Purpose
To evaluate the in vitro efficacy of rose bengal– and riboflavin-mediated photodynamic therapy for inhibition of methicillin-resistant Staphylococcus aureus (MRSA) isolates.
Design
Experimental study.
Methods
Two different multidrug-resistant, clinical MRSA isolates were grown on nutrient agar, prepared in suspension, and adjusted to concentrations of 1.5 × 10 4 colony-forming units per milliliter. Bacterial suspensions were mixed with rose bengal, riboflavin, or water according to experimental group. Tested in triplicate, groups included: Group I, MRSA control; Group II, MRSA with 0.1% rose bengal; Group III, MRSA with 0.03% rose bengal; and Group IV, MRSA with 0.1% riboflavin. All experimental groups were exposed to 3 lighting conditions: dark, ambient room light for 30 minutes, and 5.4 J/cm 2 with either green light-emitting diode (LED) or ultraviolet-A (UV-A) irradiation. Plates were photographed at 72 hours and custom software measured bacterial growth inhibition.
Results
Complete growth inhibition of both MRSA strains was demonstrated (1) for both rose bengal concentrations under ambient and green LED irradiation, and (2) for the 0.1% rose bengal in the dark. The 0.03% rose bengal in dark conditions showed complete inhibition of strain 2 but incomplete inhibition of strain 1. Riboflavin showed almost complete inhibition with UV-A irradiation but demonstrated minimal inhibition for both strains in dark and ambient light conditions.
Conclusions
Rose bengal– and riboflavin-mediated photodynamic therapy demonstrated complete growth inhibition in vitro of 2 multidrug-resistant MRSA strains. Rose bengal was also effective in dark and ambient conditions. These results may have implications for in vivo therapy.
Introduction
S taphylococcus aureus (S aureus) is among the leading causes of bacterial keratitis. S aureus produces toxic exudates that activate natural host immune responses in the cornea. In an attempt to clear the infection, the host releases proinflammatory cytokines, which results in scarring and functional damage to the cornea. In the most severe cases S aureus keratitis can cause a secondary corneal melt, with a potential corneal perforation that can lead to endophthalmitis.
Traditionally, clinical treatment of bacterial keratitis has been empirical, with a recent shift toward culture-guided practice. A patient with presumed infectious keratitis will likely receive monotherapy with a fourth-generation fluoroquinolone as a first-line treatment. Repeated and inappropriate use of antibiotics has led to the rise in drug-resistant strains of bacteria such as methicillin-resistant Staphylococcus aureus (MRSA). Increased resistance of MRSA to current fluoroquinolones and other common ocular antibiotics has led to limited and less than optimal treatment options.
One promising alternative treatment is photodynamic therapy (PDT), which has been explored to treat a variety of diseases. Photodynamic therapy involves the activation of a photosensitizing agent with light at a specific wavelength band. When the photosensitizer is activated to an excited state, it interacts with ambient oxygen to produce either type I or type II reactions, which can lead to cell death by activating apoptotic pathways, along with many other interactions.
In ophthalmology, PDT has been widely applied for the treatment of choroidal neovascularization in patients and has been tested for its effects on corneal neovascularization. Currently, the most common application of PDT in the cornea is for corneal collagen cross-linking (CXL) to treat keratoconus using riboflavin as a photosensitizing agent and ultraviolet-A (UV-A) irradiation, first established under the Dresden protocol (0.1% riboflavin solution and UV-A irradiation for a total of 5.4 J/cm 2 ). Studies have shown the value of using riboflavin CXL following the Dresden protocol as an adjunct therapy for infectious keratitis.
The efficacy of rose bengal, a commonly used ocular stain, as an alternate photosensitizer has been investigated for inhibiting the growth of microbial isolates. One group found the minimum concentration of rose bengal needed to inhibit fungal isolates to be 280 μM (0.03%), which was used as one of the concentrations of rose bengal in our study. It was hypothesized that PDT is effective in eradicating multidrug-resistant MRSA strains in vitro. To test this, 2 clinical MRSA strains were exposed to 0.03% and 0.1% rose bengal–mediated PDT and 0.1% riboflavin-mediated PDT and observed for growth inhibition.
Methods
All work for this experimental study was performed at the Ophthalmic Biophysics Center and Ocular Microbiology Laboratory, Bascom Palmer Eye Institute, University of Miami Miller School of Medicine, Miami, Florida, USA.
Figure 1 provides a general overview of methods and workflow of the in vitro PDT experiments.
Bacterial Strain and Growth (Inoculum)
Inocula were prepared from 2 different multidrug-resistant MRSA strains that were isolated from corneas of patients with confirmed infectious keratitis.
The parent cultures of both strains came from patients with similar clinical scenarios. Both patients had chronic exposure keratitis that was not well treated and required previous prolonged hospital admission, during which they were treated with a variety of ocular fortified and nonfortified drops. There was no or minimal response using fluoroquinolones. Both patients presented to Bascom Palmer Emergency Room with corneal perforation secondary to infectious keratitis (MRSA). Patients were managed with therapeutic graft surgery and fortified vancomycin.
MRSA strain 1 (MRSA-1) was resistant to 4 classes of antibiotics (×4): fluoroquinolones (ciprofloxacin, levofloxacin, moxifloxacin), macrolides (erythromycin), beta-lactams (penicillin, oxacillin), and lincosamides (clindamycin). MRSA strain 2 (MRSA-2) was resistant to 6 classes of antibiotics (×6): fluoroquinolones (ciprofloxacin, levofloxacin, moxifloxacin), aminoglycosides (gentamicin), macrolides (erythromycin), sulfonamides (trimethoprim sulfate), tetracyclines (tetracycline, doxycycline), and beta lactams (penicillin, oxacillin). In vitro susceptibilities were based on systemic minimum inhibitory concentration breakpoints.
Parent cultures of each strain were plated on nutrient agar (W31; Hardy Diagnostics, Santa Maria, California, USA) and placed in an incubator (Precision Scientific; Scientific Products, Miami, Florida, USA) at 30 C for 18–24 hours. On the day of experimentation, MRSA strains were transferred to tryptic soy broth (R064890; Remel Products, Lenexa, Kansas, USA) and concentrations standardized to 1.5 × 10 8 colony-forming units (CFU/mL) using a spectrophotometer (Novaspec Plus 80-2117-50; Biochrom LTD, Cambridge, England) and serially diluted to the final working concentration of 1.5 × 10 5 CFU/mL.
Photosensitizer Preparation
Solutions were prepared on the day of experimentation and kept in light-protected test tubes to prevent photobleaching of the photosensitizers. The 0.1% and 0.03% rose bengal solutions were prepared by dissolving 100 mg and 30 mg of rose bengal (198250; Sigma-Aldrich, St Louis, Missouri, USA), respectively, in 100 mL of high-purity water (NERL 9805; Fisher Diagnostics, Middletown, Virginia, USA). The 0.1% riboflavin solution was made by dissolving 100 mg of riboflavin-5-phosphate (R7774; Sigma-Aldrich) in 100 mL of high-purity water.
The MRSA-1 and MRSA-2 suspensions were mixed with the appropriate photosensitizer solution or high-purity water for a final bacterial concentration of 1.5 × 10 4 CFU/mL. Under minimal light conditions, corresponding to switching off the room light with residual 2 lux or 0.20 μW/cm 2 across the visible light spectrum, 1 mL aliquots of the MRSA suspension were pipetted onto nutrient agar plates (15 × 100 mm Nutrient Agar plate W31; Hardy Diagnostics, Santa Maria, California, USA), for a total preparation time of approximately 10 minutes.
Experimental groups were conducted in triplicate and the plates were divided into 4 groups for each strain of bacteria: (1) MRSA-only control, (2) MRSA with 0.1% rose bengal, (3) MRSA with 0.03% rose bengal, and (4) MRSA with 0.1% riboflavin. Each group was exposed to either (1) dark or (2) ambient light or (3) irradiation of either green light-emitting diode (LED) for rose bengal or UV-A for riboflavin.
The ambient light group was exposed for 30 minutes, the green LED irradiation was performed for 34 minutes, and the UV-A irradiation was performed for 30 minutes. After irradiation, all plates were immediately placed inside a lightproof container to prevent further light activation and stored in an incubator at 30 C.
Irradiation Sources
Two irradiation sources were built in-house using an array of LEDs ( Figure 2 ). The green LED irradiation source, used to activate rose bengal, contains LEDs in an array of diameter 47 mm. The green irradiation source had a peak wavelength of 518 nm (I 40% : 500–541 nm) (L1-0-G5TH45-1; LEDSupply, Randolph, Vermont, USA) and produced an average irradiance of 2.8 mW/cm 2 . The UV-A irradiation source, used to activate riboflavin, contains LEDs in an array of diameter 37 mm. The UV-A irradiation source had a peak wavelength of 375 nm (I 40% : 370–383 nm) (L7-0-U5TH15-1; LEDSupply) and produced an average irradiance of 3.0 mW/cm 2 .
The ambient light condition was produced by the laboratory fluorescent illumination of 300 lux and produced an irradiance of 107 μW/cm 2 across the visible light spectrum. Irradiances were measured at the surface of the agar plates using a photo power meter (Model 1916C; Newport, Irvine, California, USA). Spectra for each of the 4 light sources were recorded using a spectrometer (SM442; Spectral Products, Putnam, Connecticut, USA).
Percent Inhibition Measurements
Agar plates were placed on a custom-built retroilluminator and photographed every 24 hours with a digital camera (16.2 MP, Nikon D7000; Nikon Inc, USA) with resolution of 31.7 px/mm. Percent growth was measured with a custom-made software written in LabVIEW 6 (National Instruments, Austin, Texas, USA). For every plate, a central zone was selected for measurement corresponding to the irradiation source diameter (47 mm for green irradiator and 37 mm for UV-A irradiator).
The images were converted to grayscale and background illumination was normalized, image contrast was increased, and images were segmented by applying a series of binary threshold filters.
The resulting black-and-white image had white pixels for bacterial growth and black pixels for no growth. The area of growth was calculated by summing the total number of white pixels. Percent growth was determined by dividing total growth by the total pixel area of the irradiation zone. Percent growth values were validated by comparing to manual segmentation methods and were found to be within the same range of precision.
The control group was the dark condition inoculated with MRSA and corresponded to 100% growth. All other experimental groups were compared to this value. To calculate percent inhibition, percent growth was subtracted from 100%, as the control group has 0% inhibition.
Statistical tests were performed to assess and compare the percent inhibition between each experimental group. Statistical significance was set at P < .05.
Results
Seventy-two hours after irradiation, images were taken and percent inhibition was calculated. Results are displayed in Figure 3 .
The 0.1% and 0.03% rose bengal showed 100% inhibition of both MRSA strains in both ambient light and green LED irradiation groups. In dark conditions, 0.1% rose bengal demonstrated 100% inhibition in both strains, while 0.03% rose bengal showed 94% inhibition in MRSA-1 and 100% inhibition in MRSA-2. Photographs taken 72 hours after MRSA growth with rose bengal and exposure to either dark, ambient, or green LED irradiation are displayed in Figure 4 .
Riboflavin with UV-A irradiation showed inhibition in both MRSA strains: 98% in MRSA-1 and 100% in MRSA-2. Riboflavin with ambient light showed 13% inhibition in MRSA-1 and 15% inhibition in MRSA-2. Riboflavin in dark conditions showed 11% inhibition in MRSA-1 and 3% inhibition in MRSA-2. Photographs taken 72 hours after MRSA growth with riboflavin and exposure to either dark, ambient, or UV-A irradiation are displayed in Figure 5 .
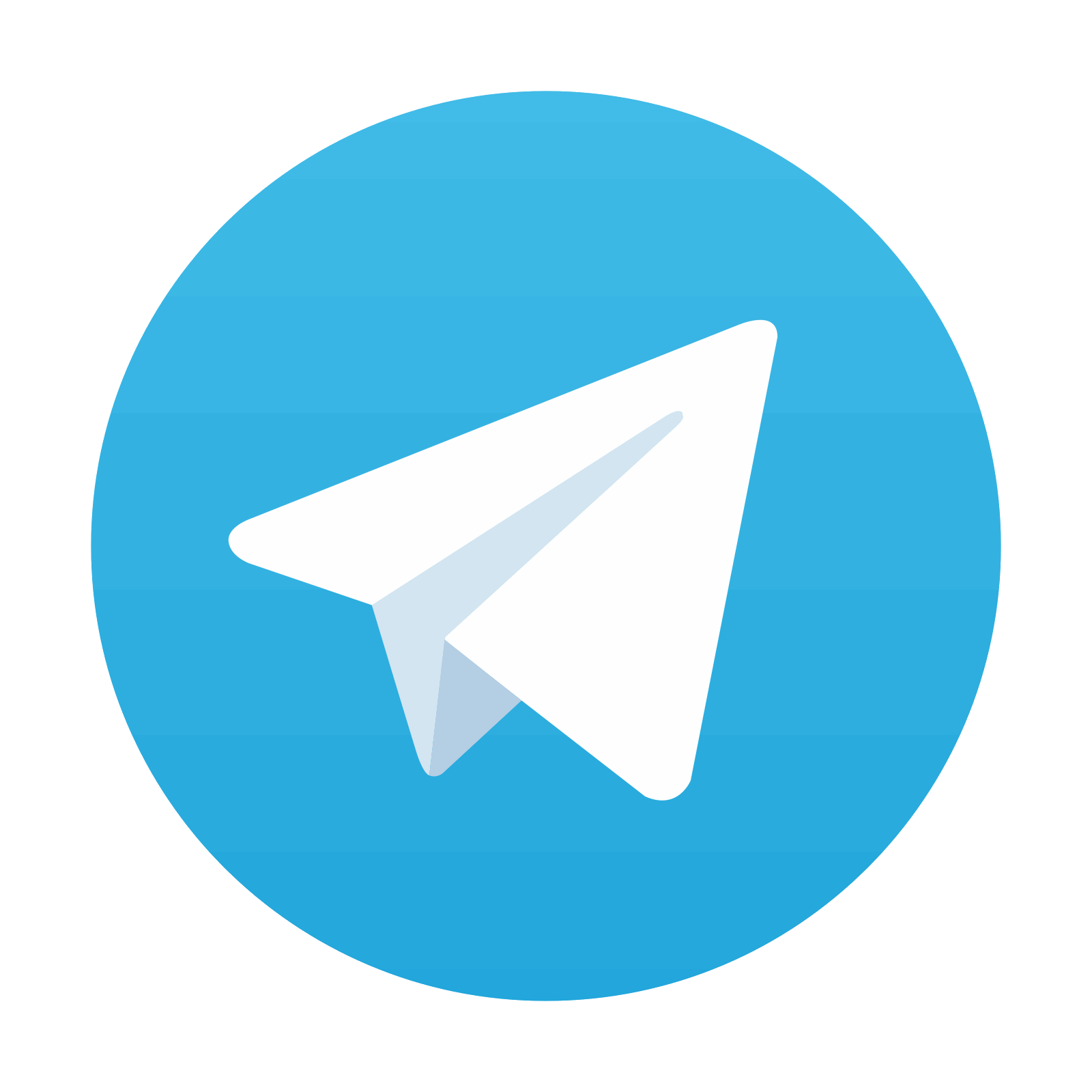
Stay updated, free articles. Join our Telegram channel

Full access? Get Clinical Tree
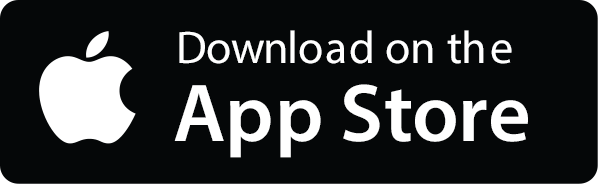
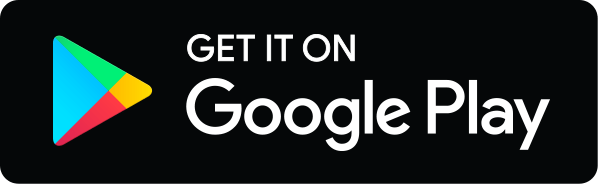
