Fig. 14.1
Saliva flow rate at 4 and 8 weeks after irradiation. (a) The saliva flow rate was measured in 10-min intervals for 30 min after pilocarpine injection. (b) Saliva flow rate for 30 min after pilocarpine injection. The normal, nonirradiated, and untreated group is indicated by the black squares. Group I was treated with basic fibroblast growth factor after irradiation, which is indicated by black circles. Group II was not treated after irradiation, which is indicated by white circles. In the 4-week comparison: normal (13 weeks old, n = 8), Group I (n = 8), Group II (n = 8). In the 8 week comparison: normal (17 weeks old, n = 8), group I (n = 8), group II (n = 8) (Image from Published Paper [22])
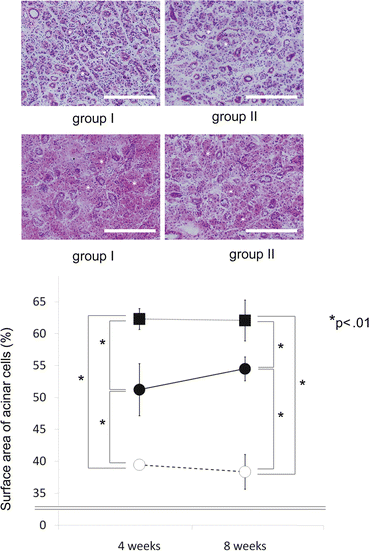
Fig. 14.2
Histologic findings. (a) Hematoxylin and eosin staining at 4 weeks after irradiation. (b) Periodic acid-Schiff staining at 4 weeks after irradiation. Scale bar: 200 lm. Asterisk indicates acinic cells. (c) The percentage of the surface area comprised of acinar cells was calculated from four mice in each group. Black squares indicated the normal, nonirradiated, and untreated group. Black circles indicate Group I. These animals were treated with basic fibroblast growth factor after irradiation. White circles indicate Group II. These animals were untreated after irradiation (Image from Published Paper [22])
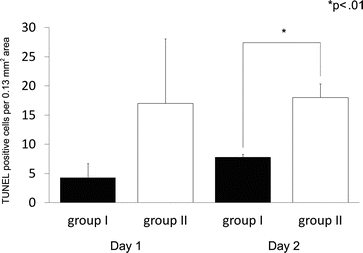
Fig. 14.3
Terminal deoxynucleotidyl transferase–mediated deoxyuridine triphosphate nick-end labeling (TUNEL)–positive cells. TUNEL-positive cells were counted in five randomly chosen fields from each mouse. The number of scored cells was averaged for each mouse. Five samples were counted for each group and day. Group I were treated with basic fibroblast growth factor after irradiation. Group II were untreated after irradiation (Image from Published Paper [22])
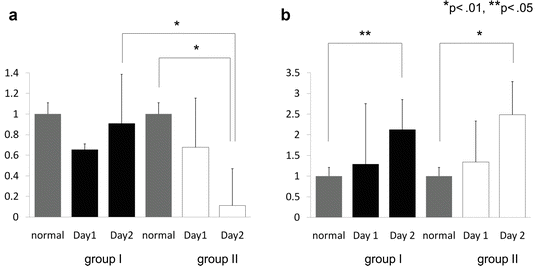
Fig. 14.4
Expression of hepatocyte growth factor (HGF) and vascular endothelial growth factor (VEGF) mRNA. Quantitative real-time polymerase chain reaction was performed in a mixture of cDNA samples, TaqMan Universal PCR Master Mix, and the TaqMan probes and primers for HGF (a) or VEGF (b). The gray bar indicates expression in the normal, nonirradiated, and untreated group. Group I (black bar) was treated with basic fibroblast growth factor after irradiation. Group II (white bar) was untreated after irradiation. Day 1 indicates 1 day after irradiation, and Day 2 indicates 2 days after irradiation. Four samples were assayed from each group and day (Image from Published Paper [22])
Other studies have shown similar results using molecular factors. Maedina et al. reported a complete reversal in radiation-induced hyposalivation through the use of histamine [27]. They demonstrated that appearance and structure was preserved, and speculated that histamine may suppress apoptosis and prevent suppression of cellular proliferation [27]. Another protein that shows radioprotective properties is heat shock protein 25. Lee et al. demonstrated its ability to inhibit radiation-induced apoptosis and mediate radiation-induced fluid loss in the salivary gland although radiation inhibits salivary gland aquaporin 5 expression [28]. Similarly, Zheng et al. demonstrated the prevention of salivary hypofunction with the use of a keratinocyte growth factor (KGF) gene transfer model. KGF promotes an increase in endothelial cell number, augments cellular proliferation, and increases the number of salivary gland progenitor stem cells [29, 30]. Additional research efforts in preventing acute radiation-induced damage include hyperbaric oxygen therapy [31], cytokine treatment [32], and injection of the heparan sulfate mimetic regenerating agent OTR4120 [33].
The above studies show the importance of protecting salivary gland progenitor stem cells from radiation-induced free radicals. Although the cytoprotectant, amifostine, is approved by the Food and Drug Administration with proved efficacy for cancer treatment, it does not constitute the standard treatment due to severe side effects [34]. Given that a priority is placed on treating cancer rather than side effects associated with radiation, it is challenging to find effective, side effect-free regimens. However, priority in devising this type of regimen is essential, unless salivary gland cells can be regenerated. Finding a solution to radiation-induced damage is important.
14.5 Regeneration
Because it is very difficult to reverse salivary gland dysfunction due to radiation or Sjögren’s syndrome, several strategies have been proposed to mediate its effects. These include gene therapy, tissue engineering, and cell therapy, all of which are considered excellent models for regenerating complex tissues structures and restoring function. From a gene therapy approach, Baum et al. developed a novel way to use aquaporin-1 cDNA as a treatment strategy in current patients with radiation-induced salivary dysfunction [35, 36]. He devised a recombinant adenoviral vector to mediate the gene transfer and administered it to patients through a phase I/ II clinical trial. From a tissue-engineering standpoint, a three-dimensional (3D) culture system using hyaluronic acid (HA) hydrogels was created to artificially simulate the salivary gland. The seeded cells produced lobular-like acini that stained positive for alpha-amylase [37, 38]. Furthermore, poly-lactic-co-glycolic acid (PLGA) has been utilized as a scaffold to promote the self-organization of cells into a 3D construct [39–41]. Ogawa et al. has demonstrated the successful replacement of salivary gland function through the transplantation of a bioengineered salivary gland germ [42]. These innovative strategies demonstrate the potential of inducing salivary gland regeneration through tissue engineering techniques. The utilization of stem cell therapy, however, appears to hold the greatest promise. Stem cell therapy has already demonstrated successful gain of function in irradiated salivary glands. Stem cells, characterized by their ability to yield substantial cellular proliferation and the differentiation into various cell types, can be harvested from tissue-specific sources or non-tissue-specific sources. The latter represents a more useful way to collect the number of cells required to demonstrate an efficacious result. Lombaert et al. has shown that bone marrow-derived stem cells (BMSCs) ameliorate acinar cell loss and vascular damage [43]. Sumita et al. demonstrated that BMSCs, once transplanted into the mouse-tail vein, also led to the regain of function in irradiated salivary glands [44, 45]. Our research group reported previously that adipose tissue-derived stem cells (ADSCs) can ameliorate radiation-induced salivary dysfunction [46]. ADSCs represent an additional source of stem cells given the feasibility in collecting large amounts of these cells through the use of minimally invasive procedures compared to the harvesting of BMCs [47]. Use of ADSCs in our study led to an improvement in salivary flow rate compared to the sham group (Fig. 14.5). Furthermore, the proliferation of blood vessels (Figs. 14.6 and 14.7), paracrine effects (Fig. 14.8), and the differentiation of ADSCs into blood endothelial cells and ductal cells were observed (Fig. 14.9). Use of ADSCs appears to result in angiogenesis, improved production of mucin and amylase, decrease in number of atrophied acinar cells, reduction in fibrotic scarring, and improved blood flow. More recently, Lim et al. demonstrated the differentiation of infused ADSCs into salivary gland cells, highlighting the remarkable capability that stem cell research holds in treating radiation-induced salivary dysfunction [47].
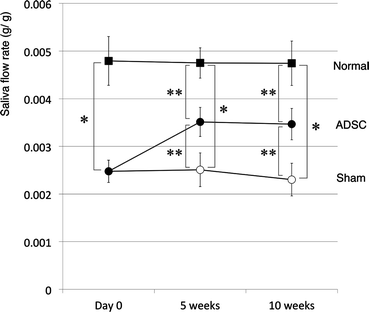
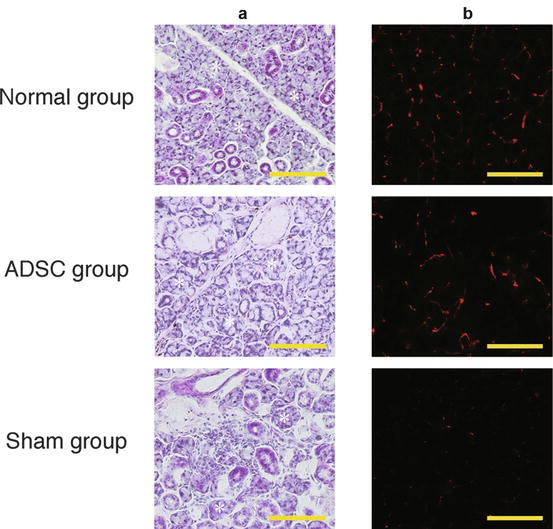
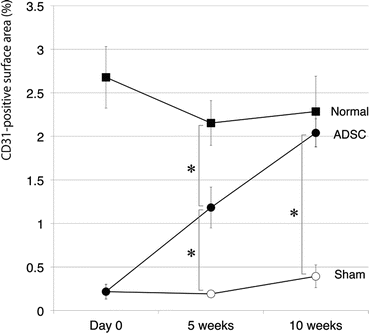
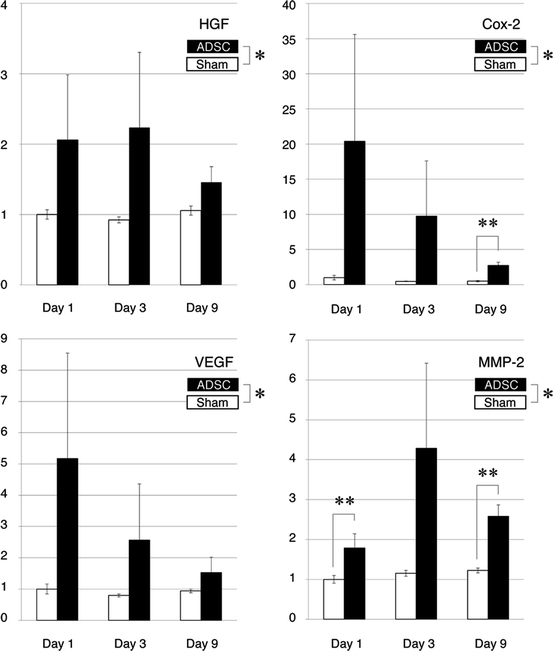
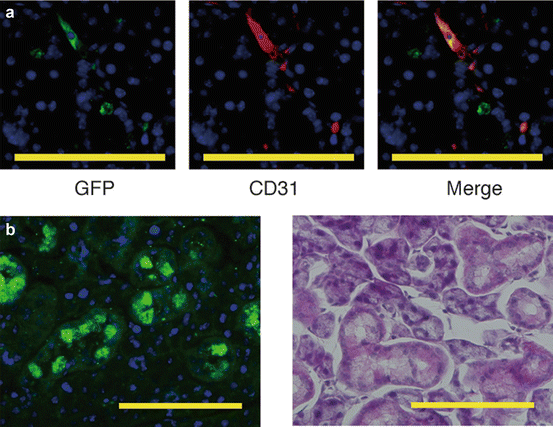
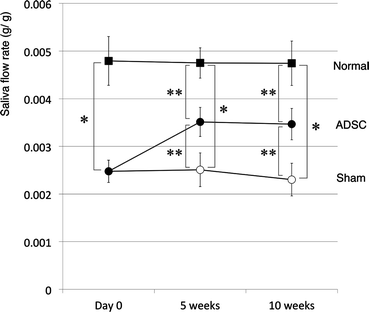
Fig. 14.5
Saliva flow rate at days 0, 5, and 10 weeks after irradiation. The saliva flow rate was assessed for 30 min after pilocarpine injection. Normal group (black square); ADSC group (black circle): sham ADSC group (white circle). *P < .01, **P < .05, one-way factorial ANOVA, post hoc Fisher PLSD (Image from Published Paper [46])
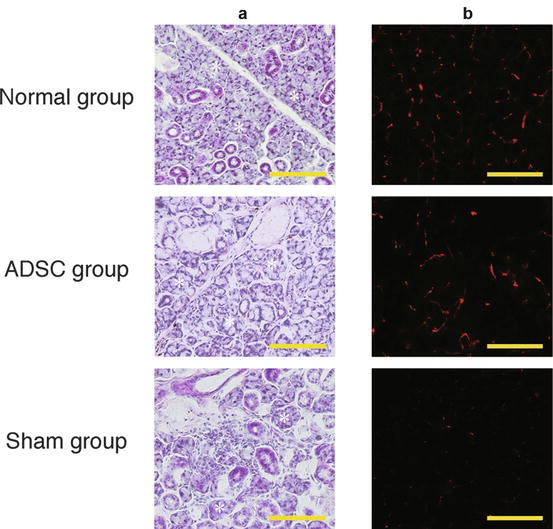
Fig. 14.6
Improvement in salivary gland tissue morphology and angiogenesis. H&E staining (a) and immunohistochemistry for CD31 (b, red signal) is shown at 10 weeks after administration/transplantation. Scale bar: 100 μm; *Acinic cells (Image from Published Paper [46])
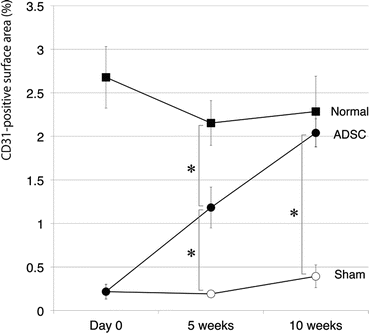
Fig. 14.7
Percentage of CD31-positive surface area in the salivary glands. The percentage of the total surface area positive for CD31 immunoreactivity was measured and calculated in 10 randomly chosen fields from sectioned salivary glands from each mouse. The percentage of the scored area was averaged for each mouse. *P < .01, one-way factorial ANOVA, post hoc Fisher PLSD (Image from Published Paper [46])
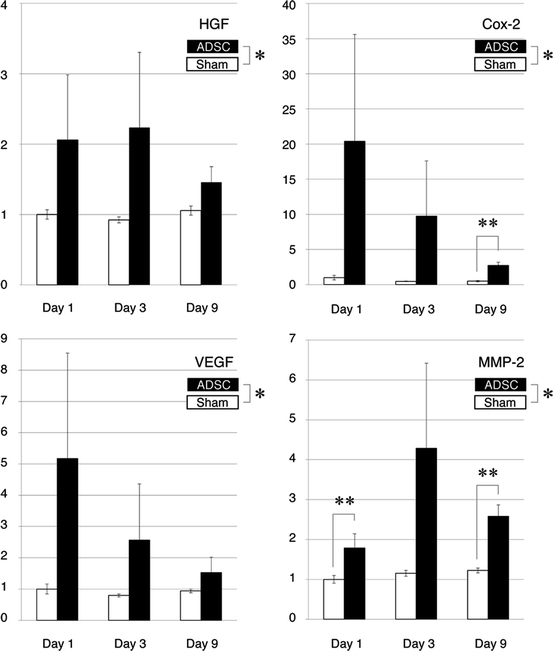
Fig. 14.8
Paracrine effects of ADSCs. Quantitative RT-PCR was performed in a mixture of cDNA samples, TaqMan® Universal PCR Master Mix, and the TaqMan® probes and primers for HGF, VEGF, Cox-2, and MMP2. Black bar (ADSC): ADSC group; white bar (Sham): sham group. Day 1 indicates 1 day after the administration of saline or ADSC cells; Day 3 indicates 3 days after administration; Day 9 indicates 9 days after administration. Five samples were assayed from each group and day. *P < .01, two-way factorial ANOVA, post hoc Fisher PLSD. **P < .01, Student’s t-test (Image from Published Paper [46])
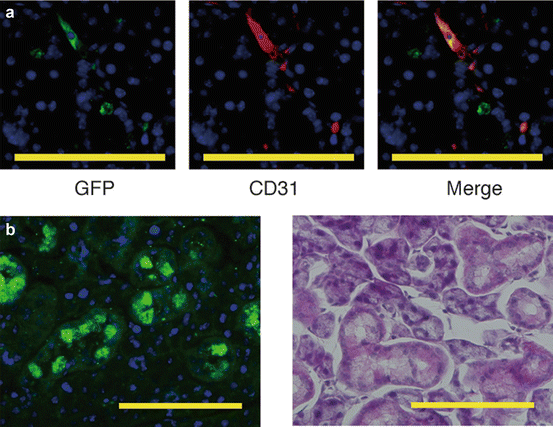
Fig. 14.9
Differentiation of transplanted ADSCs into blood endothelial cells and ductal cells. (a) Immunohistochemistry for GFP expression was used to identify transplanted ADSCs, and CD31 expression was used to identify blood endothelial cells. The merged image shows double positive cells. (b) Transplanted ADSCs are positive for GFP expression (green signals). Scale bar: 100 μm (Image from Published Paper [46])
The use of undifferentiated stem cells from the salivary gland itself has been shown to repair the function of salivary glands damaged by radiation [9, 48–52]. Radiation diminishes the capability of these stem cells to differentiate into the required cell lines, suggesting that the main reason for irreversible hyposalivation is inadequate production of acini cell precursors. Although tissue cultures are required to expand the number of undifferentiated stem cells sufficient to restore salivary function, they showcase a remarkable capability in regeneration with the provision that the required quantity of cells can be harvested. Thus, it is our hope that this innovative method constitutes the basis of treatment for patients presenting with xerostomia in the future.
14.6 Conclusion
Irreversible salivary gland hypofunction occurs from therapeutic radiation exposure to the head and neck area or Sjögren’s syndrome, both of which will lead to irreversible changes in salivary gland acinar cells.
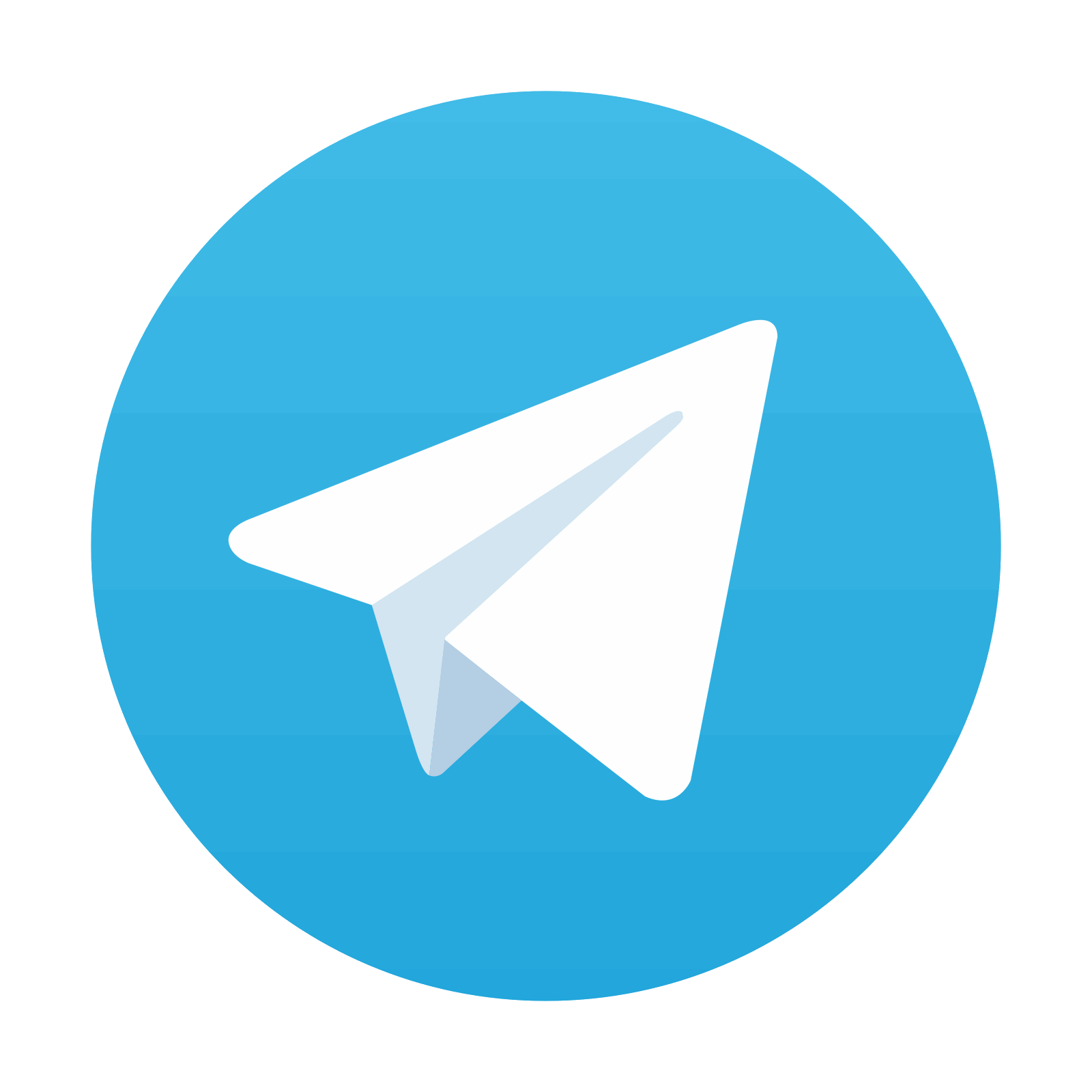
Stay updated, free articles. Join our Telegram channel

Full access? Get Clinical Tree
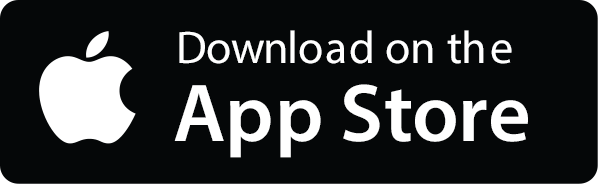
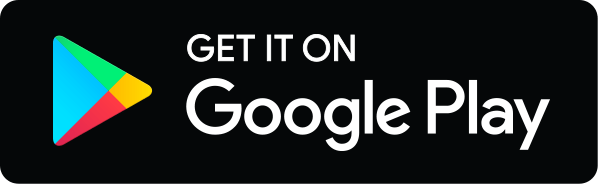