Fig. 27.1
Non-penetrating or closed-globe injuries may occur by several mechanisms thus damaging a variety of different retinal structures including the iris (1), trabecular mash work (2), lens fibers (3), retina (4), posterior pole (5), or optic nerve with the posterior ciliary vessels (6)
The following chapter demonstrates a variety of closed-globe injuries on time and spectral domain optical coherent tomography (OCT), fluorescein angiography (FA), autofluorescence (AF), and fundus photography.
27.2 Choroidal Folds
Choroidal folds were first described by Nettleship in 1884 and were initially considered as foldings of the entire retina (Nettleship 1884). They are visible striae at the posterior pole that radiate across the macula arranged in a parallel and horizontal fashion as dark and light lines. Later Norton investigated the typical fluorescein angiographic (FA) appearance and defined a clear distinction between choroidal and retinal folds (Norton 1969). The anatomical structure of choroidal folds can be explained by the typical fluorescein pattern traversing the folded choroidal vessels. Hyperfluorescent streaks occur in the early arteriovenous phase and persist throughout the late venous phase with no leakage. A stretching and atrophy of the RPE induce an increased filling of the choriocapillaris overlying this peak of the choroidal fold. Hypofluorescent streaks are on the bases of inclinated RPE in the valley of the fold, resulting to a compression of the pigment-containing base of the RPE cells and blockage of the underlying choroidal fluorescence. Histological studies confirmed a folding at the level of the RPE, Bruch’s membrane, and choroid giving the retina above a “brain-like” configuration. Cross-sectional imaging by computer tomography (CT) or B-scan ultrasonography reveals an abnormal flattening of the posterior pole, a thickening of the sclera, and a consecutive axial shortening of the globe (Dailey et al. 1986). This characteristic picture is associated with a variety of orbital and ocular conditions including trauma, hypotony, orbital tumors, thyroid disease, papillitis, or uveal effusion syndrome.
Color fundus picture (Fig. 27.2a) and fluorescein traversing the folded choroidal vessels demonstrated hyperfluorescent streaks in the early and late phase with no signs of leakage, while adjacent hypofluorescent streaks remained dark throughout the entire angiography. In vivo measurements by OCT determined a significant folding of the hyperreflective RPE-choriocapillaris complex (Fig. 27.2b) in the papillomacular area. The thickness, reflectivity, and surface contour of the retina appeared within normal limits.


Fig. 27.2
Choroidal folds. (a) In the late venous phase of the fluorescein angiography, there are multiple parallel and horizontal lines of different fluorescence in the macular area. The hyperfluorescent streaks persist with no leakage and are caused by an increased filling of the choriocapillaris overlying the peak of the choroidal fold. The hypofluorescent streaks are in the valley of the fold, where the compressed RPE blocks the underlying choroidal fluorescence. (b) The vertical OCT scan of the choroidal folds in the papillomacular area. The inner surface of the retina is flattened by image analysis using Adobe Photoshop, so that the choroidal folds become more prominent. The neuroretina in the papillomacular bundle has a moderate reflectivity and normal thickness of approximately 225 μm. There is no intraretinal or subretinal fluid. The folded hyperreflective band, in red to white colors, corresponds to the RPE-choriocapillaris complex and is firmly attached to the underlying sclera, with no signs of pigment epithelium detachment (PED). Each fold has a height of approximately 45 μm. The dark and hyporeflective spots in the deep scleral layer under each fold (white arrows) may either correspond to compressed tissue or shadowing of the folded RPE-choriocapillaris complex
Differently patients with ocular hypotony may face severe impaired vision with metamorphopsia. On fundus examination there is a significant tortuous beading of the retinal and venous vessels (Fig. 27.3a). Fluorescein angiography (FA) demonstrates a fussy fluorescence in the early and late phase (Fig. 27.3b). OCT shows a mountain-like folding of the entire retina with moderate hyporeflective areas in the subretinal space corresponding with subretinal fluid (Fig. 27.3c). Between the RPE and the sclera, there are flat large areas corresponding with choroidal fluid.


Fig. 27.3
(a) Fundus image of the right eye in patients with severe hypotony after blunt ocular trauma. Funduscopy demonstrates a chorioretinal folding of the entire retina (green arrow). (b) Fluorescein angiography demonstrates a moderate elevation of the retina with mild leakage. (c) On OCT there is a significant folding of the entire neuroretina (X) with some hyporeflective areas under the retina corresponding with subretinal fluid. Under the hyperreflective RPE-choriocapillaris complex, there is a broad hyperreflective area (*) presenting a second fluid compartment in the choroid adjacent to the sclera
27.3 Commotio Retinae
Commotio retinae are defined as a transient gray-white opacification at the level of the deep sensory retina occurring after blunt ocular trauma, and it was first described by Berlin in 1873 (Berlin 1873). The whitening and elevation of the retina appear immediately after a trauma and can be limited to the central retina although it may even involve extensive areas of the peripheral retina progressing to retinal necrosis and chorioretinitis sclopetaria (Hesse et al. 1996).
Commotio retinae are grouped in two variations: a milder type (retinal concussion) with less dramatic gray-white changes and no visible pigment alterations, which later may progress to scarring. The more severe type (retinal contusion or chorioretinitis sclopetaria) can occur anterior at the point of impact (coup) or posterior at the remote impact site (contrecoup). A coup damage is therefore caused by local trauma directly at the site of impact, while a contusive contrecoup injury occurs at the opposite site of the blunt trauma creating damage at tissue interfaces. The consecutive whitening is more severe and a creamy discoloration of the RPE appears within 48 h after the injury. Fluorescein angiography typically demonstrates an alternated retinal vascular permeability, a bright leakage due to a partial breakdown of the outer blood-retina barrier at the level of the RPE. Visual acuity may be transiently or permanently reduced. The visual prognosis is good if the damaged location is outside the fovea. However, if the fovea is involved, vision loss may maintain permanently (Fig. 27.4) (Hesse et al. 1996; Sipperley et al. 1978).


Fig. 27.4
Chorioretinitis sclopetaria. (a) Fundus image of the left posterior pole resulting from a localized destruction of both the retina and choroid (yellow arrow) with connective tissue proliferation adjacent to the impact site (green arrow). (b, c) Fluorescein angiography shows irregular filling of the choriocapillaris and partially obstructed by connective tissue plaques. (d, e) SD-OCT showing marked thinning of the entire neuroretina
The clinicopathologic features of retinal contusion or chorioretinitis sclopetaria include direct traumatic chorioretinal damage followed by marked fibrovascular proliferation with variable replacement of vascular and neuroretinal tissue (Fig. 27.4a). A visible retinal opacity at the site of the contrecoup injury is considered to be a severe injury. It may later progress to a “salt-and-pepper” appearance accompanied by a hypofluorescence on fluorescein angiography. The chorioretinal circulation disturbance corresponds with a delayed filling and narrowing of the choroidal veins and changes in choroidal vasculature causing obstruction and occlusion of the choriocapillaris (Fig. 27.4b, c). The breakdown of the outer blood-retina barrier in the RPE may increase permeability of the choriocapillaris.
Histopathologic studies demonstrated a partial loss of the nerve fiber and ganglion cell layers and loss of the photoreceptors with hypertrophy and hyperplasia of the RPE. Although the sclera and a long posterior ciliary nerve remained intact, a marked ingrowth of fibrovascular tissue may be extended from the choroid into the subretinal space where it was covered by retinal pigment epithelium. On OCT there is a marked thinning of the neuroretina in the affected area compared to the unaffected adjacent areas (Fig. 27.4d, f) (Dubovy et al. 1997; Pahor 2000).
There have been conflicting reports on histopathologic features of Berlin’s edema or commotio retinae. Although Berlin originally hypothesized that the loss of the outer retinal transparency may relate to an extracellular edema, the origin and underlying pathogenesis remain controversial. Several postmortem studies on human and animal eyes confirmed a disruption of photoreceptor outer segments followed by phagocytosis of fragmented outer segments by the retinal pigment epithelium (RPE), while some authors reported a direct damage to the neurosensory retina as seen by swelling of Müller food processes and mitochondria in the nerve fiber layer. The gross anatomy revealed swollen inner segments with mitochondrial deposits of resembling calcium, indicating an increased permeability of the plasma membranes. The blunt ocular trauma may generate mechanical contrecoup distortion of the retina via vitreoretinal attachments. Mansour et al. hypothesized that hydraulic forces stretch the neuroretina at the level of the outer segments, while intact Müller cells hold the rest of the retina together (Mansour et al. 1992).
In vivo measurements by OCT revealed a traumatic lesion at the level of the photoreceptor-RPE complex, disclosing a detached sensory retina with fluid in the subretinal space. The reflectivity of the neuroretinal tissue was reduced and the architecture less compact. The neuroretina, held together by the Müller food processes, appears to be intact up to the photoreceptors. The uncommon hyperreflective inner retinal band possibly represents the photoreceptor inner segments, with less reflective outer segments underneath and in the subretinal space. Although the neuroretinal thickness was increased, the foveolar indentation remained still visible (Fig. 27.5b). Fundus biomicroscopy revealed a mild retinal elevation at the level of neurosensory retina and the RPE in the fovea with white concentric lines (Fig. 27.5b). The RPE phagocytoses damaged the outer segments and restore central vision within weeks. We determined a full anatomical and functional recovery of the macular lesion within 3 months. The patient’s vision returned to 20/20 OU 5 months later (Meyer et al. 2003a).


Fig. 27.5
(a) Fundus image of the left posterior pole showing dull foveal reflex and mild retinal elevation in the papillomacular region. (b) Horizontal OCT scan in the papillomacular area demonstrates a detachment of the entire neuroretina with hyporeflective space underneath, corresponding with subretinal fluid
Another complication that may occur after commotio retinae is the traumatic pigment epitheliopathy. It is possible to identify RPE atrophy with areas of discoloration and pigment clumping associated with patchy lesions, which represented post-traumatic pigment epitheliopathy. Fundus autofluorescence (FAF) examination demonstrated a well-defined reduced FAF patchy area with interposed granular increased autofluorescent areas in regions of the epitheliopathy (Fig. 27.6). OCT showed an area of increased reflectivity beneath the choriocapillaris/RPE complex and focal thinning of the retina. We also demonstrated the functional damage of traumatic pigment epitheliopathy using Humphrey’s computerized visual field in one patient and compared these findings to autofluorescence imaging, which correlated to the area of decreased sensitivity of the visual field (Fig. 27.7). Therefore, alterations in autofluorescent pattern in these patients may be an indirect indication of the function of the retina and RPE and could be suggestive of poor prognosis in some cases of commotio retinae.



Fig. 27.6
(a) Color fundus picture showing peripapillary retinal pigment epithelium (RPE) atrophy with areas of discoloration and pigment clumping (Courtesy of Dr Daniel Lavinsky). (b) Fundus autofluorescence (FAF) demonstrates reduced FAF area in the same region of RPE atrophy with an increased granular autofluorescent pattern inside the lesion (Courtesy of Dr Daniel Lavinsky). (c) Optical coherence tomography (OCT) shows increased reflectivity of the choriocapillaris/RPE complex and local thinning of the retina (white arrow) (Courtesy of Dr Daniel Lavinsky)

Fig. 27.7
(a) Ocular fundus photography of the right eye showing a mild pigmentary change at the temporal superior arcade (Courtesy of Dr Daniel Lavinsky). (b) Fundus autofluorescence (FAF) demonstrates two reduced autofluorescent areas with increased autofluorescent granular areas (arrow). Humphrey’s 24-2 SITA-standard strategy perimetry demonstrates acceptable reliability scores and a significant decrease in the sensitivity of lower nasal fields, corresponding to the superior temporal pigment epitheliopathy evident on autofluorescence (arrow) (Courtesy of Dr Daniel Lavinsky)
27.4 Choroidal Rupture
Choroidal rupture, first described by von Graefe in 1854, is a breach of Bruch’s membrane, the choroid, and the RPE secondary to a blunt ocular trauma (Von Graefe 1854). They can be divided into two main categories: a direct (anterior) type occurring at the site of the impact is oriented parallel to the ora serrata. Indirect ruptures, which are more common, occur predominantly at the posterior pole away from the site of impact. The rupture is here usually crescent shaped and concentrically aligned to the optic disk, with the convexity away from the optic disk. The majority (82 %) of ruptures run temporal to the optic disk may involve the macula.
Mechanical and vascular mechanisms are the two most frequently cited hypotheses explaining the damages in choroidal ruptures. During a closed-globe injury, the eyeball is first mechanically compressed and then rapidly hyperextended. The sclera’s tensile strength resists this compression. The retina is elastic and stretches during such an injury. However, Bruch’s membrane breaks because it does not have sufficient tensile strength or elasticity. The choriocapillaris is injured and bleeds into the sub-RPE and/or subretinal space. Such hemorrhage may hide the choroidal rupture initially. Over days, the blood clears and a whitish/yellowish, curvilinear, crescent-shaped subretinal streak is visible and concentric to the optic disk. Between 5 and 10 % of all blunt ocular trauma lead to indirect choroidal ruptures (Wagemann 1902).If there is a large amount of hemorrhage, surgery may be indicated with vitrectomy subretinal tissue plasminogen activator and gas displacement.
Over time, choroidal neovascularization (CNV) can develop. In most cases, the CNV involutes over time. Secretan et al. demonstrated that ruptures greater than 4000 μm in length and located within 1500 μm of the center of the fovea are more frequently associated with CNV (Secretan et al. 1998). In about 30 %, the CNV may recur, with a serous or hemorrhagic pigment epithelial detachment, anytime following the formation of the choroidal rupture. If the rupture or CNV does not involve the foveal center, vision may not be affected. Late complications may also include migration of RPE cells, ERM (epiretinal membrane) formation, loss of photoreceptors, optic atrophy, and retinal detachment.
Fluorescein angiography demonstrates a crescent-shaped delay filling at the site of the choroidal rupture. Early on there is a hypoperfusion of the choroidal artery. Evidence of the choroidal rupture hyperfluorescent streak occurred in the late phase of the FA at the site of the choroidal rupture (Fig. 27.8a–c). In another case it is possible to observe besides the choroidal rupture and progressive hyperfluorescent lesion in the macular area corresponding to a secondary CNV (Fig. 27.9a–d). ICG may also disclose a hypofluorescent rim along the choroidal veins as signs of an increased permeability of the choriocapillaris. These feeder vessels seem to have a greater caliber and a higher tendency to proliferate and are therefore more resistant to photodynamic therapy (PDT) and selective feeder vessel treatment as demonstrated by us previously (Mennel et al. 2005). More recently some case reports have been published treating this secondary CNV with anti-VEGF therapy such as bevacizumab or ranibizumab (Janknecht 2011; Piermarocchi and BenettiE 2011).



Fig. 27.8
Choroidal rupture. (a) Fundus image demonstrates a white crescent-like rupture of the choroid in the superior temporal quadrant. (b) Fluorescein angiography early phase showing an incomplete filling of the choroidal vasculature. In the area of the choroidal rupture, there is a dark hypofluorescence visible consistent with the rupture and loss of choroidal vessels. (c) Fluorescein angiography late phase showing sharp crescent-like hyperfluorescence due to leakage of choroidal vessels and a window defect at the choroidal rupture

Fig. 27.9
Choroidal rupture. (a) Fluorescein angiography showing crescent-like hyperfluorescent lesion in the superior temporal region corresponding to the choroidal rupture. (b–d) Serial fluorescein angiography shows progressive hyperfluorescent lesion in the macular area corresponding to a secondary choroidal neovascularization (CNV). (e) The retinal thickness map shows some areas of increased retinal thickness corresponding to the fluid of the active CNV. (f) RPE map shows the irregularity of the RPE where the CNV membrane is placed. (g) The horizontal scan shows a fibrovascular RPE detachment, with the choroidal rupture subretinal and intraretinal fluid and posterior vitreous detachment (G)
SD-OCT highlights the cross-sectional features of choroidal ruptures. The first case demonstrated a mounted subretinal lesion corresponding with fibrovascular scar tissue and some fluid underneath and inside the retina showing an active CNV secondary to a choroidal rupture (Fig. 27.9e–f).
In the second case, there was an irregular hyporeflective vertical failure corresponding to the choroidal rupture and a hyperreflective band radiating from the subretinal space through the entire neuroretina to the retinal surface, indicating a transretinal migration of fibrovascular cells (Fig. 27.10a, b).


Fig. 27.10
(a) The red-free image shows a concentric whitish lesion in the inferior temporal area corresponding to the choroidal rupture. (b) The enhanced depth OCT imaging shows an irregular hyporeflective vertical lesion at the deep choroid corresponding the choroidal rupture (arrow). The retina has a normal thickness; however, there is a hyperreflective band extending from the outer retinal to throughout the entire retina to the retinal surface (Courtesy of Dr Daniel Lavinsky)
27.5 Valsalva Retinopathy
The term “Valsalva retinopathy” was first used in 1972 by Duane to describe preretinal hemorrhages secondary to a sudden increase of intrathoracic pressure, in association after vomiting, coughing, heavy lifting, or straining stool, although in some instances no initiating event can be ruled out (Duane 1972). After the Valsalva maneuver, a sudden rise in the intraocular venous pressure causes retinal capillaries to spontaneously rupture; the consequence is the hemorrhage. A well-circumscribed, round, or dumbbell-shaped mound of preretinal blood is seen in or near the central macular area.
The most common site for this hemorrhage is the posterior pole, where the premacular bursa provides a preexisting anatomic space (Foos 1972). In addition the ILM (internal limiting membrane) has no firm attachments to the retina at the posterior pole, thus predisposing hemorrhagic detachments between both layers (Fig. 27.11a, b).


Fig. 27.11
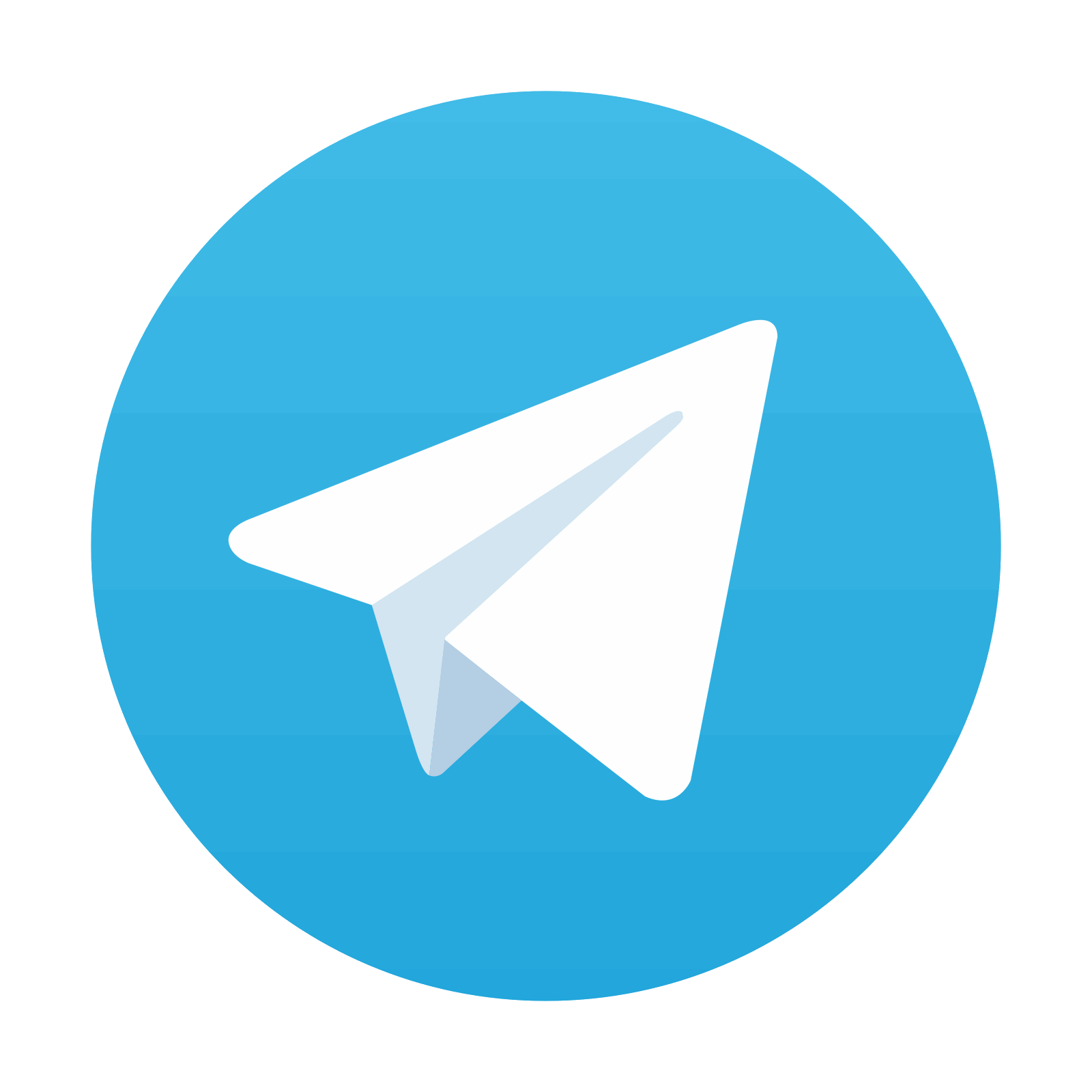
Valsalva retinopathy. (a) Fundus image showing well-circumscribed, round, or dumbbell-shaped mound of preretinal blood in or near the central macular area. (b) The entrapped blood under the ILM presents on SD-OCT as hyperreflective band in the retina surface obscuring the underlying retinal structures. (c) Fundus image taken in an upright position. The preretinal hemorrhage has a horizontal direction. (d) Fundus image taken after the head was tilted to the right side. The preretinal blood has shifted to the right side in an oblique direction
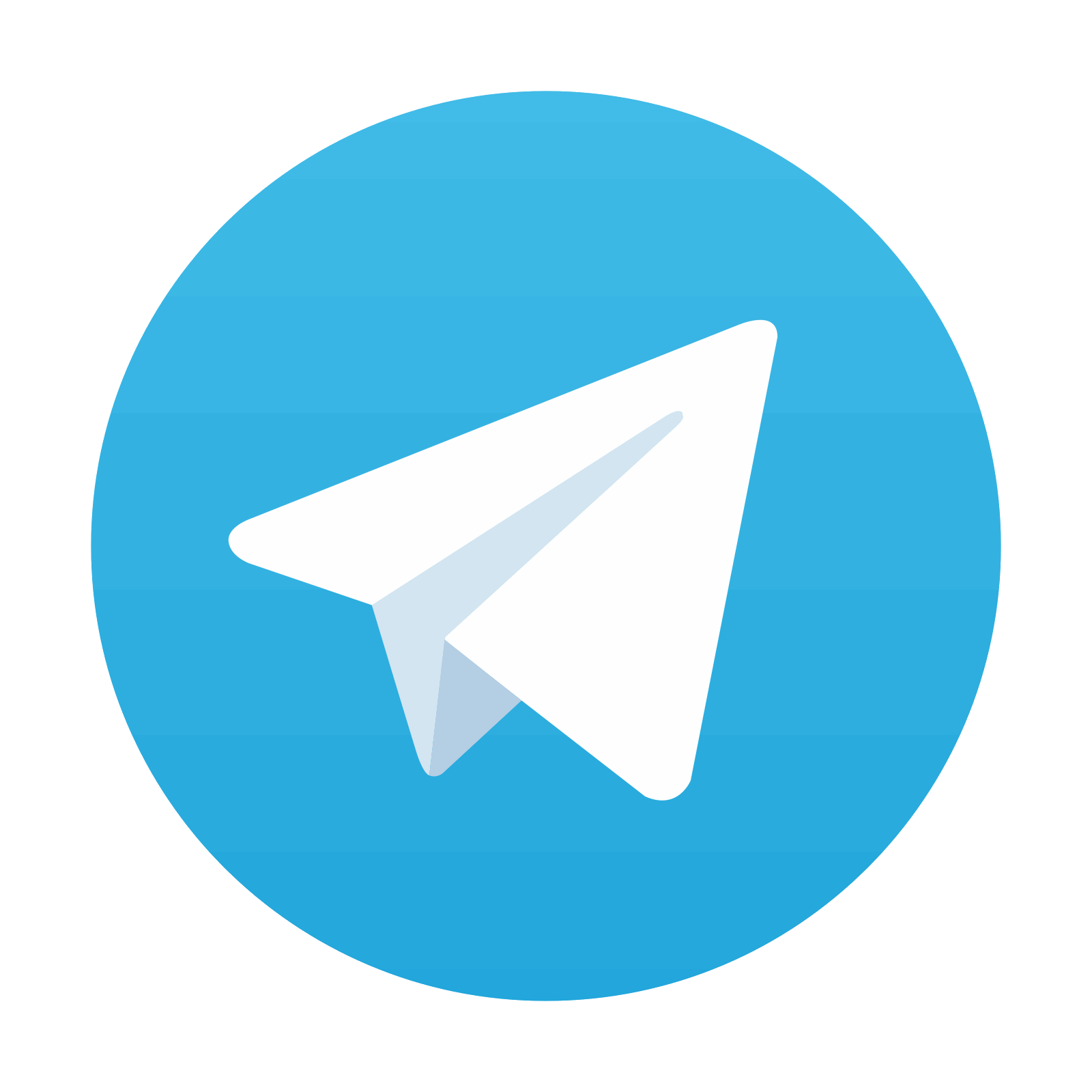
Stay updated, free articles. Join our Telegram channel

Full access? Get Clinical Tree
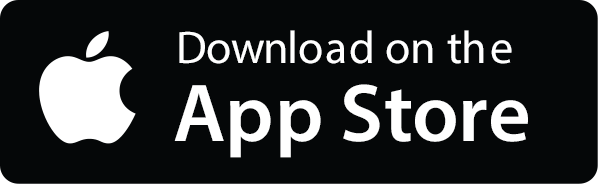
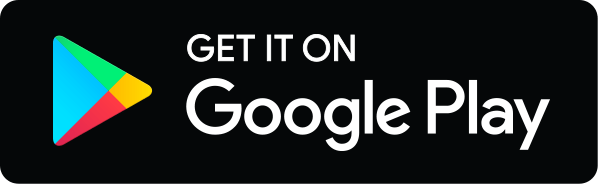
