Fig. 14.1
Da Vinci Surgical System showing surgeon looking into console viewer, robot with arms separated physically from surgeon’s console (Image courtesy of Intuitive Surgical)
Robotic Eye Surgery History
Robotic eye surgery has a relatively short history compared to robotic surgery in general. Whereas other surgical fields have accumulated a significant clinical experience using robotic surgery on patients, the focus of Ophthalmic surgeons has been on demonstrating the feasibility of specific ocular procedures using a robot in a laboratory setting. There are several reasons for this cautious approach to robotic acceptance in the field of Ophthalmology. Ocular surgery already employs minimally invasive techniques providing rapid recovery and excellent outcomes. Eye surgeons have good control and excellent views with the current standard of optical microscopes. Lastly, maneuverability of ophthalmic instruments poses little problem for experienced surgeons with trained hands.
Robotic surgery has addressed the limitations of traditional surgery in other surgical disciplines. Benefits of this system, compared to traditional surgery have demonstrated increased precision, improved range of motion, tremor elimination, increased surgeon safety, and ability to maneuver in confined anatomic spaces [17–21]. These are all central facets to advancement in any surgical field and by extension to ocular surgery. In other words, there is always room for improvement for surgeon control, safety, and patient outcomes in any surgical field.
In 1989, Guerrouad and Vidal described and developed the first robot dedicated to eye surgery, the Stereotaxical Microtelemanipulator (SMOS). The SMOS included a spherical micromanipulator that provided for 6 degrees of freedom. It demonstrated improved accuracy of specific tasks compared to manual surgery, but was slower [22].
The Robot Assisted MicroSurgery (RAMS) workstation developed by Steve Charles and collaborators with NASA’s Jet Propulsion Laboratory was first described in 1997 [23]. The RAMS workstation is a compact robot that allowed for extensive precision of movement down to 15 microns along with motion scaling, that is where large movements in the surgeon’s hand are translated to micro-movements by the robot.
At the same time, Northwestern University developed a robot that allowed for retinal vessel cannulation inside a cat’s eye. The retinal vessels ranged in internal diameter from 20 to 130 microns [24].
In 1998, Yu et al. developed a patented spherical manipulator, similar to the SMOS, specifically to demonstrate feasibility off intra-retinal vascular drug delivery, implantation of ocular microdrainage devices and the intraretinal manipulation of microelectrodes. These tasks were successfully carried out with minimal tissue damage in a laboratory setting [25].
Japanese collaborators created a prototype robotic system based on the SMOS platform that was designed to aid in multiple specific tasks of vitreoretinal surgery [26]. This robot facilitated successful surgical induction of a posterior vitreous detachment, retinal vessel sheathotomy using 25-gauge microscissors, and microcannulation of retinal vessels with a diameter of 100 microns in porcine eyes.
Investigators at Johns Hopkins University developed a steady hand manipulator (SHM) for retinal microsurgery [27]. The design places the pivot point of the articulating probe at the sclera (as it would be in traditional vitreoretinal surgery), Placing the remote center of motion (RCM) at this location minimizes undesirable tension on the eye wall. The SHM provided filtration of tremor that was demonstrated experimentally. Further innovations by this group include intra-operative retina registration that syncs with pre-operative imaging to guide treatment delivery to a specific point on the retina [28]. The Micron, a microsurgical tool that reduces unintentional tremor while preserving eye-hand coordination was also developed and tested. Surgeons experienced up to 52 % reduction in error in validation experiments using the Micron [29].
In 2006 the feasibility of robotic ocular surgery and robotic ocular telesurgery using the Da Vinci Surgical System was demonstrated through a series of studies [30, 31]. The experiments were performed on harvested porcine eyes placed in an anatomical position using a foam head on a standard operating room table. Visualization of the eye was achieved with a 3D endoscope camera directly above the globe, thus mimicking a view through a standard operating microscope. The 2 robotic arms were placed on either side of the globe at a 45-degree angle, resembling the same approach used by a surgeon to maximize exposure to the ocular surface. The surgeons performed the procedures while positioned at the surgeon control area that was located across the operating room suite. External ocular surgery (cornea suture laceration repair) was performed and deemed feasible (Fig. 14.2). Anterior segment procedures (clear cornea incision creation and anterior capsule capsulorhexis formation) were performed and felt not to be practical given the impossibility to position the remote center of motion at the fulcrum, which caused undue tissue strain. Posterior segment procedures (core vitrectomy and trocar insertion / removal) proved possible, although limitations were noted secondary visualization (difficult view of posterior chamber) and robotic arm issues that did not allow for refined movements within the vitreous cavity.


Fig. 14.2
Surgeon’s view through the Da Vinci Surgical System showing suture closing of a corneal laceration. A black diamond robotic micro-forcep (controlled by robotic arm) is shown holding one end of the suture. The other robotic micro-forcep is out of the picture frame holding the other end of the suture (Image courtesy of A Tsirbas)
We have learned over these past several decades that robotics lends itself to ocular surgery in unique ways compared with other surgical disciplines. Tactile feedback mechanisms are advantageous for other surgical fields but are not a necessity for eye surgery. Ocular surgeons rely primarily on visualization as their main tool for performing delicate intraocular tasks. Tremor elimination and motion scaling mechanisms are very important in a microsurgical field involving the eye [21, 32]. Within the confines of the orbit and eyeball, each movement needs to be precise and tremor free. Tremor reduction and motion scaling components of some of these robotic systems has demonstrated this advantage be employed in eye surgery.
Robotic Eye Surgery Today (Video 14.1)
Recent advancements have been made to overcome some of the cumbersome limitations of using the Da Vinci Surgical System.
In order to allow for a more natural vitrector motion by placing the remote center of motion at the fulcrum, a smaller micro robot, the Hexapod Surgical System (HSS) was created [33]. The HSS is mounted to an arm of the Da Vinci Surgical System. The precision and dexterity of this approach was validated by successful insertion of a vitreous cutter through a sclerotomy in porcine eyes.
A robotic forceps advancement, the “Micro hand,” was developed to allow for a membrane peeling function necessary in many types of vitreoretinal surgeries [34]. This device was designed to mimic a human hand and is pneumatically controlled, allowing titration of grasping force. Four fingers, with a length of 4 mm each, were used to manipulate fresh retinal tissue of porcine cadaver eyes.
Steerable micro-robots with an outer diameter of less than 500 μm were created and injected through a 23 g needle into the vitreous cavity of cadaver porcine eyes. Using a wireless electromagnetic control headset, researchers were able to achieve coordinated movement of the robots and targeted placement along the retina surface [35]. These micro-robots may act as a future drug delivery system that can be moved to a specific place in the eye (once injected into the eye) using robot steering technology.
Retinal vascular cannulation robots that allow for telemanipulation have been developed [36, 37]. Preliminary feasibility testing shows that this outperforms standard vascular cannulation. An integrated robotic intraocular snake, a submillimeter intraocular dexterous robot prototype has been built [38]. It can eventually be utilized as a steerable needle, steerable forceps, or steerable cannula.
The Intraocular Robotic Interventional Surgical System (IRISS) is a dedicated microsurgical platform capable of performing complete ophthalmic procedures [39]. The design features a remote console which could facilitate telesurgery. The IRISS design includes a head-mounted stereoscopic visualization system, two joystick controls with tremor filtration and scaled motion, custom designed arms appropriately sized to accommodate commercially available instrumentation, and two closely approximated remote centers of motion (pivot points) to reduce tissue stress at the point of ocular entry (Figs. 14.3 and 14.4). Recent trials have focused on three complex ocular procedures: lens capsulorhexis in cataract surgery, 23-gauge core vitrectomy, and retinal vein cannulation. The team is currently developing automated capabilities of the IRISS platform for cataract surgery.



Fig. 14.3
Intraocular Robotic Interventional Surgical System (IRISS) schematic view: custom designed arms appropriately sized to accommodate commercially available instrumentation, and two closely approximated remote centers of motion (pivot points) to reduce tissue stress at the point of ocular entry (Image courtesy of JP Hubschman)

Fig. 14.4
Intraocular Robotic Interventional Surgical System (IRISS) working prototype (Image courtesy of JP Hubschman)
The PRECEYES Surgical System is a prototype robotic system that is being developed primarily for vitreoretinal surgery [40]. It is mounted at the side of the head during vitrectomy surgery and can be brought into place and used as needed (Fig. 14.5). It functions to scale down movements for better precision, filters out tremors, and allows the instrument to freeze in place if the surgeon’s hand relaxes. This robotic system is going to be tested for its ability to deliver gene therapy into the subretinal space for a variety of inherited disorders [41].


Fig. 14.5
PRECEYES Surgical System prototype showing how it is mounted on the side of the patient’s head rest (Image courtesy of PRECEYES)
In 2015, the first human case of robot assisted eye surgery, a pterygium removal, was performed on a 73-year-old patient using the DaVinci robotic surgical system [42].
Today we realize ocular surgery is a field that continues to benefit from incremental advances in robotic technology. Improved instrument engineering, precision scaled movements, integrated tremor reduction, and improved maneuverability are all key advancements that robotic integration currently provides.
Robotic Eye Surgery of the Future
The feasibility of telesurgery was demonstrated in 2001 when Marescaux and colleagues performed the first transatlantic robotically assisted surgery on an animal model [43]. This was followed by the first transatlantic robotically assisted laparoscopic cholecystectomy in a human being [44]. Since then, telesurgery has been demonstrated successfully on multiple occasions [45]. Ocular robotic telesurgery may also be feasible, bringing emergency eye care to distant and hard-to-reach locales, such as an isolated island or war torn nation.
In 2016, the Smart Tissue Autonomous Robot (STAR) demonstrated for the first time the ability of a fully autonomous robot to suture up intestine on a live pig [46]. Autonomy would be a logical next step in robotic eye surgery. Performing routine and replicative tasks such as trocar insertions, infusion connection, and trocar removal could save the surgeon valuable time and energy on each case. Future advancements in sensor technology that prevents the vitreous cutter from hitting the retina would allow for a core vitrectomy to be an automated task. Perhaps 1 day, even the most delicate vitreoretinal surgical tasks such as internal limiting membrane peeling will be an automated experience.
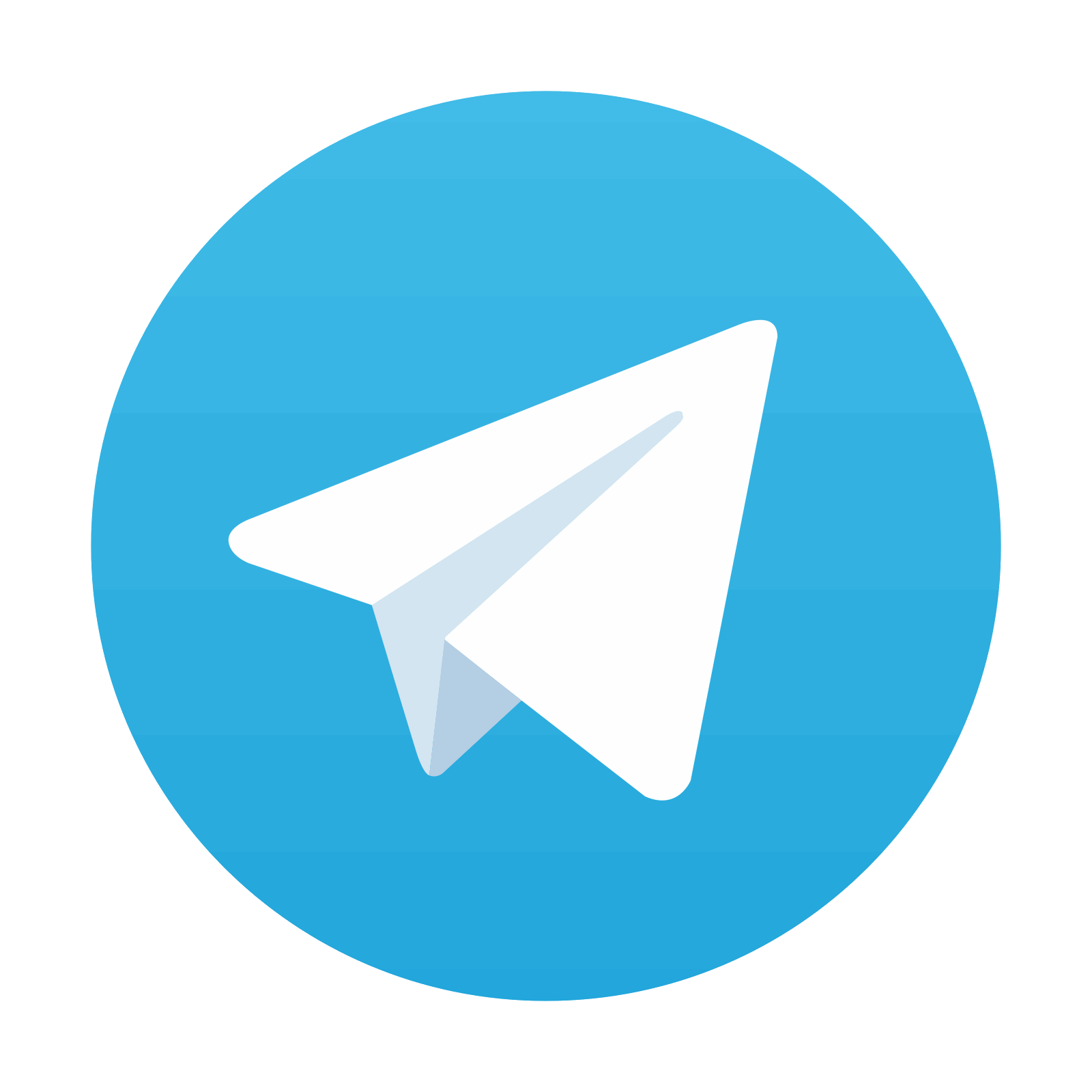
Stay updated, free articles. Join our Telegram channel

Full access? Get Clinical Tree
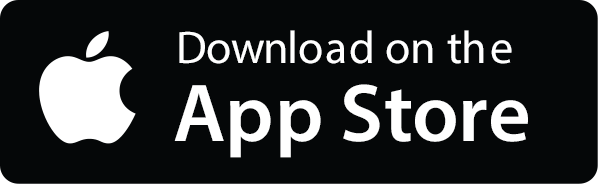
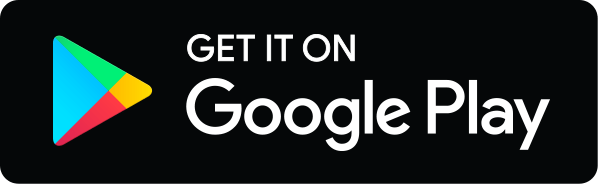