OVERVIEW
The rotational chair test (RCT) is a natural and repeatable multi-frequency stimulation of the vestibuloocular reflex (VOR; Barin, 2009). Specifically, the RCT is a low to mid frequency response of the angular VOR. Similar to caloric irrigations it is limited to horizontal semicircular canal (SCC) and superior vestibular nerve function; however, the RCT evaluates both vestibular systems simultaneously.
There are two main clinical procedures (called VOR tests) performed during RCT:
1. Sinusoidal harmonic acceleration (SHA) examines the VOR response at different frequencies (typically 0.01-0.64 Hz) and peak chair velocities of 50–60°/second . The purpose of the test is comparison of peak slow phase eye velocity (SPV) with head (chair) velocity during sinusoidal chair movements to quantify gain, phase, and symmetry of the response (Figure 6–1). Given the repeatable, stable nature of the chair movements, normative values may be quantified and compared for each of the outcome parameters.
2. Step velocity test quantifies the decay rate of nystagmus following angular acceleration and deceleration. The purpose of this test is to estimate the system time constant and gain of the VOR response.
Figure 6–1. Illustration of a single SHA cycle and calculations for gain, phase, and symmetry parameters. Black solid sine wave represents head-chair movements, and dashed sine wave represents slow phase eye velocity. From Rotational Vestibular Assessment (pp. 165) by Christopher K. Zalewski. Copyright © 2018 Plural Publishing, Inc. All Rights Reserved.
The tests are performed in complete darkness to enhance the VOR response; SPV is extracted from the chair rotation data to quantify the VOR response and the fast phase (reset saccade) is discarded (Figure 6–2). Additional procedures of VOR fixation suppression and visual-vestibular enhancement testing allow input from the visual system. These will be briefly discussed. All tests are considered passive whole-body rotations, in that the patient’s whole body rotates about a vertical axis (Barin, 2009). Modern RCT systems also allow for unilateral centrifugation testing (i.e., on and off axis rotations) to isolate otolith (utricle) dysfunction (see Shepard, Goulson, & McPherson, 2016; Zalewski, 2018 for further discussion).
Review of System Time Constants
During the step velocity test, the rotary chair accelerates to a peak velocity followed by rotation at a constant velocity. When accelerating to the right, for example, endolymph within the right and left horizontal canals will move in a counterclockwise (leftward) direction causing the right cupula to deflect toward the utricle (ampullopetal excitatory response) and the left cupula to deflect away from the utricle (ampullofugal inhibitory response). Vestibular nystagmus is initiated, with SPV movements to the left.
Figure 6–2. Illustration of a single (0.08 Hz) SHA cycle. Top solid black sine wave represents head-chair the nystagmus is extracted to recreate a SPV sine wave (dashed sine wave). The resultant SPV sine wave is then compared to the head-chair sine wave to calculate gain, phase, and symmetry.
The continuation of observed nystagmus beyond the cupular response is due to a central phenomenon within the vestibular nuclei called the velocity storage integrator. The integrator stores electrical signals from the periphery to extend the VOR response; however, the integrator is dependent upon:
1. Intact peripheral vestibular system and vestibular nuclei. If there is dysfunction to these systems the neural integrator will not charge resulting in a shorted time constant.
2. Undamaged connections between the vestibular nuclei and cerebellum. If damaged, the nystagmus response will extend beyond the normal range (prolonged time constant).
CLINICAL CONSIDERATIONS REGARDING RCT
The primary use for RCT is to further quantify the extent of bilateral peripheral vestibular weakness; however, secondary uses may include monitoring central compensation, confirming inconclusive vestibular findings, and further correlating central findings such as failure of fixation suppression (Shepard & Telian, 1996; Ruckenstein & Davis, 2015). Testing is ideal for patients who are unable to complete other portions of the vestibular testing (e.g., caloric irrigations) due to patient factors including:
• outer ear malformation or surgical alteration
• tympanic membrane perforation/PE tubes
• patient age (e.g., pediatric population)
PERFORMING ROTATIONAL CHAIR
Patient Preparation
Rotational chair VOR tests need to be performed in a dark environment, because there is a threat of the visual system reducing the VOR response. Testing may be performed with the use of electrooculography (EOG) electrodes or 2D infrared goggles (video-oculography, VOG). Testing is typically performed in a lightproof booth; however, new systems allow for testing outside of an enclosure via 2D infrared goggles with a cover in place to prevent visual fixation.
The following steps are recommended for optimal patient setup.
1. Remove eye makeup prior to testing.
2. Sit the patient fully upright in the rotational chair enclosure or open chair.
3. If performing EOG, clean electrode sites in order to obtain low skin impedance, and place electrodes on cleaned sites (typically with ground on forehead, and right and left sides of the eyes). If using VOG, place the infrared goggles on the patient and adjust the cameras to focus on the pupils. Ensure that the eye(s) are centered horizontally and the pupil crosshairs and/or threshold are adjusted to adequately track the pupil(s) during testing. Patients will not be able to wear glasses during testing, but may continue to wear contact lenses.
4. The patient’s head should be tilted 30° downward (positioning of horizontal SCCs within plane of rotation) and secured to the chair to reduce the potential for unwanted head movements.
5. Additional security measures should be in place including use of a seatbelt, and shoulder and ankle straps, which are imperative for high velocity testing.
6. The chair may be unlocked once the patient is positioned correctly and secured.
7. Check to make sure that the patient is in total darkness prior to testing.
8. Next, instruct the patient on the calibration procedure that is typically is a saccade task. Calibration will automatically stop when finished. Re-instruct the patient and repeat calibration if necessary. Select default calibration if patient is unable to complete the task
Operator Preparation
The examiner is positioned at a workstation adjacent to the rotational chair. The workstation may include the intercom to communicate with the patient in the lightproof enclosure and computer system to run the equipment and monitor the patient’s eyes during testing. The examiner instructs the patient that their eye movements will be recorded while the chair gently moves. It is important to instruct the patient that most aspects of testing will be performed in the dark; however, they will need to keep their eyes wide open and look forward during the test to accurately record their eye movements. Keep in mind Alexander’s law during testing (i.e., if the patient’s line of gaze is to the right, right-beating nystagmus will be enhanced during testing and this could skew the results). The patient’s line of gaze should be in center gaze position (looking straight ahead).
Additionally, it is necessary to prepare the patient for mental tasking during testing to improve the quality and strength of the VOR response. The patient is given continuous alerting task such as naming items or counting tasks (see Appendix B). Lastly, the patient is informed that breaks will be given as necessary.
TEST PROTOCOLS, ANALYSES AND INTERPRETATIONS
Sinusoidal Harmonic Acceleration (SHA) Test
During SHA, the chair moves in a sinusoidal pattern and the excursion of chair decreases as the test frequency increases. Thus, the time for testing is longer for lower frequencies (e.g., 0.01 Hz) as compared to higher frequencies (0.64 Hz). A time-efficient protocol is suggested for monitoring the VOR response across the low to mid frequency range: (frequencies of 0.08, 0.32 and then 0.01 or 0.02 Hz). If the outcome parameters of gain, phase, and symmetry are normal, further SHA testing is not necessary; however, if any of the outcome parameters are abnormal at any of these frequencies, the following recommendations apply:
• Repeat the frequencies to confirm abnormal findings.
• Check the patient’s level of mental alertness/fatigue.
• Add additional frequencies (0.04, 0.16, 0.64 Hz) to monitor the response across the SHA frequency range.
• Goulson, McPherson, & Shepard (2016) recommend performing 3 cycles at 0.01 and 0.02 Hz, 4 cycles at 0.04 Hz, 8 cycles at 0.08–0.32 and 10 cycles at 0.64 Hz.
Note: It is recommended to perform the low frequencies (i.e., 0.01 and 0.02) last as these are the most symptom-provoking (Goulson et al., 2016).
Gain values denotes average response of rightward and leftward chair movements and provides an indication of the responsiveness of the peripheral vestibular system. Gain is calculated by dividing the maximum SPV by the maximum head (chair) velocity (see Figure 6–1). When eye velocity (SPV) is completely compensatory to head velocity, the gain value is 1.0, which is observed during natural head movement range of 0.5–5 Hz (Leigh & Zee, 2015). However, the VOR response is not as sensitive in the rotational chair frequency range (<0.5 Hz) and thus reduced gain values are observed.
Peripheral vestibular dysfunction further reduces the VOR response, with VOR gain values outside normative ranges (Figure 6–3). However, this interpretation is dependent upon the strength of the SPV. If VOR gain is significantly impaired, phase and gain cannot be calculated. Abnormally low gain values in the presence of normal SHA phase results suggests suppression of the VOR response either due to patient factors (symptomatic during testing, lack of mental alerting, level of fatigue) and/or environmental factors such as visual fixation (Shepard et al., 2016).
Abnormally high gain values may imply central dysfunction (i.e., cerebellar damage), but it is important to clinically correlate these findings with other measures such as oculomotor performance (i.e., saccadic pursuit) or hyperactive caloric irrigations (Shepard et al., 2016). Migraine can also produce high gain values (Zalewski, 2018).
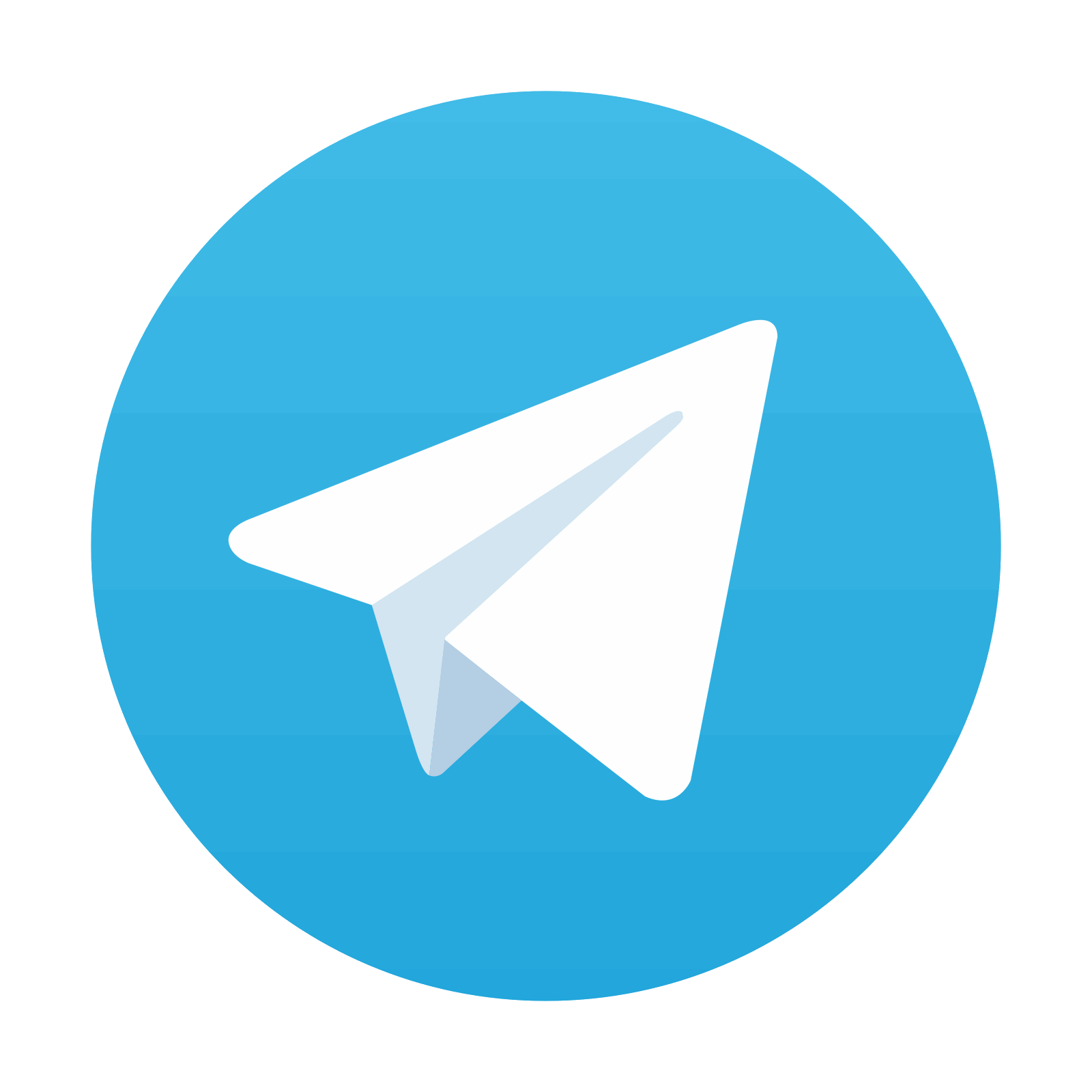
Stay updated, free articles. Join our Telegram channel

Full access? Get Clinical Tree
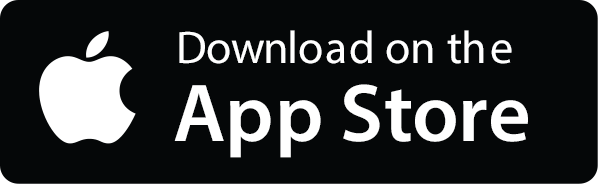
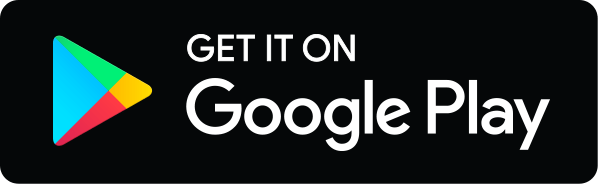