number and size of renal stones [66]. However, bone mineral density does not improve with cinacalcet therapy [67].
Cincalcet has also been shown to correct hypercalcemia and hyperparathyroidism in kidney transplant recipients [68].
Recent data have emerged to indicate that patients with mild, asymptomatic PHPT who do not meet surgical criteria for parathyroidectomy experience more effective biochemical control with cinacalcet compared to patients with surgical criteria. Patients without surgery criteria are more likely to reach normocalcemia at the end of the initiation phase, they maintain significantly lower serum calcium levels throughout treatment, and they experience stronger reductions in PTH level when compared to patients with surgical criteria [69]. This represents an active area of investigation, as neither the FDA nor the European Medical Agency currently approves cinacalcet for the treatment of PHPT with surgical criteria.
Surgical Intervention
Parathyroidectomy for SHPT is generally considered if the serum PTH levels are >1000 pg/ml with associated hypercalcemia, the volume of at least one hyperplastic gland is >500 mm3 or SHPT is refractory to treatment.
Patients undergoing parathyroidectomy achieved the target KDOQI ranges 14–43 % of the time for calcium and 65–76 % for phosphate. However, most patients had a PTH below target [70]. Several smaller studies have looked at the survival benefit of parathyroidectomy versus medical treatment and have shown better cardiovascular and all-cause mortality with surgical intervention [71, 72]. There is ongoing debate about the preferred method of surgery; subtotal parathyroidectomy versus total parathyroidectomy with auto transplantation [73]. Currently total parathyroidectomy with or without autotransplantation is considered safe in patients with uncontrolled SHPT.
Summary
In this chapter, we have reviewed the etiology and treatment of secondary hyperparathyroidism. Secondary hyperparathyroidism is characterized by elevated PTH levels for an appropriate stimulus of hypocalcemia. In chronic kidney disease, the elevations in PTH fail to maintain calcium and phosphorus homeostasis. Current treatment options aimed at maintaining the phosphate balance and prevention of hyperphosphatemia. The new KDIGO guidelines do not recommend a specific target range for PTH, calcium, or phosphorus but rather individualize targets based on renal dysfuntion.
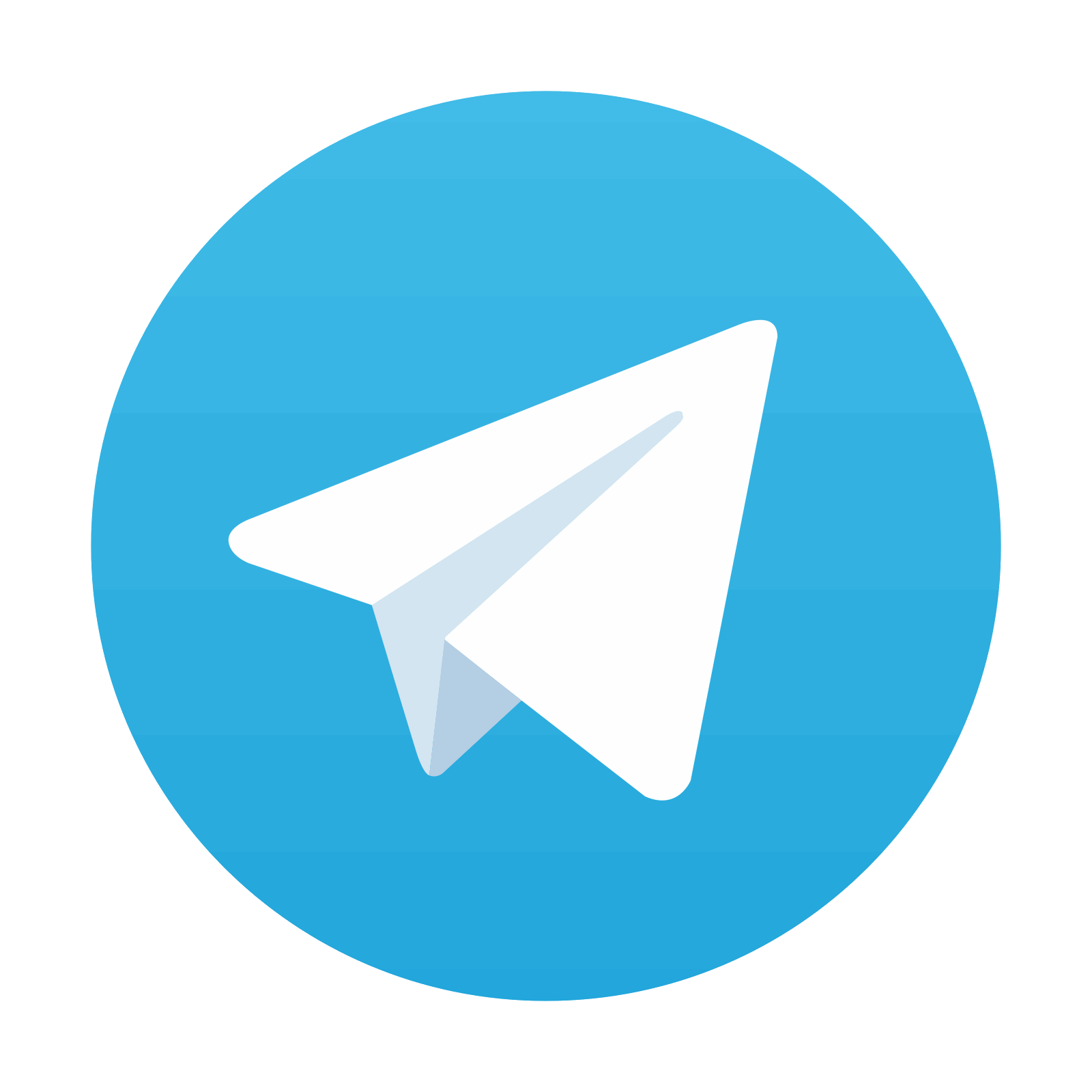
Stay updated, free articles. Join our Telegram channel

Full access? Get Clinical Tree
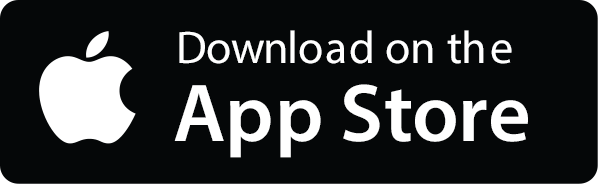
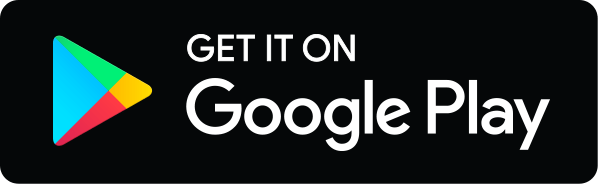