41 Robotics, Image Guidance, and Computer-Assisted Surgery in Otology/Neurotology
What is the true definition of a surgical robotic system? Although the definition is constantly evolving, Maja Mataric defines a robot as “… an autonomous system which exists in the physical world, can sense its environment, and can act on it to achieve some goals.” None of what is commercially available truly meets this strict definition of a robotic system. Da Vinci (Intuitive Surgical Inc., Sunnyvale, California, United States), the most popular and widely used “robotic” system, is more accurately described as an active arm or manipulator working in a master–slave set-up; it is not functioning autonomously.1 Although the term robotics has been most commonly used, in a broader perspective, computer-assisted surgery (CAS) may be a better term. CAS incorporates wide-ranging technologies such as smart tools, portable devices (e.g., smartphones or tablet computers), or even the use of “smart” beds and fabric that can record heart rate, respiratory rate, and temperature. Ideally, these revolutionary technologies will make even complex and lengthy surgeries safer by reducing the rate of perioperative complications.
Robotic systems and CAS are continuing to grow in importance in health care, especially when considering the combined civilian, military, and space sectors. They can perform telemedicine functions within a hospital or remote locations, as well as virtual surgical assistants or scrub technicians. The medical robotics market will continue to grow in importance due to an aging population and the looming doctor and nursing shortage. In addition, due to a reduced tolerance to errors and complications by insurance companies, the federal government (e.g., Medicare), and patients alike, there is a potential for an improved quality of care with the use of CAS. A recent study suggests that the surgical robot device markets are anticipated to reach $8.5 billion by 2018.2
One of the earliest functions of robotics was to perform as assisting devices, such as holding a laparoscope or endoscope.1 One of the earliest surgical robotics projects involved a transurethral resection of the prostate (TURP) with Probot, which resected benign prostatic hypertrophy (BPH) via ultrasound guidance.3 The reported benefits of robotic systems include tremor and fatigue reduction through the use of an ergonomic robotic arm that is more precise, predictable, reproducible, and accurate than the human arm.3–5 It can potentially minimize trauma to the patient and reducing subsequent morbidity and mortality.6 There is much that the field of otology/neurotology may learn from other disciplines’ use of robotics, including cardiac surgery, gynecologic surgery, bariatric surgery, neurosurgery, and urology. If otology/neurotology has a viable robotic system created, there is a possibility for a paradigm shift to occur. When Mouret first reported laparoscopic cholecystectomy in 1987, which made open cholecystectomy a much less desirable option for patients it also arguably shifted general surgery’s main focus from the traditional open surgery to a minimally invasive approach.7
A slow evolution in robotics and CAS will continue to occur, particularly as improvements in hardware and software occur. These improvements will occur in image guidance, ergonomics, virtual reality/simulation, the addition of haptic feedback, and miniaturization.3 This chapter will focus on the most relevant robotic systems currently available.
Use of Robotics Systems in Urology, Orthopedics, and Neurosurgery
Approximately 1000 da Vinci systems are currently in the world with approximately 80% of them located in the United States8 (Fig. 41.1). The system has a patented EndoWrist technology with six degrees of freedom, making it easy and comfortable to use. The biggest driver of da Vinci use has been in urologic oncology. Currently, in the United States, the majority of prostatectomies for prostate cancer are done robotically using the da Vinci. This procedure is also known as robot-assisted laparoscopic prostatectomy (RALP). RALP was first performed in the United States in 2000, the same year the U.S. Food and Drug Administration (FDA) approved the device.8,9 Before the inclusion of the robot in this procedure, urologic surgeons found it technically challenging to perform this procedure laparoscopically. The da Vinci’s exquisite three-dimensional (3D) magnified vision and tremor reduction with ergonomic instruments/arms have allowed this procedure to develop.3,8 The growth in RALP has occurred worldwide, including in Europe and Asia, although those regions are about a decade behind the United States in use. The results have been reproducible across surgeons and continents, due to the quality of mentoring and ability to teach through video.10 However, there is a reported learning curve of 100 to 200 cases, particularly with locally advanced disease, obese patients, a history of intra-abdominal surgery, or other high-risk cases.10 Single-institution series of RALPs exceeding 1000 cases are now available.8 Some of this increase is marketing related but has been supported by data in peer-reviewed publications.3 The da Vinci has clearly become, at this point in time, the most successful soft-tissue surgical robotic system. Its improvement has allowed it to be used in otolaryngology, including the head and neck (e.g., for thyroidectomies) as well as for transoral robotic surgeries (TORS).
For neurosurgery, the Pathfinder was developed as an image-guided robotic system that has been used for placement of biopsy needles, deep brain stimulator electrodes, and drug-delivery catheters.6 The system uses a preoperative computed tomography (CT) scan with fiduciary markers on the head. After the scanning is complete, a 3D model of the head is reconstructed. The surgeon then uses the workstation to assess the coordinates of the target zone that needs treatment or intervention and then plans entry points and routes accordingly.6 The Pathfinder robot is then placed next to the patient with photo-reflective fiduciary markers replacing the ones used by the CT scan. The head is rigidly fixed to the base of the Pathfinder system. The surgeon performs the appropriately sized craniotomy. The Pathfinder uses its mounted digital camera to locate the patient’s head. Images are taken with both ambient lighting as well as with an arm-mounted light-emitting diode (LED) ring, allowing for subtraction methods that leave the photo-reflective fiducials in place. The fiduciary markers are translated from their 3D locations to robotic coordinates. The goal is to achieve submillimeter accuracy, but errors in positioning affect overall accuracy. This can be due to patient anatomic changes, as well as errors due to geometric and nongeometric factors (thermal effects, flexibility of links, and tool length). Other neurosurgical robotic systems used, primarily for research, include the NeuroMate (Rainshaw, County of Gloucestershire, United Kingdom) and NeuroMaster (Robotics Institute of Beihang University, Beijing, China) systems.
A German research team has used robot-assistance for craniotomies and cranioplasty in the surgical treatment of craniosynostosis.11 The group performed the procedures on animal models using a force–torque sensor, pneumatic overload-protection system, infrared instrument tracking, and a real-time operating system. To prevent inadvertent injury, the team used redundant safety systems as well as emergency stop buttons. Before CT scanning, the head was immobilized, and four titanium screws were placed for registration and acted as fiduciary markers. Autonomous drilling was then done by the robotic system based on the above information.
Klein et al used image guidance for placement of craniofacial/auricular implants; the titanium implants were 3.75 mm in diameter with a length of 3 to 4 mm.12 The robot used was an improvement of the SurgiScope robot (Elekta IGS, Grenoble, France); this original robotic system was to position microscopes and was held from the ceiling. The goal was to achieve better functional and aesthetic outcomes. Surgeon training was performed using both artificial models and cadavers. The team performed robot-assisted surgery on 13 patients (age range, 14 to 49 years) using rapid prototyping as well as preoperative computed tomography scans. Since active robotic systems require fixation of the patient’s head and stability, the fixation system involved a custom-made polyurethane headrest and maxillomandibular splint.12 Using the robotic system, absolute implant position accuracy was 0.5 ± 0.4 mm. The fixation system used was the same for the imaging and the procedure. The rigid fixation of the teeth fixed the patient’s head to the operating room (OR) table. A reference frame for the navigation system was also attached to the dental splint. The robot controller received the registration information from the planning tool. The CT image data was used to create a mirror replica of the normal, healthy contralateral ear. The system used infrared navigation and computers for both the navigation and the manipulator. The robot did not work autonomously but interactively with the surgeon. The robot held the drill hand-piece; movement occurred when the surgeon moved the arm; however, movements are restricted with the robot limiting movement along the proposed drilling axis to achieve preoperatively planned implant position. In 10 patients, no intraoperative complications occurred. Robot preparation and set-up took approximately 30 minutes. The procedure required an additional 25 minutes over a standard, conventional operation.
Figure 41.1 The da Vinci surgical system from Intuitive Surgical, Inc.
©2012 Intuitive Surgical Inc., Sunnyvale, California, United States. Printed with permission.
In the orthopedic realm, Cartiaux et al used a bone-substitute model to assess cutting accuracy by comparing free-hand cutting, navigation assisted free-hand cutting, and robotic-assisted technologies with an oscillating saw.13 The authors examined three variables: cut height and two angles. Not surprisingly, the six-axis robot-assisted technique did the best.
Use of Robotics and Computer-Assisted Surgery in Otology/Neurotology
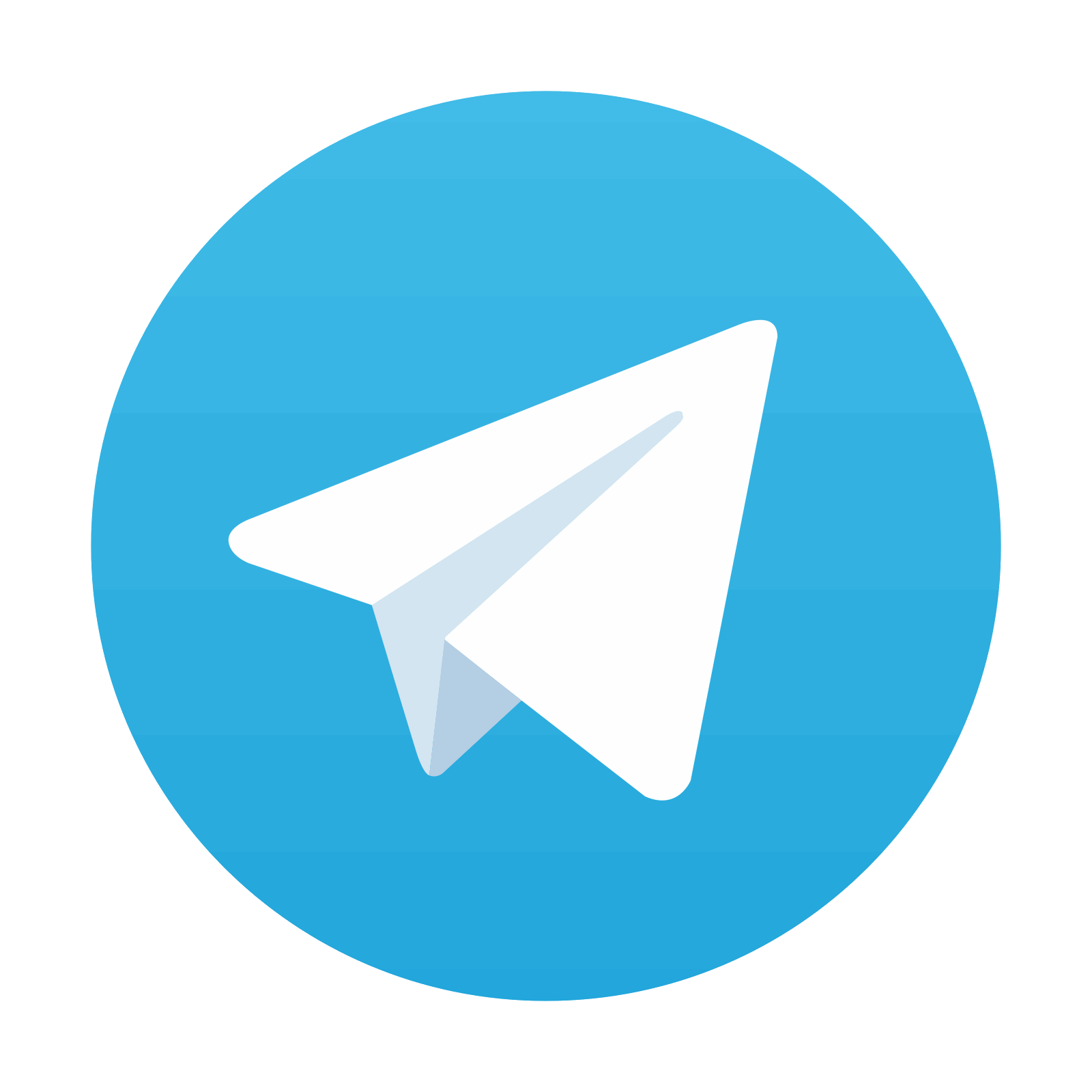
Stay updated, free articles. Join our Telegram channel

Full access? Get Clinical Tree
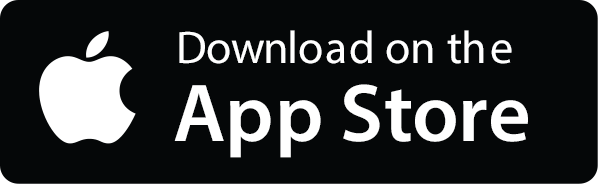
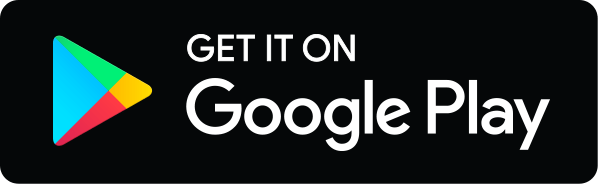